Abstract
Ulvan is a sulfated polysaccharide found in the cell wall of the green algae Ulva. We first isolated several ulvan-utilizing Alteromonas sp. from the feces of small marine animals. The strain with the highest ulvan-degrading activity, KUL17, was analyzed further. We identified a 55-kDa ulvan-degrading protein secreted by this strain and cloned the gene encoding for it. The deduced amino acid sequence indicated that the enzyme belongs to polysaccharide lyase family 24 and thus the protein was named ulvan lyase. The predicted molecular mass of this enzyme is 110 kDa, which is different from that of the identified protein. By deletion analysis, the catalytic domain was proven to be located on the N-terminal half of the protein. KUL17 contains two ulvan lyases, one long and one short, but the secreted and cleaved long ulvan lyase was demonstrated to be the major enzyme for ulvan degradation.
(A) Domain structure of UllAKUL17 and plasmids containing UllAKUL17. (B) CBB R-250 staining of 10% SDS-PAGE gel. (C) Activity staining with ulvan-containing agarose gel.
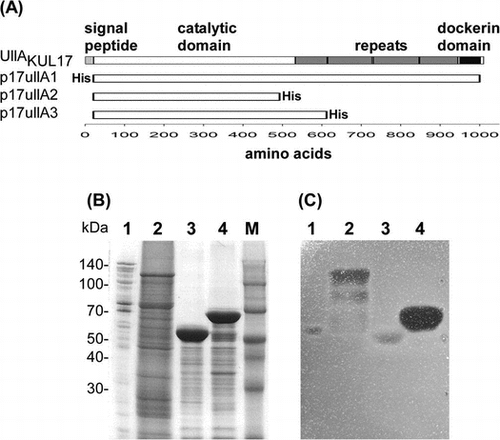
Energy shortages and environmental pollution affect the sustainable development of the world. In addressing this problem, marine macroalgal species have gained considerable global attention as a source of renewable biomass.Citation1) Among the three main divisions of macroalgae – red, brown, and green algae – green algae remain largely unexploited. In the past decade, marine eutrophication has promoted the proliferation of the algae Ulva sp., causing phenomena commonly known as algal blooms or green tide.Citation2) As a consequence of their strong environmental impact, the interest in exploiting these green seaweeds has increased significantly, especially in its long chain polymer, ulvan.Citation3)
The main constituents of ulvan are 3-sulfated rhamnose (Rha3S), D-glucuronic acid (GlcA), L-iduronic acid (IdoA), and D-xylose (Xyl).Citation4) Each repeating unit of ulvan is a disaccharide composed of Rha3S linked with GlcA or IdoA, termed ulvanobiuronic acid A or B, respectively, or xylose in lower amounts (Supplementary Fig. S1). Ulvan has many potential applications in food, pharmaceutical, agricultural, and chemical industries because of its physicochemical and biological features.Citation4)
The conversion of polymers into fermentable oligomers requires chemical or biological break down. Chemical conversion could be very expensive and thus may not be an economical choice for large-scale production.Citation5) Enzymatic hydrolysis has been suggested as a more economical alternative because it requires low energy and results in the formation of fewer byproducts.Citation6) Thus, the discovery of new enzymes for ulvan degradation becomes a key step in improving the management and use of this resource.
Few ulvan-degrading enzymes have been isolated from nature. Ulvan lyase activity was first detected in a marine bacterium in 1997.Citation7) Later, the marine bacterium Persicivirga ulvanivorans sp. nov. (re-classified as Nonlabens ulvanivorans) PLR was found to degrade ulvan.Citation8) Two ulvan lyases were purified from the culture supernatant of this speciesCitation9) and were later found to be 74% identical.Citation10) These were the first ulvan-degrading enzymes characterized biochemically. The N. ulvanivorans ulvan lyase cleaves the glycosidic bond between R3S and GlcA or IdoA via a β-elimination mechanism, resulting in the formation of an unsaturated uronic acid (Δ, 4-deoxy-L-threohex-4-enopyranosiduronic acid) at the non-reducing end (Supplementary Fig. S1E).
Recently, the genes encoding for the two types of ulvan lyase, long and short, were identified in three strains of Alteromonadales ulvan-degrading bacteria.Citation11) The strains were found to contain very similar short ulvan lyases. The long-type ulvan lyase was shown to be composed of two modules: the catalytic module, which is similar to the short-type ulvan lyase, and the C-terminal module. Both types of ulvan lyase exhibit enzyme activity. The ulvan lyases isolated from N. ulvanivorans and from the three Alteromonadales bacteria show no sequence similarity. The objective of this study is to discover more ulvan-degrading bacteria that can produce novel ulvan-degrading enzymes, thereby contributing to the potential use of ulvan as a source of oligosaccharides.
Materials and methods
Extraction of ulvan
Green alga Ulva ohnoiCitation12) was collected at Uranouchi Bay, Tosa, Kochi, Japan (33°26′13″ N, 133°26′35″ W) in August 2013. The washed and air-dried seaweeds were ground into fine pieces using a laboratory blender. Freshly ground seaweeds were soaked in 75% ethanol for 1 day and recovered by filtration with a 60 μm nylon mesh. Ulvan was extracted with hot water method.Citation13)
Screening and isolation of ulvan-utilizing bacteria
Mollusks and shrimps were fed with U. ohnoi suspended in artificial seawater in the laboratory. Some of the small marine animals ate the seaweed, and their feces were used as sources of ulvan-utilizing bacteria. The feces were inoculated into half strength marine broth (referred as 1/2 MB: 18.7 g Difco Marine broth 2216 and 9.8 g NaCl in 1000 mL DW) containing 0.2% ulvan and then incubated at 25 °C overnight. The cell suspension was spread on ulvan agar media (0.2% ulvan in three-quarter strength artificial seawater with 1.5% agar) and incubated at 25 °C for several days. Colonies were replicated onto 1/2 MB agar as master plates and ulvan agar plates for screening. Once cell growth was observed, the ulvan agar plates were flooded with 5% cetylpyridinium chloride (CPC), which reacts with acidic polysaccharides, such as ulvan, to form insoluble precipitates. Thus, the formation of clear haloes indicates the presence of bacterial cells that degrade ulvan to oligosaccharides. Additionally, a bigger halo corresponds to a higher degradation activity.Citation14)
Identification of ulvan-utilizing bacteria
The 16S rRNA partial gene fragments of the ulvan-utilizing strains were amplified directly from colonies via PCR using the universal primers pr0R2 (5′-AGAGTTTGATCNTGGCTCAG-3′) and 907R (5′-CCGTCAATTCCTTTRAGTTT-3′). The amplified fragments were sequenced using a BigDye terminator v3.1 cycle sequencing kit (Applied Biosystems) and an ABI PRISM 3100-Avant Genetic Analyzer (Applied Biosystems).
Extraction of secreted ulvan-degrading enzymes
Ulvan-utilizing bacterial cells grown in 40 mL of 1/2 MB were transferred to 4 L of fresh 1/2 MB containing 0.05% ulvan and were then cultivated at 25 °C for 3 days in a mini jar fermentor (TSO-M, Takasaki Kagaku). Bacterial culture supernatant was prepared by centrifuging the cultures at 12,000 × g for 30 min. Ammonium sulfate was added to the supernatant to 70% saturation in order to extract secreted proteins. The precipitate was suspended in a small volume of 20 mM Tris-HCl (pH 8.0) and dialyzed against the same buffer. For a small volume of culture supernatant, an ultrafiltration concentrator (Vivaspin 500, Sartorius) was used.
Purification of crude ulvan-degrading enzymes
Proteins precipitated with ammonium sulfate were loaded onto 10 mL of Q Sepharose Fast Flow (GE Healthcare) equilibrated with 20 mM Tris-HCl buffer (pH 8.0). Bound proteins were eluted with a 100-mL linear gradient of NaCl (0–0.5 M) in the same buffer. Proteins in each fraction were analyzed using SDS-PAGE, and active fractions containing ulvan-degrading enzymes were identified using the ulvan-gel overlay assay indicated in the next section.
Active fractions were pooled and ammonium sulfate was added to a final concentration of 2 M. This solution was loaded onto 10 mL of Phenyl Sepharose 6 Fast Flow (GE Healthcare) equilibrated with 20 mM Tris-HCl buffer (pH 8.0) containing 2 M ammonium sulfate. Bound proteins were eluted with a 100-mL linear gradient of ammonium sulfate (2–0 M) in 20 mM Tris-HCl (pH 8.0). Active fractions were assayed as above and concentrated using Vivaspin 500.
Activity staining overlaid on ulvan-containing agarose gel
We adopted the protocol for assaying alginate lyase activityCitation15) with the following modifications. Proteins were separated via SDS-PAGE, and enzymes were renatured by incubating the gel in 100-mL of 20 mM Tris-HCl (pH 8.0) for 10 min. Incubation was done three times. The polyacrylamide gel was overlaid onto an agarose gel (0.2% ulvan in three-quarter strength artificial seawater with 1.0% agar) and incubated overnight at 30 °C. The agarose gel was immersed in 5% CPC, and proteins with ulvan-degrading activity were visualized as clear bands.
Assay for ulvan-degrading enzyme
The formation of reducing ends was measured by the 3,5-dinitrosalicylic acid (DNS) method.Citation16) Enzyme reaction was carried out in 500 μL of solution (0.2% (w/v) ulvan, 20 mM Tris-HCl (pH 8.0), 500 mM NaCl, and purified enzyme) for 1 h at 25 °C. After adding 500 μL of DNS reagent (1% 3,5-dinitrosalicylic acid, 30% potassium sodium tartrate, 0.4 M NaOH), the mixture was boiled for 10 min and then cooled. The amount of reducing ends was monitored by measuring the absorbance at 540 nm. L-Rhamnose was used to make a standard curve. For the ulvan lyase assay, 0.1% (w/v) ulvan solution was incubated with 0.2 μg purified enzyme in 20 mM Tris-HCl buffer (pH 8.0) with 500 mM NaCl. The formation of double bonds was monitored by the increase in absorbance at 235 nm.Citation11)
Identification of ulvan lyase genes
Proteins that exhibited ulvan-degrading activities were analyzed using SDS-PAGE and transferred to a PVDF membrane using a blotting buffer (10 mM CAPS (pH 11.0) and 10% methanol) at 4 °C. Proteins were stained with CBB R-250 and excised from the membrane. The N-terminal amino acid sequences of the excised proteins were determined using the protein sequencer PPSQ-31A (Shimadzu). In order to identify genes encoding the proteins, the determined amino acid sequences were searched against the protein database deduced from the draft genome sequence of the ulvan-utilizing strain using the program BLASTP.
Cloning of ulvan lyase gene
The full-length long-type ulvan lyase gene (UllA) of the KUL17 strain was amplified from chromosomal DNA via PCR using the primers 17ullAA1 and 17ullAB1 and PrimeSTAR HS DNA polymerase (Takara Bio). C-terminal truncated versions of UllAKUL17 were amplified using the primer pairs 17ullAA2 and 17ullAB2, and 17ullAA2 and 17ullAB3. All the PCR products were cloned into pET21a vector using In-Fusion HD Cloning Kit (Takara Bio). The primer sequences and constructed plasmids are listed in Table . The nucleotide sequence of UllAKUL17 has been deposited in DDBJ under the accession number LC278382.
Table 1. Primers and plasmids used in this study.
Purification of ulvan lyase
Escherichia coli BL21(DE3) cells were transformed with the constructed plasmids and the resulting transformants were used to overexpress ulvan lyases by adding 1 mM IPTG as previously reported by Yoshimochi et al.Citation17) After IPTG addition, the cells were incubated at 20 °C for 4 h and then harvested and disrupted by sonication. After removing cell debris, the supernatant was purified using a Ni2+ affinity column (Ni-NTA Agarose, Qiagen).
HPLC analysis
Oligosaccharides produced by the degradation of ulvan were analyzed by size-exclusion chromatography using a Superdex Peptide 10/300 GL (GE Healthcare) column on LaChrom Elite (Hitachi High Technologies). Analysis was performed at a flow rate of 0.5 mL min−1 using a 20 mM Tris-HCl (pH 8.0) eluent with 200 mM NaCl. A refractive index detector (Hitachi High Technologies) was also used.
Results
Isolation of ulvan-utilizing bacteria
In this study, we screened the feces of small Ulva-eating animals for ulvan-utilizing bacteria. The plate assay that we used to test for ulvan-degrading activity was analogous to the assay for testing alginate lyase activity.Citation15) The isolated bacteria showed different levels of ulvan-degrading activity as indicated by the different sizes of the haloes. Three strains, KUL10, KUL17, and KUL42, showed high enzyme activity. Among these, KUL17, which was isolated from a mollusk, had the fastest growth and the highest enzyme activity; consequently, this strain was analyzed further. KUL17 utilized ulvan as a sole carbon source; thus, ulvan was very likely converted into the monomeric carbohydrate L-rhamnose. KUL17 indeed grew well on agar plates with 1% L-rhamnose as a sole carbon source (data not shown). The partial nucleotide sequence of its 16S rRNA gene indicates that KUL17 belongs to the genus Alteromonas.
Identification of ulvan-degrading enzymes
Firstly, we tested if ulvan-degrading enzymes were inducible. KUL17 cells were cultivated in 1/2 MB with varying concentrations of ulvan. Almost no ulvan-degrading enzymes were detected in fractions from cells grown in media without ulvan. In contrast, at least three bands of proteins with enzyme activity were visible when fractions from cells grown in media with ulvan concentrations as low as 0.01% were analyzed (Supplementary Fig. S2). We used 0.05% ulvan for enzyme induction throughout this study.
Secreted proteins from KUL17 grown in 1/2 MB with 0.05% ulvan were precipitated with ammonium sulfate. They were then subjected to strong anion exchange chromatography and hydrophobic interaction chromatography in order to partially purify ulvan-degrading enzymes. Although multiple active proteins were detected in the secreted fractions, we focused on the most active ca. 55-kDa protein. After the second chromatography, SDS-PAGE analysis of the fractions and the assay for ulvan-degrading activity on ulvan-containing gels were conducted simultaneously (Fig. ). The candidate for ulvan-degrading activity is indicated by an arrowhead (Fig. (A)).
Fig. 1. SDS-PAGE analysis of proteins separated by phenyl Sepharose chromatography.
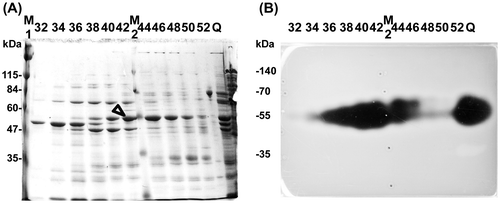
Fractions 37–46 of KUL17 proteins were pooled and re-analyzed using SDS-PAGE (Fig. ). Among the three proteins with molecular mass around 50 kDa, protein “a” was most likely to have ulvan-degrading activity; therefore, we determined its N-terminal sequence. Analysis of the draft genome of KUL17 revealed that this sequence, “EVTLEQQIKITDEGL,” matched with the internal sequence of a fibronectin type III domain protein. This protein is 90% identical to a long ulvan lyase in Alteromonas sp. PLSVCitation11); thus, we identified protein “a” as the gene product of UllA (ulvan lyase A).
Fig. 2. Identification of ulvan-degrading enzyme.
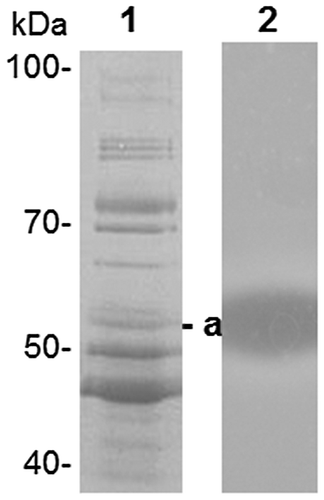
Cloning of the UllA gene and expression in E. coli
The domain structure of KUL17 UllA (UllAKUL17) is shown in Fig. (A). UllAKUL17 is composed of 999 amino acids with a predicted 21-amino-acid signal peptide. Indeed, the determined N-terminal sequence of the secreted ulvan-degrading protein matches the sequence without the signal peptide of UllAKUL17. The catalytic domain is located in the N-terminal half and multiple repeats and a dockerin domain is located in the C-terminal half.
Fig. 3. Domain structure and purification of UllA.
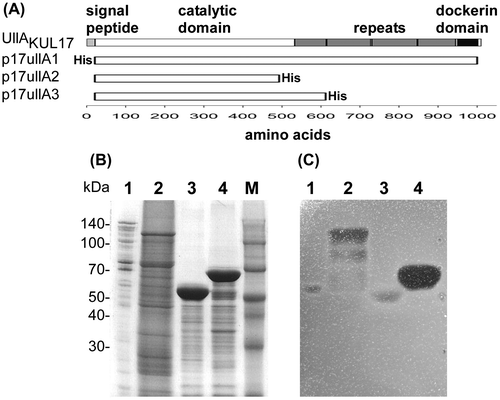
We cloned the full-length UllA without the signal peptide and with a His-tag at the N-terminus, and then inserted this sequence into the expression vector pET21a to construct p17UllA1 (Fig. (A)). When the full-length UllA was expressed as an active enzyme (Fig. (C), lane 2), many bands of degraded protein, aside from the band corresponding to the intact 110-kDa protein, were observed (Fig. (B), lane 2). Since the His-tag was fused to the N-terminus, degradation should have occurred at the C-terminus. Degraded UllA fragments of various lengths showed activity until they reached the size of UllA secreted from the original strain (Fig. (C), lane 1 vs. 2). The size of the secreted UllA suggests that almost all the repeats and the dockerin domain should be deleted. We then designed several C-terminal truncated versions of UllA (Fig. (A)). When deletion was initiated at a site slightly beyond the putative catalytic domain (p17UllA2), enzyme activity dramatically decreased (Fig. (C), lane 3). On the other hand, other truncated version of UllA showed high enzyme activity (Fig. (C), lane 4). These results indicate that the C-terminal half is dispensable, at least for ulvan-degrading activity, which is consistent with the lack of the C-terminal half in the secreted natural UllA.
Characterization of enzymes
The purified full-length UllA1KUL17 was mixed with an ulvan solution. When ulvan is degraded by an elimination reaction, a double bond is generated between C4 and C5 of the sugar ring at the newly formed non-reducing end. The double bond formation can be monitored as a change in absorbance at 235 nm.Citation18) As expected, the absorbance at 235 nm increased (Fig. ), clearly demonstrating that UllA is a polysaccharide lyase, not a glycoside hydrolase. Since the full-length UllA was unstable, we used the truncated version for further analysis and calculated the kinetic parameters by measuring the amount of reducing ends formed by the reaction between ulvan and UllA3KUL17. The values of Vmax and Km for UllA3KUL17 are 185 μmol min−1·mg protein−1 and 0.72% (w/v), respectively.
Identification of degradation products of ulvan
Ulvan was allowed to react with purified UllA3KUL17 for varying lengths of time, and the products were analyzed using size-exclusion chromatography. With shorter incubation times, relatively large depolymerization products were generated. The amount of products with lower degrees of polymerization (DP), such as DP6, DP4, and DP2, increased when incubation times became longer. Most of the products were DP6 and DP4 at 24 h in this reaction condition (Fig. ). This indicated that UllA3 is an endo-ulvan lyase. When the amount of enzyme used was increased, almost all products became DP4 (Fig. ).
Fig. 5. Analysis of reaction products of ulvan by UllA3.
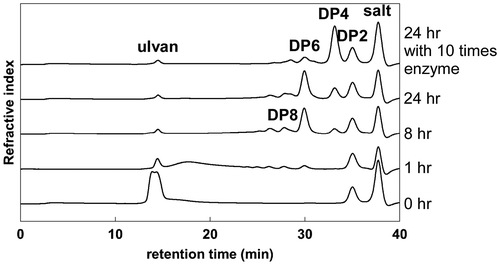
Discussion
So far, three strains belonging to the order Alteromonadales – Alteromonas sp. LTR, Alteromonas sp. LOR, and Pseudoalteromonas sp. PLSV – have been reported to contain two types of ulvan lyases.Citation11) All of the ulvan lyases belong to the family PL24. The N-terminal half of the long ulvan lyase is homologous to the full-length of the short ulvan lyase, with about 70% similarity. We have isolated three ulvan-utilizing strains belonging to Alteromonadales: KUL10 (Glaciecola sp.),Citation19) and two Alteromonas strains, KUL17 and KUL42. The three KUL strains contain genes for two types of ulvan lyase (unpublished data). When the long-type ulvan lyases are compared phylogenetically, enzymes from KUL17 and KUL42 form a clade distinct from those of LOR and PLSV (Fig. ), although the similarity among the four enzymes is greater than 90%. The enzyme from KUL10, however, is less similar.
Fig. 6. Evolutionary relationship of long-type ulvan lyase. The evolutionary history was inferred using the Neighbor-Joining method.Citation30)
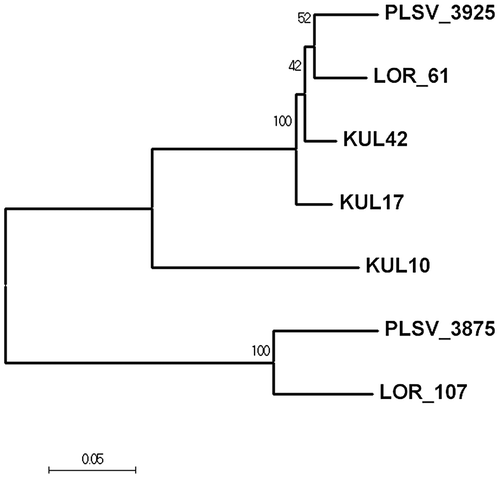
While the importation of a polysaccharide such as alginate across the cytoplasmic membrane by the ABC transporter is possible,Citation20) ulvan has never been known to be incorporated into cells. Ulvan lyase must be secreted in order to break ulvan down to oligosaccharides small enough to be imported through transporters. The long-type ulvan lyase was proven to be secreted from the marine Alteromonas strain in its C-terminal truncated form. We could not detect the short-type ulvan lyase in the secreted proteins from KUL17. These data demonstrate that the long-type ulvan lyase is the major enzyme to depolymerize ulvan, at least outside of cells.
The long-type ulvan lyase is composed of two modules. The N-terminal half contains the catalytic module, which is similar to the short-type ulvan lyase.Citation11) We also demonstrated that the C-terminal truncated long ulvan lyase showed high enzyme activity. Although the full-length long-type ulvan lyases were also active, they were very much unstable, indicating that the C-terminal half is a target for protein degradation. The sizes of the secreted ulvan lyases are ca. 55 kDa, which exactly matches that of the N-terminal catalytic domain. It is unclear whether the C-terminus is removed before or after secretion. The long-type ulvan lyase from N. ulvanivorans PLR, which is smaller by 534 amino acids and has no similarity with Alteromonadales long ulvan lyases, is composed of two domains.Citation21) The N-terminal region contains the catalytic domain, and the C-terminus specifically binds to ulvan. The C-terminus of the long-type ulvan lyase from KUL17 contains 3–4 repeats with ca. 100 amino acids each and a dockerin domain. The function of the repeats is unknown, but it is possible that ulvan binds to them. If this is the case, then the long-type ulvan lyase must be secreted in its full-length form and the C-terminus must be cleaved after secretion.
We detected at least three secreted proteins with ulvan-degrading activity from KUL17, one of which was the long-type ulvan lyase. Since the activity staining on ulvan-containing agarose gel is based on the precipitation of sulfated polysaccharides with CPC,Citation15) cleavage of either the glycosidic linkages of the carbohydrate backbone or the sulfate ester groups can produce clear bands. We detected a sulfatase from the preliminary results of the N-terminal amino acid sequence analyses of secreted proteins, which could be another protein with ulvan-degrading activity. K-Carrageenan is a sulfated polysaccharide from red algae.Citation22) A carrageenan-sulfatase isolated from Pseudoalteromonas atlantica behaves as an endo-acting enzyme and catalyzes the desulfation of polymers instead of oligosaccharides.Citation23) Secreted sulfatases could remove the sulfate group of the polymer ulvan.
Recently, a new ulvan lyase from Pseudoalteromonas sp. PLSV (PLSV_3936) belonging to PL25 was identified.Citation24) This new ulvan lyase could be secreted, although no direct evidence has been demonstrated so far. We have found the homologous gene in KUL17 (unpublished data). Because the short-type ulvan lyase does not seem to be secreted, this third ulvan lyase could be one of the secreted proteins with ulvan-degrading activity.
KUL17 grew on media with either ulvan or rhamnose as a sole carbon source, indicating that the strain completely degrades ulvan to rhamnose. There are many steps in the ulvan degradation pathway, the first of which is catalyzed by ulvan lyase. Genes coding for proteins involved in the polysaccharide degradation pathway co-localize in a cluster referred to as the polysaccharide utilization loci (PUL).Citation25) We found many genes related to ulvan degradation surrounding UllA (unpublished data). Foran et al. have clearly demonstrated that genes in the PUL of Alteromonas sp. LOR encode ulvan-degradation enzymes, such as β-glucuronyl hydrolase and rhamnosidase.Citation26) Many proteins are involved in the complete degradation of ulvan and there is still a need to elucidate the entire degradation pathway.
Author contribution
Chuan He (CH) and Kouhei Ohnishi (KO) designed the experiments. CH performed the experiments. Hisashi Muramatsu, Shin-ichiro Kato, CH, and KO analyzed the data. CH and KO wrote the paper.
Disclosure statement
No potential conflict of interest was reported by the authors.
Funding
This work was supported by The Kochi University President’s Discretionary Grant.
Supplemental material
Supplemental material for this article can be accessed at https://doi.org/10.1080/09168451.2017.1379352.
Acknowledgements
We thank Masanori Hiraoka for providing useful information about green algae Ulva sp.
References
- Nigam PS, Singh A. Production of liquid biofuels from renewable resources. Prog Energy Combust Sci. 2011;37:52–68.10.1016/j.pecs.2010.01.003
- Smetacek V, Zingone A. Green and golden seaweed tides on the rise. Nature. 2013;504:84–88.10.1038/nature12860
- Bobin-Dubigeon C, Lahaye M, Guillon F, et al. Factors limiting the biodegradation of Ulva sp. cell-wall polysaccharides. J Sci Food Agr. 1997;5:341–351.
- Lahaye M, Robic A. Structure and functional properties of ulvan, a polysaccharide from green seaweeds. Biomacromolecules. 2007;8:1765–1774.10.1021/bm061185q
- Huber GW, Iborra S, Corma A. Synthesis of transportation fuels from biomass: chemistry, catalysts, and engineering. Chem Rev. 2006;106:4044–4098.10.1021/cr068360d
- Yun YM, Kim DH, Oh YK, et al. Application of a novel enzymatic pretreatment using crude hydrolytic extracellular enzyme solution to microalgal biomass for dark fermentative hydrogen production. Bioresour Technol. 2014;159:365–372.10.1016/j.biortech.2014.02.129
- Lahaye M, Brunel M, Bonnin E. Fine chemical structure analysis of oligosaccharides produced by an ulvan-lyase degradation of the water-soluble cell-wall polysaccharides from Ulva sp, (Ulvales, Chlorophyta). Carbohydr Res. 1997;304:325–333.10.1016/S0008-6215(97)00270-X
- Barbeyron T, Lerat Y, Sassi JF, et al. Persicivirga ulvanivorans sp. nov., a marine member of the family Flavobacteriaceae that degrades ulvan from green algae. Int J Syst Evol Microbiol. 2011;61:1899–1905.10.1099/ijs.0.024489-0
- Nyvall Collén P, Sassi JF, Rogniaux H, et al. Ulvan lyases isolated from the flavobacteria Persicivirga ulvanivorans are the first members of a new polysaccharide lyase family. J Biol Chem. 2011;286:42063–42071.10.1074/jbc.M111.271825
- Kopel M, Helbert W, Henrissat B, et al. Draft genome sequence of Nonlabens ulvanivorans, an Ulvan-degrading bacterium. Genome Announc. 2014;2:e00793–14.
- Kopel M, Helbert W, Belnik Y, et al. New family of ulvan lyases identified in three isolates from the alteromonadales order. J Biol Chem. 2016;291:5871–5878.10.1074/jbc.M115.673947
- Hiraoka M, Shimada S, Uenosono M, et al. A new green-tide-forming alga Ulva ohnoi Hiraoka et Shimada sp. nov. (Ulvales, Ulvophyceae) from Japan. Phycol Res. 2004;52:17–29.
- Robic A, Sassi JF, Lahaye M. Impact of stabilization treatments of the green seaweed Ulva rotundata (Chlorophyta) on the extraction yield, the physico-chemical and rheological properties of ulvan. Carbohdr Polym. 2008;74:344–352.10.1016/j.carbpol.2008.02.020
- Ruijssenaars HJ, Hartmans S. Plate screening methods for the detection of polysaccharase-producing microorganisms. Appl Microbiol Biotechnol. 2001;55:143–149.10.1007/s002530000477
- Sawabe T, Takahashi H, Ezura Y, et al. Cloning, sequence analysis and expression of Pseudoalteromonas elyakovii IAM 14594 gene (alyPEEC) encoding the extracellular alginate lyase. Carbohydr Res. 2001;335:11–21.10.1016/S0008-6215(01)00198-7
- Miller GL. Use of dinitrosalicylic acid reagent for determination of reducing sugar. Anal Chem. 1959;31:426–428.10.1021/ac60147a030
- Yoshimochi T, Hikichi Y, Kiba A, et al. The global virulence regulator phca negatively controls the Ralstonia solanacearum hrp regulatory cascade by repressing expression of the prhIR signaling proteins. J Bacteriol. 2009;191:3424–3428.10.1128/JB.01113-08
- Preiss J, Ashwell G. Alginic acid metabolism in bacteria. I. Enzymatic formation of unsaturated oligosaccharides and 4-deoxy-l-erythro-5-hexoseulose uronic acid. J Biol Chem. 1962;237:309–316.
- He C, Ohnishi K. Efficient renaturation of inclusion body proteins denatured by SDS. Biochem Biophys Res Commun. 2017;490:1250–1253.10.1016/j.bbrc.2017.07.003
- Maruyama Y, Itoh T, Kaneko A, et al. Structure of a bacterial ABC transporter involved in the import of an acidic polysaccharide alginate. Structure. 2015;23:1643–1654.10.1016/j.str.2015.06.021
- Melcher RL, Neumann M, Fuenzalida Werner JP, et al. Revised domain structure of ulvan lyase and characterization of the first ulvan binding domain. Sci Rep. 2017;7:44115.10.1038/srep44115
- Whyte JNC, Hosford SPC, Englar JR. Assignment of agar or carrageenan structures to red algal polysaccharides. Carbohydr Res. 1985;40:336–341.10.1016/0008-6215(85)85135-1
- Préchoux A, Genicot S, Rogniaux H, et al. Enzyme-assisted preparation of furcellaran-like κ-/β-carrageenan. Mar Biotechnol. 2016;18:133–143.10.1007/s10126-015-9675-3
- Ulaganathan T, Boniecki MT, Foran E, et al. New ulvan-degrading polysaccharide lyase family: structure and catalytic mechanism suggests convergent evolution of active site architecture. ACS Chem Biol. 2017;12:1269–1280.10.1021/acschembio.7b00126
- Helbert W. Marine polysaccharide sulfatases. Front Mar Sci. 2017;4:6. doi:10.3389/fmars.2017.00006
- Foran E, Buravenkova V, Kopel M, et al. Functional characterization of a novel “ulvan utilization loci” found in Alteromonas sp. LOR genome. Algal Res. 2017;25:39–46.10.1016/j.algal.2017.04.036
- Marchler-Bauer A, Bryant SH. CD-Search: protein domain annotations on the fly. Nucleic Acids Res. 2004;32:W327–331.10.1093/nar/gkh454
- Petersen TN, Brunak S, Von Heijne G. SignalP 4.0: discriminating signal peptides from transmembrane regions. Nat Methods. 2011;8:785–786.10.1038/nmeth.1701
- Heger A, Holm L. Rapid automatic detection and alignment of repeats in protein sequences. Proteins. 2000;41:224–237.10.1002/(ISSN)1097-0134
- Saitou N, Nei M. The neighbor-joining method: A new method for reconstructing phylogenetic trees. Mol Biol Evol. 1987;4:406–425.
- Kumar S, Stecher G, Tamura K. MEGA7: molecular evolutionary genetics analysis version 7.0 for bigger datasets. Mol Biol Evol. 2016;33:1870–1874.10.1093/molbev/msw054