Abstract
We investigated suppression of the slow growth of an Escherichia coli ispA null mutant lacking farnesyl diphosphate (FPP) synthase (i.e. IspA) by plasmids carrying prenyl diphosphate synthase genes. The growth rates of ispA mutant-transformants harboring a medium-copy number plasmid that carries ispA or ispB were almost the same as that of the wild-type strain. Although the level of FPP in the transformant with the ispA plasmid was almost the same as that in the wild-type strain, the level in the transformant with the ispB plasmid was as low as that in the ispA mutant. Purified octaprenyl diphosphate synthase (IspB) could condense isopentenyl diphosphate (IPP) with dimethylallyl diphosphate (DMAPP) to form octaprenyl diphosphate and nonaprenyl diphosphate. It is possible that suppression of the slow growth of the ispA mutant by ispB was due to condensation of IPP not only with FPP but also with DMAPP by octaprenyl diphosphate synthase.
Graphical abstract
Overexpression of ispB encoding octaprenyl diphosphate synthase suppressed the phenotype of E. coli null mutant ispA encoding farnesyl diphosphate synthase.
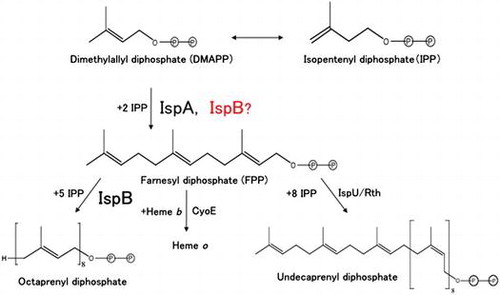
Farnesyl diphosphate (FPP) is a key intermediate in isoprenoid biosynthesis and a direct precursor of octaprenyl diphosphate, undecaprenyl diphosphate, and heme o in Escherichia coli (Figure ) [Citation1,2]. There are three genes encoding prenyl diphosphate synthases in the chromosome of E. coli: ispA, encoding FPP synthase [Citation2,3]; ispB, encoding octaprenyl diphosphate synthase [Citation4]; and ispU/rth, encoding undecaprenyl diphosphate synthase [Citation5,6]. FPP synthase catalyzes the sequential condensation of isopentenyl diphosphate (IPP) with dimethylallyl diphosphate (DMAPP) and geranyl diphosphate to form all-E-FPP, which is the substrate for both octaprenyl diphosphate synthase and undecaprenyl diphosphate synthase. Both ispB [Citation7] and ispU/rth [Citation6] genes are essential for growth. The lack of these genes is considered to result in the absence of indispensable compounds such as the isoprenoid quinones, derived from octaprenyl diphosphate, and undecaprenyl phosphate, derived from undecaprenyl diphosphate, respectively.
We previously constructed an E. coli ispA null mutant, which contained significantly lower levels of isoprenoid quinones and a modestly lower level of undecaprenyl phosphate than the parental strain and grew slower [Citation8]. Recently, Krute et al. constructed a null ispA mutant of Staphylococcus aureus, and they showed that the mutant synthesized a low level of FPP [Citation9]. It would be interesting to clarify the origin of FPP in cells lacking the canonical FPP synthase. To determine which gene product substitutes for FPP synthase in such a mutant of E. coli, suppression of the slow growth of the mutant by plasmids carrying the genes for other prenyl diphosphate synthases was investigated.
Materials and methods
Bacterial strains and plasmids
The E. coli K-12 strains used are listed in Table . SF7 is an ispA null mutant, and SF5 is an isogenic control strain [Citation8]. SF7N is an ispA null derivative of W3110 constructed in this study. pUC118 was used as a high-copy number vector (200 copies per cell) [Citation10]. pCL1920 and pCL1921 were used as medium-copy number vectors (five copies per cell) [Citation10]. Expression vector pTrcHis30 was constructed as described previously [Citation11]. Plasmids pR10 [Citation3], pKA5 [Citation4], and pJK286-rth+ cdsA+ [Citation6] were sources of ispA, ispB, and ispU/rth genes, respectively (Figure ). Plasmid pHX1 [Citation3] was a high-copy number plasmid that carried the ispA gene. pHT1 was constructed by inserting the 1.5-kb EcoRI-SalI fragment of pR10 into the same sites of pCL1921. To construct pHT2 encoding hexahistidine-tagged octaprenyl diphosphate synthase, the ispB gene in pKA5 was amplified by PCR using a forward primer containing the BamHI site, 5′-GAAAGGATCCGCTTTTGCGATGAATTTAG-3′ and a reverse primer containing the XbaI site, 5′-TCACTCTAGACCTGATGGCCTATTGCTCT-3′. The amplified DNA was digested with BamHI and XbaI, then inserted into the same sites of pTrcHis30. pKN7 was constructed by inserting the 2.8-kb HindIII fragment of pJK286-rth+ cdsA+ into the same site in pUC118. pEDA5 was constructed by inserting the 1.2-kb EcoRV fragment of pKN7 into the HincII site of pCL1920.
Table 1. Bacterial strains used in this study.
Figure 2. Restriction maps of the plasmids used in this study. Boxes denote the vector regions. The small arrowheads and the gray areas in the boxes denote the promoters and vector-derived coding regions for hexahistidine tags. Large solid arrows denote the coding regions of the ispA, ispB, and ispU/rth genes. Small arrows denote primer binding sites for PCR. Abbreviations: E, EcoRI site; H, HindIII site; S, SalI site; V, EcoRV site; Plac, lac promoter; Ptrc, trc promoter.
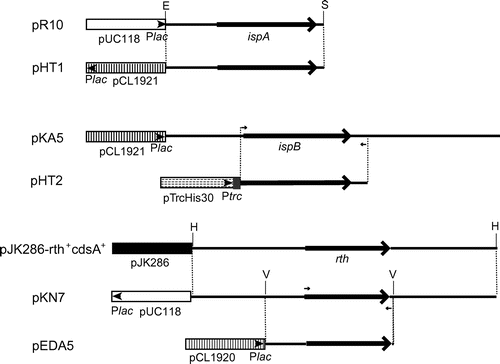
Chemicals
[1-14C]IPP (specific activity 2.04 TBq/mol) was purchased from Amersham Co. Unlabeled IPP, DMAPP, all-E-FPP, and all-E-geranylgeranyl diphosphate were purchased from Sigma Chemical Co. Ni-nitrilotriacetic acid agarose was a product of Qiagen Co.
Genetic and recombinant DNA procedures
The genetic and recombinant DNA procedures used were essentially based on the methods of Miller [Citation12] and Sambrook et al. [Citation13] P1kc was used for transductions [Citation12].
Measurement of the growth rate
Cells were grown in 5 mL of L-broth [Citation12] in glass tubes at 37˚C with shaking. Optical densities at 660 nm were measured at 30-min intervals.
Extraction and analysis of FPP
Cells grown in 100 or 300 mL of L-broth overnight were collected and washed with 0.1 M potassium phosphate buffer (pH 7.4). The cell pellets were resuspended in 1.0 mL of acetonitrile–methanol–water (4:4:2 v/v/v) containing 0.1 M formic acid (acidic extraction solution) [Citation14]. Dioctanoylglycerol diphosphate was added to the suspension as an internal standard. The mixture was stirred for 1.0 h at 4 °C using a magnetic stirrer. Following centrifugation, the supernatant was loaded onto an LC-NH2 column (Sigma Co.) equilibrated with acidic extraction solution. After the resin was washed with 2 mL of methanol-water (7:3 v/v), FPP and dioctanoylglycerol diphosphate were eluted with methanol-water (7:3 v/v) containing 4% ammonia. The eluted fraction was dried under nitrogen gas and dissolved in 500 μL of methanol, and 5 μL of this solution was injected into a LCMS-8040 (Shimadzu Co.) equipped with a reversed phase Purospher STAR RP-18 column (diameter, 2.0 mm; length, 125 mm; Merck Co.) for high-performance liquid chromatography (HPLC)-mass spectrometry (MS) analysis. The elution was performed by a gradient between 5 mM ammonium formate containing 0.1% triethylamine (solution A) and acetonitrile-water (4:1 v/v) containing 0.1% triethylamine (solution B) [Citation15]. The gradient was: 0–2 min, 0% B to 20% B; 2–3 min, 20% B to 50% B; 3–8 min, 50% B to 100% B; 8–9 min, 100% B; 9–9.1 min, 100% B to 0% B; 9.1–15 min, equilibration with 0% B. The flow rate was 0.2 mL/min. FPP eluted at 7.1 min (m/z of parent ion, 391.1; m/z of product ion, 79.0), and dioctanoylglycerol diphosphate eluted at 7.4 min (m/z of parent ion, 503.2; m/z of product ion, 79.0). The lower limit of quantification of FPP was 10 pg.
Extraction and analysis of isoprenoid quinones and undecaprenyl phosphate
Extraction and analysis of isoprenoid quinones and undecaprenyl phosphate were carried out as described previously [Citation2] with slight modifications. Cells grown in 10 mL of L-broth were collected and suspended in 2 mL of methanol-0.3% NaCl (10:1, v/v). Solutions of ubiquinone-10, phylloquinone, and solanesyl phosphate were added to the suspension as internal standards for the quantitation of ubiquinone, menaquinone, and undecaprenyl phosphate, respectively. The mixture was shaken with 2 mL of hexane for extraction of isoprenoid quinones. The upper hexane layer was collected, and the extraction was repeated twice more. Polyprenyl phosphates were extracted from the residual aqueous layer. To the aqueous layer was added 1 mL of 60% KOH, and the mixture was heated in a boiling water bath for 60 min. After the treatment with alkali, polyprenyl phosphates were extracted with diethyl ether. The diethyl ether extract was washed with 5% acetic acid. The hexane extract containing isoprenoid quinones was loaded onto a 0.4-g column of neutral alumina (grade III). Menaquinones were eluted with 2% diethyl ether in hexane, and ubiquinones were eluted with 6% diethyl ether in hexane. Isoprenoid quinones were analyzed by HPLC [Citation6]. HPLC-MS analysis was carried out using an LCMS-2010 system (Shimadzu Co.). An STR ODS-II column (diameter, 2.0 mm; length, 150 mm; Shinwa Chemical Ind. Ltd.) was used with 2-propanol-methanol (1:1 v/v), and the flow rate was 0.2 mL/min. Absorption of ultraviolet light (200–300 nm) was monitored by a photo diode array detector. Ionization was carried out using an electrospray ionization probe. Diethyl ether extract containing polyprenyl phosphate was dried under nitrogen gas, and dissolved in chloroform. The chloroform solution was loaded on a 0.3-g column of silicic acid. The column was washed with chloroform, and polyprenyl phosphates were eluted with chloroform-methanol (1:4 v/v). Polyprenyl phosphates were analyzed by HPLC.
Expression and purification of hexahistidine-tagged octaprenyl diphosphate synthase
E. coli SF5 cells harboring pHT2 were grown in Luria-Bertani [Citation12] medium at 37 °C. After addition of isopropyl β-D-thiogalactopyranoside (final concentration 0.1 mM), cells were grown for 2.5 h. Cells from 50 mL of medium were collected, washed with ice-cold 50 mM sodium phosphate buffer (pH 8.0) containing 300 mM NaCl and 10 mM imidazole, suspended in 4 mL of the same buffer, and disrupted by sonication. Purification of the hexahistidine-tagged IspB from the cell homogenate was carried out using a Ni-nitrilotriacetic acid agarose kit (Qiagen Co.) as per the manufacturer’s protocol.
Prenyl diphosphate synthase activity
The activities of purified IspB were measured as described previously [Citation6] with slight modifications. The reaction mixture for the condensation of IPP with allylic diphosphate contained, in a final volume of 0.2 mL, 1 μmol of MgCl2, 0.1% (w/v) Triton X-100, 10 μmol of potassium phosphate buffer (pH 7.5), 2 nmol of [1-14C]IPP, the indicated amount of allylic diphosphate, and 0.2 μg of the purified enzyme. After incubation for 60 min at 30 °C, 50 μL of 0.1 M EDTA trisodium salt (pH 8.0) were added to stop the enzyme reaction. The products of the reaction were extracted with 1-butanol, and the radioactivity in the extract was measured after washing the extract with water saturated with 1-butanol.
Analysis of the prenyl diphosphates
Prenyl diphosphates were treated with acid phosphatase as described previously [Citation16]. The resulting polyprenols were extracted with hexane and analyzed by normal phase and reversed phase thin layer chromatography (TLC). Normal-phase TLC was carried out on a silica gel 60 plate (Merck Co.) with benzene-ethyl acetate (4:1 v/v). Reversed phase TLC was carried out on an LKC-18 plate (Whatman Co.) with acetone-water (9:1 v/v). Radioactivity on the plates was detected using a BAS-1000 (Fujifilm Co.).
Results
Reconstruction of ispA null strain
An ispA null strain was reconstructed, because the ispA null mutant SF7 that was constructed in a previous study [Citation8] had very low efficiency of transformation. A transductant, W3110ΔispA::neo, was obtained by introduction of the mutation in SF7 into the chromosome of wild-type strain W3110 by P1-mediated transduction. Subsequently, the recA gene was inactivated by P1 transduction with SF7 as a donor, yielding W3110ΔispA::neo Δ(srl-recA)306::Tn10 (SF7N) (Table ). The transformation efficiency of SF7N was as good as that of the parental strain W3110. The presence of the insertionally inactivated ispA gene in the chromosome of SF7N was confirmed by PCR analysis (not shown).
Growth properties of ispA null mutant and its transformants
This transformation study was carried out using the newly constructed ispA null mutant, SF7N. The doubling time of SF7N was 61 min. The doubling times of transformants harboring pHT1 (which carries ispA) and pKA5 (which carries ispB) were 32 and 36 min, respectively, almost the same as the wild-type strain, SF5 (32 min) (Table ). Thus, the transformants of SF7N harboring medium-copy number plasmids that encode ispA or ispB grew as fast as the wild-type control strain, although the transformant of SF7N harboring a high-copy number plasmid carrying ispA, pHX1, grew almost the same as SF7N (not shown). The growth rate of the transformant harboring the medium-copy number plasmid pEDA5 that carries ispU/rth was lower than that of SF7N (Table ). The growth rates of transformants of wild-type strain SF5 harboring medium-copy number plasmids pHT1, pKA5, and pEDA5 were almost same as that of SF5 without any plasmid (not shown).
Table 2. Growth rates of strains and transformants used in this study.
Quantification of FPP in ispA null mutant and its transformants
The FPP level in the ispA null mutant SF7N was 25% of that in the wild-type strain, SF5 (Figure ). SF7N harboring pHT1 (which carries the ispA gene) contained almost the same level of FPP as SF5. The FPP level in SF7N harboring pKA5 (which carries ispB) was as low as that in SF7N. Thus, the FPP level was not restored by the overexpression of the ispB gene.
Figure 3. The level of farnesyl diphosphate (FPP) in strains and transformants used in this study. SF5 (the wild-type strain), SF7N (the ispA-null mutant), SF7N harboring pHT1 (which carries ispA), and SF7N harboring pKA5 (which carries ispB) were used for the quantification of FPP. Bar graphs show averages of three assays. Error bars show standard deviations. The statistical significance is indicated (*p < 0.05 compared with SF7N; **p < 0.01 compared with SF7N).
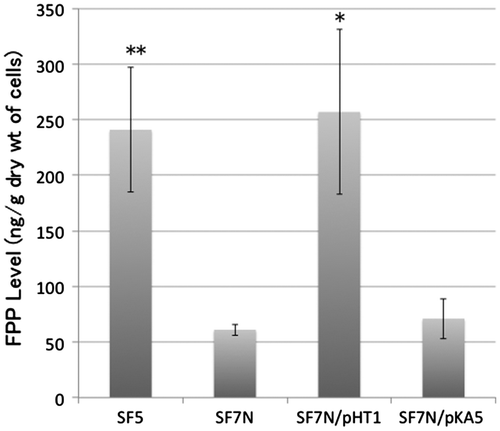
Analysis of isoprenoid quinones and undecaprenyl phosphate in ispA null mutant and its transformants
Levels of isoprenoid quinones were determined from the peak area ratio of ultraviolet absorbance. In the analysis of the ubiquinone fraction of SF7N, we observed a small absorbance peak (peak b) at 275 nm between peaks corresponding to ubiquinone-8 (peak a) and the internal standard ubiquinone-10 (peak c) (Figure (A)). HPLC-MS analysis was performed to identify this compound. The photodiode array spectrum of this compound showed that the compound was ubiquinone with λmax 275 nm. The mass spectrum of this compound showed an intense peak of m/z 817.7, corresponding to the expected monoisotopic mass of the sodium adduct ion ([M+Na]+) of ubiquinone-9 (Figure (B)). Thus, the compound giving rise to peak b was identified as ubiquinone-9.
Figure 4. High-performance liquid chromatography-mass spectrometry analysis of the ubiquinone fraction of SF7N. (A) Chromatogram of absorbance detected at 275 nm. Peak a, ubiquinone-8; peak b, ubiquinone-9; peak c, ubiquinone-10. (B) Mass spectrum of peak b.
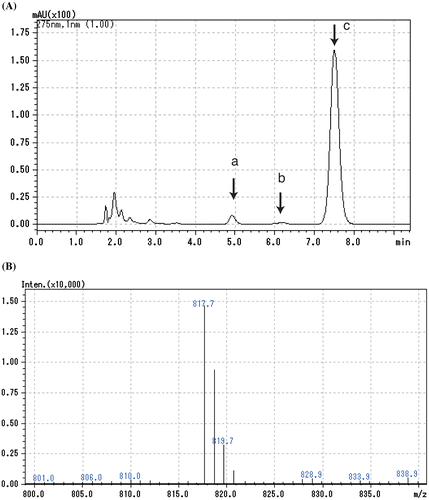
The levels of ubiquinone-8 and menaquinone-8 in SF7N harboring pKA5, which carries ispB, were 5-fold and 1.6-fold higher than those in SF7N harboring the empty vector, pCL1921, respectively, and were almost the same as those in SF7N harboring pHT1 (which carries ispA) (Figure ). The level of ubiquinone-9 in SF7N harboring pKA5 was higher than that in SF7N harboring the empty vector, though the ubiquinone-9 level in SF7N harboring pHT1 was the same as that in SF7N harboring the empty vector. The levels of isoprenoid quinones in SF7N harboring pEDA5, which carries ispU/rth, were lower than those in SF7N harboring the empty vector.
Figure 5. The level of isoprenoid quinones and undecaprenyl phosphate in transformants of ispA null mutant E. coli strain SF7N. The transformants of SF7N, harboring pCL1921 (empty vector), pHT1 (which carries ispA), pKA5 (which carries ispB), and pEDA5 (which carries ispU/rth) were used for the analysis of isoprenoids. Bar graphs show averages of three assays. Error bars show standard deviations. The statistical significance is indicated (**p < 0.01 compared with SF7N/pCL1921). Abbreviations: UQ-8, ubiquinone-8; UQ-9, ubiquinone-9; MK-8, menaquinone-8; UP, undecaprenyl phosphate.
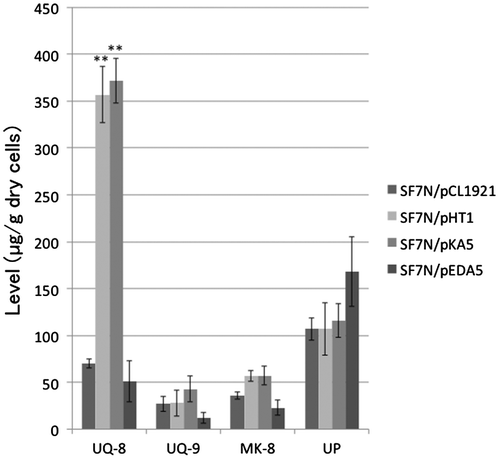
The undecaprenyl phosphate levels in the transformants harboring pKA5, pHT1, and the empty vector were almost the same as each other. The level of undecaprenyl phosphate in the transformant harboring pEDA5 was 1.6-fold higher than in SF7N harboring the empty vector. Thus, both the growth rate and the levels of isoprenoid quinones that have octaprenyl side chains were restored in the ispA null mutant by overexpression of the ispB gene, as well as by complementation with ispA.
Properties of hexahistidine-tagged octaprenyl diphosphate synthase
To examine whether octaprenyl diphosphate synthase, IspB, catalyzes the condensation of IPP with DMAPP to form FPP or other prenyl diphosphates, hexahistidine-tagged IspB was expressed in E. coli and purified. Protein with an apparent mass of 37 kDa was identified by SDS-PAGE. The most effective substrate for the purified enzyme was FPP, and the activity of the condensation of IPP with DMAPP was only 0.7% of that with FPP when the concentration of allylic diphosphate was 5 μM (Table ). The Km value for DMAPP that was obtained while holding the concentration of IPP constant (10 μM) was 340 μM. The Km value for IPP that was obtained while holding the concentration of DMAPP constant (250 μM) was 0.7 μM. To determine the products condensed from DMAPP, the reaction was performed using 10 μM of [1-14C]IPP and 250 μM of DMAPP. TLC analysis of the polyprenols obtained by phosphatase treatment of the reaction product revealed octaprenol (Rf = 0.52 in the normal phase TLC, Rf = 0.21 in the reversed phase TLC) and nonaprenol (Rf = 0.52 in the normal phase TLC, Rf = 0.15 in the reversed phase TLC) (Figure (A) and (B)). Thus, the reaction products of the condensation of IPP with DMAPP by the purified IspB were octaprenyl diphosphate and nonaprenyl diphosphate.
Table 3. Activity of hexahistidine-tagged octaprenyl diphosphate synthase.
Figure 6. Autoradiograms of thin layer chromatography (TLC) of prenyl alcohols obtained by enzymatic hydrolysis. Reaction of [1-14C]IPP and DMAPP was catalyzed by purified hexahistidine-tagged IspB. The products were extracted with butanol and treated with acid phosphatase. The resulting polyprenols were extracted with hexane and analyzed by normal phase TLC on a silica gel 60 plate using benzene-ethyl acetate (4:1 v/v) (A) and by reversed phase TLC on an LKC-18 plate using acetone-water (9:1 v/v) (B). Abbreviations: Ori, Origin; SF, solvent front; C15, E,E-farnesol; C20, E,E,E-geranylgeraniol; C45, solanesol (all-E-nonaprenol); C50, ficaprenol-50 (Z,E-mixed decaprenol); C55, ficaprenol-55 (Z,E-mixed undecaprenol); C60, ficaprenol-60 (Z,E-mixed dodecaprenol).
![Figure 6. Autoradiograms of thin layer chromatography (TLC) of prenyl alcohols obtained by enzymatic hydrolysis. Reaction of [1-14C]IPP and DMAPP was catalyzed by purified hexahistidine-tagged IspB. The products were extracted with butanol and treated with acid phosphatase. The resulting polyprenols were extracted with hexane and analyzed by normal phase TLC on a silica gel 60 plate using benzene-ethyl acetate (4:1 v/v) (A) and by reversed phase TLC on an LKC-18 plate using acetone-water (9:1 v/v) (B). Abbreviations: Ori, Origin; SF, solvent front; C15, E,E-farnesol; C20, E,E,E-geranylgeraniol; C45, solanesol (all-E-nonaprenol); C50, ficaprenol-50 (Z,E-mixed decaprenol); C55, ficaprenol-55 (Z,E-mixed undecaprenol); C60, ficaprenol-60 (Z,E-mixed dodecaprenol).](/cms/asset/7a443bfb-8007-4d70-8d8a-e4eb7d78dacb/tbbb_a_1398066_f0006_oc.gif)
Discussion
The ispA null mutant newly constructed in this study showed the same phenotype as an ispA mutant constructed in previous study [Citation8], for example, slow growth and low levels of isoprenoid quinones, which confirmed that these properties were due to the lack of the ispA gene. However, the transformation efficiency of the new mutant was as high as that of the parental strain, unlike for the ispA null mutant constructed previously, indicating that the low transformation efficiency was related to unidentified mutations in strain SF7, rather than to the lack of ispA.
Transformation study revealed that the slow growth of the ispA null mutant was rescued by complementation with ispA expressed from a medium-copy number plasmid. However, the growth rate of the transformant with a high-copy number plasmid that carried ispA was as low as that of the ispA null mutant. Thus, it was shown that an excess level of FPP synthase is deleterious to the growth of the cells. The level of FPP synthase should be within a certain range for optimal growth, though it is not clear whether the enzyme activity or the amount of the protein is critical. FPP is one of the substrates of two prenyl diphosphate synthases, octaprenyl diphosphate synthase and undecaprenyl diphosphate synthase [Citation17]. Overexpression of the genes for these prenyl diphosphate synthases gave contrasting results. Overexpression of ispB suppressed the slow growth of the ispA null mutant, while the growth rate of the transformant with a plasmid carrying ispU/rth was lower than that of the ispA null strain without any plasmid. These results suggest that the growth of the ispA null mutant is limited by a shortage of the products of octaprenyl diphosphate, such as isoprenoid quinones, rather than by a shortage of the products of undecaprenyl diphosphate. Actually, the level of ubiquinone-8 in the null mutant was significantly lower (10–20%) (p < 0.01) than that in the wild-type strain, while the level of undecaprenyl phosphate was only moderately lower (40–70%) in our previous study [Citation8].
This study showed that the phenotype of the ispA null mutant that lacked FPP synthase (IspA) was suppressed by overexpression of the ispB gene that encodes octaprenyl diphosphate synthase. The growth rate of the ispB-transformant was almost the same as that of the wild-type strain, and the levels of isoprenoid quinones were almost the same as those in the ispA null mutant complemented with the ispA gene. There are two possibilities for the action of octaprenyl diphosphate synthase in the ispB-transformant: IspB might catalyze the condensation of IPP with DMAPP to form octaprenyl diphosphate directly, or it might catalyze the condensation to form FPP or geranylgeranyl diphosphate in certain conditions. Purified, hexahistidine-tagged IspB could condense IPP with DMAPP, though the Km value for DMAPP was 2,000-fold higher than that for FPP [Citation17]. The reaction products were octaprenyl diphosphate and nonaprenyl diphosphate, and conditions in which FPP or geranylgeranyl diphosphate were formed could not be found. It is possible that suppression of the slow growth of the ispA mutant by ispB was due to condensation of IPP not only with FPP but also with DMAPP by octaprenyl diphosphate synthase, resulting in the observed increase in the levels of isoprenoid quinones.
Further study is necessary to elucidate the origin of FPP in the ispA null mutant. A small amount of FPP was detected in strain SF7N by HPLC-MS analysis. Though the growth rate and the isoprenoid quinone levels of the ispA null mutant were restored by overexpression of the ispB gene, the FPP level was not markedly increased. We could not detect the formation of FPP by purified hexahistidine-tagged IspB. However, an enzyme that showed FPP and geranylgeranyl diphosphate forming activity was separated from the homogenate of the previously constructed ispA null mutant, albeit the activity was low [Citation18]. It is possible that this enzyme was generated by a modification of the ispB product, octaprenyl diphosphate synthase, such as limited proteolysis, covalent attachment of a small molecule, or oligomer formation with (an)other polypeptide(s). In particular, oligomer formation is likely, because IspB is known to form complex with one of different subunits of yeast heteromeric decaprenyl diphosphate synthase to be stabilized [Citation19]. The product specificities of geranyl diphosphate synthase in mint [Citation20] and geranylgeranyl diphosphate synthase in fission yeast [Citation21] are reported to be changed by the oligomer formation with other proteins. A study of the crystal structure of hexaprenyl diphosphate synthase showed that the substrate and product binding pocket started in one subunit and reached another subunit [Citation22], which might explain the change of product specificity on oligomer formation.
Author contribution
SF designed research. HT, YA, YO, YM, KN, MI, and MS performed experiments and analyzed the data. SF wrote the manuscript. KN helped to write the manuscript.
Disclosure statement
No potential conflict of interest was reported by the authors.
Acknowledgments
We thank Dr Yukinobu Nishimura (Toho University) for valuable discussions, and Dr. Atsushi Fukumoto and Dr Yojiro Anzai (Toho University) for help with HPLC-MS analysis. We are also grateful to Ms Mayuko Takahashi for plasmid construction. We thank James Allen, DPhil, from Edanz Group (www.edanzediting.com/ac) for editing a draft of this manuscript.
References
- Ogura K, Koyama T. Enzymatic aspects of isoprenoid chain elongation. Chem Rev. 1998;98:1263–1276.10.1021/cr9600464
- Fujisaki S, Nishino T, Katsuki H, et al. Isolation and characterization of an Escherichia coli mutant having temperature-sensitive farnesyl diphosphate synthase. J Bacteriol. 1989;171:5654–5658.10.1128/jb.171.10.5654-5658.1989
- Fujisaki S, Hara H, Nishimura Y, et al. Cloning and nucleotide sequence of the ispA gene responsible for farnesyl diphosphate synthase activity in Escherichia coli. J Biochem. 1990;108:995–1000.10.1093/oxfordjournals.jbchem.a123327
- Asai K, Fujisaki S, Nishimura Y, et al. The identification of Escherichia coli ispB (cel) gene encoding the octaprenyl diphosphate synthase. Biochem Biophys Res Commun. 1994;202:340–345.10.1006/bbrc.1994.1933
- Apfel CM, Takacs B, Fountoulakis M, et al. Use of genomics to identify bacterial undecaprenyl pyrophosphate synthetase: cloning, expression, and characterization of the essential uppS gene. J Bacteriol. 1999;181:483–492.
- Kato J, Fujisaki S, Nakajima K, et al. The Escherichia coli homologue of yeast RER2, a key enzyme of dolichol synthesis, is essential for the carrier lipid formation in bacterial cell wall synthesis. J Bacteriol. 1999;181:2733–2738.
- Okada K, Minehira M, Zhu X, et al. The ispB gene encoding octaprenyl diphosphate synthase is essential for growth of Escherichia coli. J Bacteriol. 1997;179:3058–3060.10.1128/jb.179.9.3058-3060.1997
- Fujisaki S, Takahashi I, Hara H, et al. Disruption of the structural gene for farnesyl diphosphate synthase in Escherichia coli. J Biochem. 2005;137:395–400.10.1093/jb/mvi049
- Krute CN, Carroll RK, Rivera FE, et al. The disruption of prenylation leads to pleiotropic rearrangements in cellular behavior in Staphylococcus aureus. Mol Microbiol. 2015;95:819–832.10.1111/mmi.2015.95.issue-5
- Lerner CG, Inouye M. Low copy number plasmids for regulated low-level expression of cloned genes in Escherichia coli with blue/white insert screening capability. Nucleic Acids Res. 1990;18:4631.10.1093/nar/18.15.4631
- El Ghachi M, Bouhss A, Blanot D, et al. The bacA gene of Escherichia coli encodes an undecaprenyl pyrophosphate phosphatase activity. J Biol Chem. 2004;279:30106–30113.10.1074/jbc.M401701200
- Miller JH, editor. A short course in bacterial genetics: a laboratory manual and handbook for Escherichia coli and related bacteria. Cold Spring Harbor (NY): Cold Spring Harbor Laboratory Press; 1992.
- Sambrook J, Fritsch EF, Maniatis T. Molecular cloning: a laboratory manual. 2nd ed. Cold Spring Harbor (NY): Cold Spring Harbor Laboratory Press; 1989.
- Zhou K, Zou R, Stephanopoulos G, Too HP. Metabolite profiling identified methylerythritol cyclodiphosphate efflux as a limiting step in microbial isoprenoid production. PLoS ONE. 2012;7:e47513.10.1371/journal.pone.0047513
- Henneman L, van Cruchten AG, Denis SW, et al. Detection of non-sterol isopreneoids by HPLC-MS/MS. Anal Biochem. 2008;383:18–24.10.1016/j.ab.2008.08.023
- Fujii H, Koyama T, Ogura K. Efficient enzymatic hydrolysis of polyprenyl pyrophosphate. Biochim Biophys Acta. 1982;712:716–718.
- Fujisaki S, Nishino T, Katsuki H. Isoprenoid synthesis in Escherichia coli. Separation and partial purification of four enzymes involved in the synthesis. J Biochem. 1986;99:1327–1337.10.1093/oxfordjournals.jbchem.a135600
- Saito K, Fujisaki S, Nishino T. Short-chain prenyl diphosphate synthase that condenses isopentenyl diphosphate with dimethylallyl diphosphate in ispA null Escherichia coli strain lacking farnesyl diphosphate synthase. J Biosci Bioeng. 2007;103:575–577.10.1263/jbb.103.575
- Cui T-Z, Kaino T, Kawamukai M. A subunit of decaprenyl diphosphate synthase stabilizes octaprenyl diphosphate synthase in Escherichia coli by forming a high-molecular weight complex. FEBS Lett. 2010;584:652–656.10.1016/j.febslet.2009.12.029
- Burke C, Croteau R. Interaction with the small subunit of geranyl diphosphate synthase modifies the chain length specificity of geranylgeranyl diphosphate synthase to produce geranyl diphosphate. J Biol Chem. 2002;277:3141–3149.10.1074/jbc.M105900200
- Ye Y, Fujii M, Hirata A, et al. Geranylgeranyl diphosphate synthase in fission yeast is a heteromer of farnesyl diphosphate synthase (FPS), Fps1, and an FPS-like protein, Spo9, essential for sporulation. Mol Biol Cell. 2007;18:3568–3581.10.1091/mbc.E07-02-0112
- Sasaki D, Fujihashi M, Okuyama N, et al. Crystal structure of heterodimeric hexaprenyl diphosphate synthase from Micrococcus luteus B-P 26 reveals that the small subunit is directly involved in the product chain regulation. J Biol Chem. 2011;286:3729–3740.10.1074/jbc.M110.147991