Abstract
There are many reports about carotenoid-producing bacteria and carotenoid biosynthesis genes. In databases for Pseudomonas genome sequences, there are genes homologous to carotenoid biosynthesis genes, but the function of these genes in Pseudomonas has not been elucidated. In this study, we cloned the carotenoid biosynthesis genes from a Pseudomonas sp. strain, named Akiakane, which was isolated from the excrement of the Autumn Darter dragonfly. Using an Escherichia coli functional expression system, we confirmed that the idi, crtE, crtB, crtI, and crtY gene products of the Akiakane strain show predictable catalytic activities. A cluster of six genes was also found, which was comparable to other carotenoid-producing bacteria that belong to the α-Proteobacteria or γ-Proteobacteria class.
The carotenoid biosynthesis genes of a Pseudomonas sp. atrain Akiakane isolated from the excrement of the Autumn Darter, were isolated and analyzed.
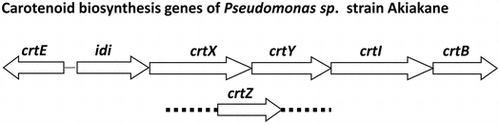
Strains belonging to the genus Pseudomonas of the class γ-Proteobacteria are one of the most ubiquitous aerobic bacteria in nature. Pseudomonas are gram-negative bacteria and have an important role in material circulation in ecosystems. In particular, Pseudomonas aeruginosa and P. syringae are well-known invasive pathogens for animals (including humans) and higher plants, respectively, and P. fluorescens lives in the rhizosphere nourishing plants [Citation1,2]. These Pseudomonas strains have yellow color because of carotenoid biosynthesis. Therefore, it is possible that carotenoids protect Pseudomonas strains from photo-oxidative damage. In Pseudomonas genome sequence databases, there are homologs to carotenoid biosynthesis genes. However, the structure and function of Pseudomonas carotenoid biosynthesis genes have not been reported.
Carotenoids are natural pigments composed of isoprene units and are produced by some bacteria, archaea, and fungi as well as all plants and algae. More than 750 species of carotenoids have been isolated from nature. Recent work showed that carotenoids have anti-cancer and anti-oxidation effects [Citation3]. Carotenoids are also used for cosmetics, health foods, and supplements [Citation4]. Because most carotenoids exist in only trace amounts in nature, construction of a mass production system for carotenoids is needed for further functional testing.
Carotenoids produced by bacteria are diverse, even though only some bacteria can biosynthesize carotenoids [Citation5–7]. For example, Paracoccus sp. strain N81106 and Brevundimonas sp. strain SD212 belonging to the same α-Proteobacteria class were reported to synthesize astaxanthin β-D-glucoside and 2-hydroxyastaxanthin, respectively, in addition to astaxanthin that has strong antioxidant activity [Citation8,9]. The typical carotenoid biosynthesis pathway (i.e. for the α-Proteobacteria and γ-Proteobacteria classes) begins with the reaction of IPP (C5) isomerization to DMAPP (C5) by IPP isomerase (Idi). Afterwards, GPP (C10), FPP (C15), and GGPP (C20) are synthesized. Synthesis of GGPP is catalyzed by GGPP synthase (CrtE). Phytoene (C40) is further synthesized from two GGPP molecules by phytoene synthase (CrtB). Phytoene desaturase (CrtI) and lycopene β-cyclase (CrtY) synthesize lycopene from phytoene and β-carotene from lycopene (via γ-carotene), respectively.
Some bacteria belonging to the γ-Proteobacteria class (e.g. Pantoea ananatis formerly Erwinia uredovora strain 20D3, P. agglomerans formerly Erwinia herbicola strain Eho10, and Cronobacter sakazakii strain BAA 894) produce carotenoids, and their carotenoid biosynthesis (crt) genes have been elucidated [Citation10–12] Pseudomonas also belongs to γ-Proteobacteria and has crt genes, but the functions of these genes have not been analyzed. In this study, we isolated a Pseudomonas sp. strain named Akiakane from Autumn Darter excrement. It is interesting whether Autumn Darter uses the carotenoids produced by the Pseudomonas sp. strain Akiakane. So, we cloned the carotenoid biosynthesis genes from this Pseudomonas strain, and mainly carried out their functional analysis.
Materials and methods
Isolation of the Pseudomonas sp. strain Akiakane
Serial dilutions of Autumn Darter (Sympetrum frequens) excrement were spread on tryptone soya agar (Eiken Chemical, Tokyo, Japan) to isolate the carotenoid-producing strains. The media were incubated at 30 °C for 3 days under the aerobic condition. Although the yellow colonies were infrequently observed, one colony with the yellow color from 3.9 × 105 colonies was selected. The strain was designated as Pseudomonas sp. strain Akiakane by sequencing of 16S ribosomal RNA (rRNA) as described below.
Extraction of genomic DNA from strain Akiakane
The Pseudomonas sp. strain Akiakane was grown in 2YT medium with shaking at 37 °C overnight and the culture was centrifuged for 2 min. Genomic DNA was extracted from the bacterial pellet by the CTAB method [Citation13].
Cloning of carotenoid biosynthesis genes and the 16S rRNA gene
Based on the genome sequences of P. parafulva strain NS96 (GenBank accession No. NZ_LDSN01000016), primers were designed to isolate carotenoid biosynthesis genes (idi, crtE, crtB, crtI, crtY, crtZ, and crtX) by PCR (Table ) [Citation14]. The 16S rRNA gene was amplified using the gene-specific primers, 27F and 1492R (Table ) [Citation15]. The PCR products were cloned into a plasmid vector, transformed into E. coli (DH5α), and sequenced.
Table 1. Primers used in this study.
Sequence analysis
DNA sequences were analyzed using DNASIS DNA analysis software (Hitachi Solutions, Tokyo, Japan). Homology search was performed by BLAST (http://blast.ncbi.nlm.nih.gov/Blast.cgi) [Citation16]. DNA sequences were aligned by CLUSTAL W (http://clustalw.ddbj.nig.ac.jp) [Citation17], and a phylogenetic tree was constructed using Tree View (http://taxonomy.zoology.gla.ac.uk/rod/treeview.html).
Inverse PCR
To isolate the flanking region of the crt genes, inverse PCR was performed. One microgram of genomic DNA from the Akiakane strain was each digested with SalI, EcoRI, or NcoI. Digested DNA (0.1 μg/μL) was self-ligated with ligation solution (Takara, Kyoto, Japan). PCR was performed using 50 pg of these circular DNAs as the template in 25 μL of the reaction solution with the gene-specific primers (Table ). The amplified fragments were cloned into pGEMTeasy vector (Promega, WI, USA) and sequenced.
Expression of the Akiakane strain crt genes in recombinant E. coli
As described above, crtE, crtB, crtI, crtY, idi and crtZ were amplified using the gene-specific primers which include the restriction sites as shown in Table . The amplified crtE, crtB, crtI, and crtY fragments were digested with EcoRI and NotI and inserted between the EcoRI and NotI sites of pETDuet vector (Merk, Darmstadt, Germany). The amplified idi fragment was digested with SalI and BamHI and cloned into the SalI and BamHI sites of pRK404 vector. The amplified crtZ fragment was digested with EcoRI and NotI and cloned into the EcoRI and NotI sites of pUC18 vector [Citation18]. These plasmids were named for pETD-crtE, pETD-crtB, pETD-crtI, pETD-crtY, pRK-idi, and pUC-crtZ. Each plasmid was introduced into the carotenoid-producing E. coli JM101(DE3). The pETD-crtE, pETD-crtB, and pETD-crtI vectors were introduced into E. coli containing pACCAR25ΔcrtEX, which expresses the crtB, crtI, crtY, and crtZ genes derived from Pantoea ananatis, pACCAR25ΔcrtB, which expresses the crtE, crtI, crtY, crtZ, and crtX genes, and pACCRT-EB, which expresses the crtE and crtB genes, respectively (Figure ) [Citation19–21]. The pETD-crtY and pRK-idi vectors were introduced into E. coli containing pACCRT-EIB, which express the crtE, crtB, and crtI genes (Figure ) [Citation21]. The pUC-crtZ vector was introduced into E. coli containing pACHP-Beta, which expresses the crtE, crtB, crtI, crtY genes, and the Haematococcus pluvialis idi gene [Citation22]. Empty vectors were also introduced into E. coli for a negative control.
Figure 1. Schematic representation of plasmids used for E. coli expression system. crtE, GGPP synthase gene from Pantoea ananatis; crtB, phytoene synthase gene from P. ananatis; crtI, phytoene desaturase gene from P. ananatis; crtY, lycopene β-cyclase gene from P. ananatis; crtZ, β-carotene hydroxylase gene from P. ananatis; crtX, zeaxanthin glucosyltransferase from P. ananatis; Cmr, Chloramphenicol resistance gene.
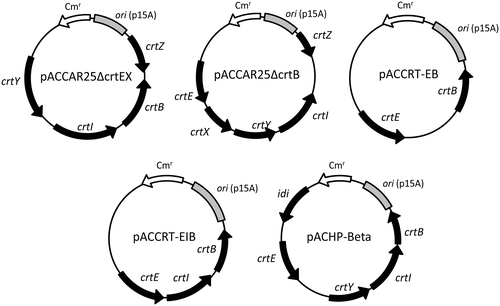
Growth condition of strain Akiakane and recombinant E. coli for carotenoid analysis
The Akiakane strain and each recombinant E. coli were grown in 2YT medium at 37 °C for 16 h. Then, this culture was inoculated into new 2YT medium and cultured at 20 °C for 48 h.
Carotenoid extraction from E. coli and Akiakane cells, and HPLC-PDA analysis
The bacterial cultures were centrifuged and 100 μL of methanol was added to the cell pellets. Fifty microliters of 50 mM Tris-HCl (pH7.5), 150 μL of 1 M NaCl, and 200 μL of chloroform were added to the solution, mixed for 5 min, and centrifuged. The lower phase was collected and dried by centrifugal evaporation. The pellet was suspended with ethyl acetate and analyzed using HPLC-PDA. HPLC analysis was performed using Waters Alliance 2695–2996 system with a photo diode array detector as described previously [Citation22]. A TSKgel ODS-80s column (4.6 × 150 nm, 5 μg; Tosoh) was used. Samples were eluted at a flow rate of 1.0 ml/min at 25 °C with two solvents—solvent A (water–methanol, 5:95, v/v) and solvent B (tetrahydrofuran–methanol, 30:70, v/v). Solvent A was perfused for 5 min, followed by a linear gradient from solvent A to solvent B for 5 min, and then solvent B alone for 8 min. Carotenoids were identified by comparing retention times and absorbing spectra with authentic standards (Table ). All chromatograms are shown with each peak at its λmax (Maxplot).
Table 2. Retention time and absorbing spectra of the authentic standards.
Statistical analysis
For statistical analysis, we performed Student’s t test to understand the difference between the E. coli transformed by crt genes and E. coli transformed by empty vector.
Results
Carotenoids in the Akiakane strain
We analyzed and extracted carotenoids by HPLC to investigate the carotenoid profile of the Akiakane strain. Two peaks were detected (Figure ) and identified as β-carotene at 14.7 min. and β-cryptoxanthin at 11.4 min. The amount of β-carotene and β-cryptoxanthin were 7.29 ± 1.78 and 1.28 ± 0.42 μg/gFW, respectively. This result indicates that the Akiakane strain synthesizes these carotenoids and has carotenoid biosynthesis genes.
Phylogenetic analysis of the Akiakane strain
To reveal the phylogenetic relationship of the Akiakane strain with other Pseudomonas, the sequences of the 16S rRNA gene were compared (Figure). The DNA sequences of Akiakane strain was 99.9% homologous to both those of the P. fulva strain NRIC 0180 and the P. parafulva strain DSM 17004, suggesting that the Akiakane strain belongs to P. putida group [Citation23,24]. However, the DNA and amino acid sequences of carotenoid biosynthesis genes isolated from Akiakane strain significantly differed from those of any other known Pseudomonas including P. putida group as described later. Therefore, we named this Pseudomonas sp. strain Akiakane.
Isolation of carotenoid biosynthesis genes from the Akiakane strain
Based on the carotenoid profile from the Akiakane strain, we predicted the carotenoid biosynthesis pathway (Figure ). In this study, zeaxanthin di-glucoside was not detected in Pseudomonas sp. strain Akiakane. However, crtX homologs existed in the genome of several Pseudomonas, we tried to find crtX. To isolate the genes encoding idi, crtE, crtB, crtI, crtY, crtZ, and crtX, we carried out PCR cloning of each gene. Six genes—idi, crtE, crtB, crtI, crtY, crtX—were amplified but not crtZ. Because the sequences of the designed reverse primer (PAcrtZ R1:5′-CGCGGCCGCTCAGAGAGCAGAGCCCCT-3′) were significantly different from the genomic sequences of the Akiakane strain. Therefore, we constructed another primer for crtZ (PAcrtZ R2:5′-CGCGGCCGCTCAGTGACCGGCGTCCC-3′) and carried out PCR cloning, which resulted in isolation of crtZ. The accession numbers for idi, crtE, crtB, crtI, crtY, crtX genes, and for crtZ gene are LC317091 and LC317092, respectively (Supplementary Figure ). The amino acid sequences of crtE, crtB, crtI, crtY, crtZ, and crtX coding proteins showed 43, 56, 74, 54, 49, and 48% homologies to those of Pantoea ananatis, respectively. The amino acid sequences of idi coding protein showed 63% homology to that of Pantoea sp. PSNIH2. These identities suggested that the isolated genes had the activities of corresponding enzymes. As a result of the comparison with other bacteria, the amino acid sequences of crtY coding protein are most homologous (100%) to that of P. fulva. The amino acid sequences of crtB, crtX and idi coding proteins showed the highest homologies, 96, 99 and 97%, respectively, to those of P. parafulva On the other hand, the amino acid sequences of crtE, crtI and crtZ coding protein were up to 98, 99 and 97% homologous to those of P. putida, respectively. These results also suggest that the Akiakane strain belongs to the P. putida group. When the DNA sequences of the Akiakane carotenoid biosynthesis genes are compared to those of other Pseudomonas, all of them showed highest homologies (92–95%) to those of P. parafulva. These results suggest that the Akiakane strain is most closely related to P. parafulva, but different from any other known Pseudomonas.
CrtE has GGPP synthase activity
GGPP is synthesized from FPP by CrtE (GGPP synthase). Subsequently, carotenoids (phytoene, lycopene, β-carotene and zeaxanthin) downstream of GGPP are able to be synthesized. To investigate the enzymatic activity of Akiakane CrtE, we used recombinant E. coli transformed with plasmid pACCAR25ΔcrtEX [E. coli (pACCAR25ΔcrtEX)], which includes crtB, crtI, crtY, and crtZ. Because this E. coli has low GGPP synthase activity, it produces little zeaxanthin and turns light yellow. When the empty pETD vector was introduced into E. coli (pACCAR25ΔcrtEX), the transformed E. coli was light yellow and had little zeaxanthin (Figure (a), peak 1). In contrast, when the pETD-crtE was introduced into E. coli (pACCAR25ΔcrtEX), the resulting recombinant E. coli was yellow, and HPLC analysis of the pigments showed that zeaxanthin was abundantly produced (Figure (b), peak 1). We performed Student’s t test, and there was a significant difference p < 0.01 between empty pETD vector and the pETD-crtE (Table ). These results strongly suggest that Akiakane CrtE has GGPP synthase activity.
Figure 5. HPLC analysis of carotenoids produced by the recombinant E. coli. HPLC chromatograms of the extracts from E. coli that carried (a) pACCAR25ΔcrtEX plus pETD vector; (b) ACCAR25ΔcrtEX plus pETD-crtE; (c) pACCAR25ΔcrtB plus pETD vector; (d) pACCAR25ΔcrtB plus pETD-crtB. 1; zeaxanthin, 2; β-cryptoxanthin.
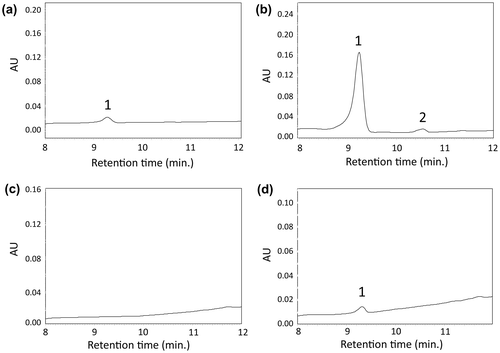
Table 3. Carotenoids produced by recombinant E. coli.
CrtB has phytoene synthase activity
CrtB catalyzes the reaction from GGPP to phytoene. To analyze the function of Akiakane CrtB, we used E. coli containing the plasmid pACCAR25ΔcrtB, which includes the crtE, crtI, crtY, crtZ, and crtX genes. When we introduced empty vector pETD into this E. coli, the transformed E. coli were white. In contrast, when pETD-crtB was introduced, E. coli turned light yellow. Furthermore, HPLC analysis showed that E. coli (pETD-crtB and pACCAR25ΔcrtB), but not pETD (instead of pETD-crtB), produced zeaxanthin detected at 9.1 min. of retention time (Figure (c) and (d)). These results indicate that Akiakane CrtB has phytoene synthase activity.
CrtI and CrtY has phytoene desaturase and lycopene β-cyclase activity, respectively
To analyze CrtI activity, pETD-crtI was introduced into the phytoene-producing E. coli, which contains pACCRT-EB. The recombinant E. coli were pink, whereas E. coli transformed with empty vector pETD were white. HPLC analysis showed that E. coli (pETD and pACCRT-EB) produced only phytoene detected at 16.4 min. (Figure (a)), while E. coli (pETD-crtI and pACCRT-EB) produced lycopene at 14.0 min. (Figure (b)). These results indicate that Akiakane CrtI has phytoene desaturase activity.
Figure 6. HPLC analysis of carotenoids produced by the recombinant E. coli. HPLC chromatograms of the extracts from from E. coli that carried (a) pACCRT-EB plus pETD vector; (b) pACCRT-EB plus pETD-crtI; (c) pACCRT-EIB plus pETD vector; (d) pACCRT-EIB plus pETD-crtY. Individual peak of 1; phytoene, 2; lycopene, 3; β-carotene.
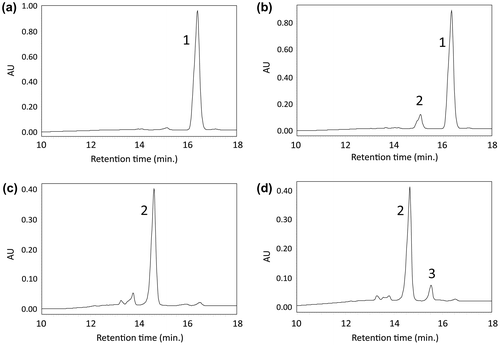
Next, we investigated CrtY activity using lycopene-producing E. coli, which contains pACCRT-EIB. E. coli transformed with empty vector pETD were pink – the color of lycopene (Figure (c), peak 2). On the other hand, E. coli transformed with pETD-crtY were orange—the color of β-carotene (Figure (d), peak 3). These results indicate that Akiakane CrtY has lycopene β-cyclase activity.
Idi has IPP isomerase activity
The pRK-idi and pRK404 empty vector were introduced into E. coli containing pACCRT-EIB [Citation25]. Measurement of lycopene showed that E. coli transformed with pRK-idi produced approximately 3-fold more lycopene compared to E. coli transformed with pRK404 (Figure ). This result strongly suggests that Akiakane Idi has IPP isomerase activity, since this catalytic characteristic is typical to that of IPP isomerase.
CrtZ does not have β-carotene hydroxylase activity in E. coli
To analyze the activity of Akiakane CrtZ, pETD-crtZ was introduced into E. coli containing the pACHP-Beta plasmid, which produces β-carotene. This recombinant E. coli did not produce zeaxanthin similar to that of E. coli transformed with empty vector pETD (data not shown). We used another vector, such as pUC, and another host E. coli, such as BL21 (DE3), to express crtZ, but we could not detect Akiakane CrtZ activity (data not shown).
Carotenoid biosynthesis genes cluster
Clusters of carotenoid biosynthesis genes exist in some carotenoid-producing bacteria. To understand whether carotenoid genes in Akiakane also form a cluster, we sequenced the genomic region around these genes and found that idi, crtX, crtY, crtI, and crtB form a cluster (Figure ). On the other hand, the crtZ gene was not included in this cluster and was located between a peroxidase and a magnesium transporter coding gene. The crtE gene is located in the opposite direction of the other genes in the cluster. This suggests that the expression of crtE and crtZ are independently controlled from the genes in the cluster.
The start codons of crtX, crtI, and crtB genes overlapped with the stop codons of the idi, crtY, and crtI genes, respectively (Supplementary Figure ). The start codon of the crtY gene was predicted as GTG and found in the crtX gene coding sequence (Supplementary Figure ). Consequently, the stop codon of the crtX gene was located in crtY gene coding sequence. These results suggest the idi, crtX, crtY, crtI, and crtB genes are transcribed together.
Discussion
In this study, we isolated a new Pseudomonas strain named Akiakane from the excrement of the Autumn Darter dragonfly. This Pseudomonas strain produced typical β-carotenoids, such as β-carotene and β-cryptoxanthin. This suggests that this bacterium has carotenoid biosynthesis genes similar to other carotenoid-producing bacteria that belong to the α-Proteobacteria or γ-Proteobacteria class.
Phylogenetic analysis of the 16S rRNA gene of this Pseudomonas suggested that it may be a different species from other known Pseudomonas. Although it is not clear whether this is a new species or not, we called it “Akiakane” in this paper. The sequences of the crt genes cloned here were most similar to those of P. parafulva but significantly different from those of other reported Pseudomonas genome sequences. This raises the possibility that the Akiakane strain is a new species which belongs to P. putida group.
We isolated seven genes homologous to known carotenoid biosynthesis genes. Five of the seven genes – idi, crtE, crtB, crtI, and crtY – had functional enzyme activities as expected. In contrast, we did not detect CrtZ activity in E. coli. There are several possibilities for this result. First, the Akiakane crtZ gene may have no activity, but crtZ is not thought to be a pseudogene from its sequence. Holger et al. (2011) reported that P. putida KT2440 has a crtZ homolog that has no β-carotene hydroxylase activity [Citation26]. In our case, β-cryptoxanthin was present in Akiakane. Therefore, another gene might have β-carotene 3,3′-hydroxylase activity. For example, Synechocystis sp. PCC6803 CrtR and Themus thermophiles HB27 CYP175A1 are reported to have β-carotene hydroxylase activity [Citation27,28]. However, genes homologous to crtR or CYP175A1 have not yet been found in known Pseudomonas genomes. Alternatively, Akiakane crtZ may not function in a heterologous system like E. coli.
Pseudomonas sp. strain Akiakane accumulated β-carotene and β-cryptoxanthin as the major carotenoids but not zeaxanthin. This result suggests that Akiakane β-carotene hydroxylase prefers the first conversion step from β-carotene to β-cryptoxanthin rather than the second reaction from β-cryptoxanthin to zeaxanthin as in the case of Arabidopsis CHYB [Citation29].
We found a carotenoid gene cluster in the Akiakane genome. Similar clusters have also been reported in carotenoid-producing bacteria that belong to the α-Proteobacteria or γ-Proteobacteria class (Figure ). Pseudomonas belongs to the γ-Proteobacteria class that is same to Pantoea ananatis and Cronobacter sakazakii. The structure of the Akiakane gene cluster was comparable to other γ-Proteobacteria. In particular, the order and direction of crtX, crtY, crtI, and crtB are highly conserved. In contrast, the locations of the crtE, idi, and crtZ genes differ between species. Carotenoid gene clusters also exist in α-proteobacteria like Paracoccus marcusii and Brevundimonas sp. SD212. The organizations of crtY, crtI, and crtB are also conserved, which suggests that the order of these genes might be important for their function.
In this study, we showed that the Pseudomonas sp. strain Akiakane can produce carotenoids. This is the first report to reveal that the carotenoid biosynthesis genes of Pseudomonas are functional. It also raises the possibility that Autumn Darter uses the carotenoids produced by the Pseudomonas sp. strain Akiakane and further study is needed to understand this interaction.
Author contribution
MT designed the study and performed the cloning of genes. YF carried out the cloning and the functional analysis of the carotenoid biosynthesis genes. TK isolated the Pseudomonas sp. Akiakane. TM and KS analyzed the carotenoids of Pseudomonas sp. Akiakane. NM coordinated collaborations and discussed on the data. YF and MT wrote the paper.
Disclosure statement
No potential conflict of interest was reported by the authors.
Funding
This work was partially supported by New Energy and Industrial Technology Development Organization (NEDO).
Supplemental data
Supplemental data for this article can be accessed at https://doi.org/10.1080/09168451.2017.1398069.
supplementaryFig1.docx
Download MS Word (26.9 KB)Acknowledgments
We thank Mrs. Fuchimoto for her technical support.
References
- Alrahman MA, Yoon SS. Identification of essential genes of Pseudomonas aeruginosa for its growth in airway mucus. J Microbiol. 2017;55(1):68–74.10.1007/s12275-017-6515-3
- Kato J, Kim HE. Chemotaxis in environmental bacteria: review of chemotaxis researches in Pseudomonas. J Environ Biotechnol. 2007;7(2):93–98.
- Maoka T. Biochemical functions of carotenoids. Food Clin Nutr. 2007;2:3–14.
- Tominaga K, Hongo N, Karato M, et al. Cosmetic benefits of astaxanthin on human subjects. Acta Biochim Pol. 2012;59(1):43–47.
- Zhou Z, Zhang W, Su S, et al. CYP287A1 is a carotenoid 2-β-hydroxylase required for deinoxanthin biosynthesis in Deinococcus radiodurans R1. Appl Microbiol Biotechnol. 2015;99(24):10539–10546.10.1007/s00253-015-6910-9
- Hannibal L., Lorquin J., D’Ortoli NA, et al. Isolation and characterization of canthaxanthin biosynthesis genes from the photosynthetic bacterium Bradyrhizobium sp. strain ORS278. J Bacteriol. 2000;182(13):3850–3853.10.1128/JB.182.13.3850-3853.2000
- Tao L, Yao H, Kasai H, et al. A carotenoid synthesis gene cluster from Algoriphagus sp. KK10202C with a novel fusion-type lycopene beta-cyclase gene. Mol Genet Genom. 2006;276(1):79–86.10.1007/s00438-006-0121-0
- Maruyama T, Kasai H, Choi SK, et al. Structure of a complete carotenoid biosynthesis gene cluster of marine bacterium Paracoccus sp. strain N81106. Carotenoid Sci. 2007;11:50–55.
- Park JS, Chyun JH, Kim YK, et al. Astaxanthin decreased oxidative stress and inflammation and enhanced immune response in humans. Nutr Metab. 2010;7(1):18:1–10. 10.1186/1743-7075-7-18.
- Misawa N, Nakagawa M, Kobayashi K, et al. Elucidation of the Erwinia uredovora carotenoid biosynthetic pathway by functional analysis of gene products expressed in Escherichia coli. J Bacteriol. 1990;172(12):6704–6712.10.1128/jb.172.12.6704-6712.1990
- Hundle B, Alberti M, Nievelstein V, et al. Functional assignment of Erwinia herbicola Eho10 carotenoid genes expressed in Escherichia coli. Mol Gen Genet. 1994;245(4):406–416.10.1007/BF00302252
- Zhang W, Hu X, Wang L, et al. Reconstruction of the carotenoid biosynthetic pathway of cronobacter sakazakii baa894 in Escherichia coli. PLoS ONE. 2014;9(1):e86739.10.1371/journal.pone.0086739
- Wilson K. Preparation of genomic DNA from bacteria. VCurr Protoc Mo Biol. 2001;2.4.1–2.4.5.
- Midha S, Bansal K, Sharma S, et al. Genomic Resource of Rice Seed Associated Bacteria. Front Microbiol. 2015;6:1551:1–8. 10.3389/fmicb 2015.01551.
- Weisburg WG, Barns SM, Pelletier DA, et al. 16S ribosomal DNA amplification for phylogenetic study. J Bacteriol. 1991; 173(2):697–703.10.1128/jb.173.2.697-703.1991
- Altschul SF, Madden TL, Schäffer AA, et al. Gapped BLAST and PSI-BLAST: a new generation of protein database search programs. Nucleic Acids Res. 1997;25(17):3389–3402.10.1093/nar/25.17.3389
- Thompson JD, Higgins DG, Gibson TJ. CLUSTAL W: improving the sensitivity of progressive multiple sequence alignment through sequence weighting, position-specific gap penalties and weight matrix choice. Nucleic Acids Res. 1994;22(22):4673–4680.10.1093/nar/22.22.4673
- Ditta G, Schmidhauser T, Yakobson E, et al. Plasmids related to the broad host range vector, pRK290, useful for gene cloning and for monitoring gene expression. Plasmid 1985;13(2):149–153.10.1016/0147-619X(85)90068-X
- Sandmann G, Misawa N, Wiedemann M, et al. Functional identification of al-3 from Neurospora crassa as the gene for geranylgeranyl pyrophosphate synthase by complementation with crt genes, in vitro characterization of the gene product and mutant analysis. J Photochem Photobiol B. 1993;18(2–3):245–251.10.1016/1011-1344(93)80071-G
- Chamovitz D, Misawa N, Sandmann G, et al. Molecular cloning and expression in Escherichia coli of a cyanobacterial gene coding for phytoene synthase, a carotenoid biosynthesis enzyme. FEBS Lett. 1992;296(3):305–310.10.1016/0014-5793(92)80310-D
- Misawa N, Satomi Y, Kondo K, et al. Structure and functional analysis of a marine bacterial carotenoid biosynthesis gene cluster and astaxanthin biosynthetic pathway proposed at the gene level. J Bacteriol. 1995;177(22):6575–6584.10.1128/jb.177.22.6575-6584.1995
- Takemura M, Maoka T, Misawa N. Biosynthetic routes of hydroxylated carotenoids (xanthophylls) in Marchantia polymorpha, and production of novel and rare xanthophylls through pathway engineering in Escherichia coli. Planta. 2015;241(3):699–710.10.1007/s00425-014-2213-0
- Mulet M, Lalucat J. Garcia-Valdés E DNA sequence-based analysis of the Pseudomonas species. Envion Microbiol. 2010;12(6):1513–1530.
- Anzai Y, Kim H, Park J, et al. Phylogenetic affiliation of the pseudomonas based on 16S rRNA sequence. Int J System Evol Microbiol. 2000;50:1563–1589.10.1099/00207713-50-4-1563
- Kajiwara S, Fraser PD, Kondo K, et al. Expression of an exogenous isopentenyl diphosphate isomerase gene enhances isoprenoid biosynthesis in Escherichia coli. Biochem J. 1997;324(2):421–426.10.1042/bj3240421
- Beuttler H, Hoffmann J, Jeske M, et al. Biosynthesis of zeaxanthin in recombinant Pseudomonas putida. Appl Microbiol Biotechnol. 2011;89(4):1137–1147.10.1007/s00253-010-2961-0
- Masamoto K, Misawa N, Kaneko T, et al. Beta-carotene hydroxylase gene from the cyanobacterium Synechocystis sp. PCC6803. Plant Cell Physiol. 1998;39(5):560–564.10.1093/oxfordjournals.pcp.a029405
- Blasco F, Kauffmann I, Schmid RD. CYP175A1 from Thermus thermophilus HB27, the first beta-carotene hydroxylase of the P450 superfamily. Appl Microbiol Biotechnol. 2004;64(5):671–674.10.1007/s00253-003-1529-7
- Sun Z, Gantt E, Cunningham FX Jr. Cloning and functional analysis of the β-carotene hydroxylase of Arabidopsis thaliana. J Biol Chem. 1996;271(40):24349–24352.10.1074/jbc.271.40.24349