Abstract
Green-plant membrane is a phytonutrient present in green leafy vegetables at high concentration. Postprandial increases in blood triglyceride levels result in insulin resistance and type 2 diabetes. Additionally, dietary life and eating order also affect postprandial hypertriglyceridemia. In this study, the effects of once-daily intake of green-plant membrane with dietary oil on postprandial hypertriglyceridemia were investigated in vitro and in vivo. In vitro, green-plant membrane bound hydrophobic bile acids but did not inhibit pancreatic lipase activity. Following the administration, green-plant membrane with dietary oil in rats, oral fat tolerance tests, increases in serum triglycerides levels were significantly reduced. Moreover, fecal total lipid and bile acid volumes were significantly increased in rats that administered 200 mg/mL green-plant membrane. These results suggest that green-plant membrane with dietary oil inhibits dietary fat absorption via promotion of bile acid excretion in feces and the effectiveness of eating green-plant membrane, such as green leafy vegetables, with meals.
Green-plant membrane with meal inhibits dietary fat absorption via excretion of bile acid in feces and the effectiveness of taking such as green leafy vegetables.
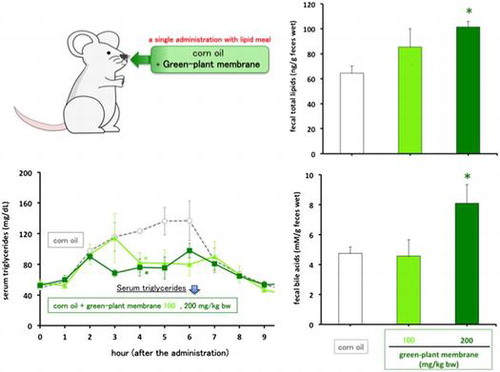
Worldwide, more than 1 billion adults are overweight, with a body mass index (BMI) between 25 and 30 kg/ m2, and more than 300 million adults are obese, with BMI >30 kg/ m2. Obesity increases the risk for type 2 diabetes, cardiovascular disease, and several cancers [Citation1]. Postprandial hypertriglyceridemia also predicts the development of insulin resistance, glucose intolerance, and subsequent type 2 diabetes [Citation2].
Dietary triglycerides are primarily hydrolyzed by pancreatic lipase in the proximal part of the small intestine, giving rise to sn-2 monoacylglycerols and free fatty acids. The products of fat digestion are solubilized by bile acids, which are synthesized in the liver from cholesterol and combined with glycine and taurine to form mixed micelles to absorb dietary fats [Citation3]. Free fatty acids that are absorbed from the intestinal lumen are transported to lymphatic vessels, taken up into the liver, and released as very low-density lipoprotein [Citation4]. Following the emulsification of bile with fat in the small intestine, most bile acids are actively reabsorbed through the terminal ileum into the enterohepatic circulation. Alternatively, bile acids can be trapped by dietary fibers in the large intestine and excreted feces.
Plant-foods, such as fruits and vegetable, play an important role in human diets because they support normal functioning of various body systems [Citation5,6]. They provide our cells with energy, vitamins, minerals, fiber, essential oils, and phytonutrients, also known as phytochemicals. Eating at least five servings of fruits and vegetables per day is recommended to reduce risks for several diseases, including obesity and cardiovascular disease [Citation7]. Specifically, leafy vegetables come from a very wide variety of plants. We are all familiar with lettuce, spinach, and kale; these vegetables with green leaves are popularly used as food. Green leafy vegetables contain a number of different phytochemicals that have a high nutritional value. They are rich sources of antioxidant and are particularly high in vitamins A, C, E, and K. Additionally, many of β-carotene, selenium, omega-3 fatty acids, lutein, and zeaxanthin are also supplied by these vegetables. Spinach extracts have several beneficial effects, such as having anticancer and antiaging properties, increasing nicotinamide adenine dinucleotide, and protecting the central nervous system [Citation8,9]. Recently, a number of studies, both in human subjects and in animals, have reported that thylakoids, isolated from the chloroplast membrane of green leaves, contain proteins, lipids and pigments; increase satiety; and promote weight loss [Citation10–12]. The satiety-promoting effects of thylakoids were explained by their interaction with dietary lipids, prolonging the digestion of dietary fat [Citation13]. Additionally, thylakoids have been found to create a physical barrier on the mucosal surface of the intestine in vitro, causing a decreased uptake of methyl-glucose and macronutrients via the intestinal wall [Citation14]. However, no effects of once-daily intake of thylakoid, green plant membranes, with meal on postprandial hypertriglyceridemia have been shown previously. The oral fat tolerance test (OFTT) is a widely used clinical tool for assessing the risk of several diseases [Citation15]. In the present study, we performed OFTTs and investigated the effects of a single administration with dietary oil, corn oil, and green-plant membrane on postprandial blood triglyceride levels in rats that fed high-fat meals. In addition, we examined bile acid-binding capacities in vitro and determined the effects on fecal parameters, such as bile acids and lipid levels, in vivo.
Materials and methods
Sample materials
The green-plant membranes, particularly thylakoids, used in the present study were obtained from Greenleaf Medical AB (Stockholm, Sweden). This product was prepared from baby spinach leaves using the pH-method, as described by Emek et al. [Citation12] and Montelius et al. [Citation16] followed by drum drying. One hundred grams of green-plant membrane contain 41.7 g carbohydrate (of which 38.7 g was insoluble fiber), 23.5 g protein, and 11.9 g fat.
Bile acid-binding capacity in vitro
Bile acid-binding capacities of green-plant membranes were measured using the method of Matsuda et al. [Citation15] and Eastwood et al. [Citation17] with modifications, and the bile acid-binding ratios of RS to Questran® containing approximately 45% cholestyramine (Sanofi; Tokyo, Japan) were determined. Briefly, green-plant membranes (5, 15, or 30 mg) or cholestyramine (5 mg) was added to each solution of four types of bile acids containing cholic acid, deoxycholic acid, chenodeoxycholic acid, or taurocholic acid at a concentration of 1 mM in 1 mL of 0.1 M phosphate buffer (pH 6.8) and incubated at 37 °C for 1 h in a shaking water bath. Supernatants were then centrifuged at 1750 × g for 10 min, and bile acid contents were measured using an enzymatic assay kit (total bile acid test kit; Wako, Osaka, Japan).
Lipase activity assays in vitro
The inhibition of pancreatic lipase activity by green-plant membranes was measured using Lipase Kit S (the method using a blind test; DS Pharma Biomedical, Osaka, Japan) following the manufacturer’s instructions with modifications. Briefly, 10 μL of 0.1, 0.5, and 1 mg/mL of green-plant membrane in 50% ethanol was added to 190 μL of a coloring solution containing 10 μL of 0.1 mg/mL lipase from porcine pancreas (Wako) and 4 μL of an esterase inhibitor in 0.1 M citric acid buffer. Mixtures were incubated at 30 °C for 5 min and were then reacted with the substrate solution (2,3-dimercaptopropan-1-ol tributyrate, BALB) 20 μL in the dark at 30 °C for 30 min. After the reactions were stopped by the stop solution 400 μL (total volume was 634 μL), lipase activities were measured using a spectrophotometer at 412 nm and were calculated as ratios to the value at 50% ethanol measurement.
Oral fat tolerance tests
Animals were handled in accordance with the guidelines established by the Institutional Animal Care and Use Committee of the Tokyo University of Marine Science and Technology. Four-week-old male Wistar rats were purchased from SLC Japan (Shizuoka, Japan) and assigned to four experimental groups (n = 4) of equal weights (average, 153 g) after feeding on a commercial CE-2 pellet diet (Clea Japan, Tokyo, Japan) for 7 days. Animals were individually housed at 23 ± 2 °C with a 12-h light/12-h dark cycle, and water and food were provided ad libitum.
OFTTs were performed in rats as previously described [Citation15,18]. Briefly, dietary oil samples were prepared with corn oil (Wako) containing 0 (control group), 100, or 200 mg/kg bw green-plant membrane, and these samples were orally administered at 5 mL/kg bw after overnight fasting. Blood samples were collected from the tail veins (50 μL) before and 1–10 h after the administration. Serum triglyceride levels were measured using the TG E-test (Wako). Additionally, fecal samples were collected 16 h before and 5–16 h after the administration.
Fecal total lipids and moisture contents
Fecal pellets from individual rats were pooled and lyophilized. The fecal total lipid contents, such as triglyceride and cholesterol, were measured in sufficient volumes of powdered feces (150 mg) using the gravimetric method described by Folch et al. [Citation19]. Stool moisture contents were measured by the difference between fresh and dry fecal weights.
Fecal bile acid contents
Lyophilized and powdered feces (150 mg) were suspended in 5 volumes of 99.5% ethanol (Wako), incubated at 70 °C for 1 h, and then centrifuged at 3500 × g for 15 min. Bile acid contents were then measured using a total bile acid test kit, as described above.
Statistical analysis
Values were expressed as means ± standard errors (SE) of the mean. Differences between the treatment groups were identified using Dunnett’s multiple comparison tests and were considered significant when *p < 0.05 or **p < 0.01.
Results and discussion
In vitro, green-plant membranes bound several bile acids but did not inhibit pancreatic lipase activity
The percentage of bile acid binding by green-plant membranes is shown in Table . Cholestyramine was used as a positive control and these values calculated as the ratio of cholestyramine to 100%. The results show that 15 and 30 mg/mL green-plant membrane had the highest binding capacity for deoxycholic acid and chenodeoxycholic acid, respectively, with degrees of 47–73%. Choleic acid and taurocholic acid were slightly bound by these concentrations of green-plant membrane, and the binding capacity around 15–34%. Additionally, even at 5 mg/mL green-plant membrane, the binding capacity values for deoxycholic acid, chenodeoxycholic acid, and choleic acid were approximately 15–26%. However, green-plant membrane did not inhibit pancreatic lipase activity, regardless of the concentration (Table ).
Table 1. In vitro bile acid-binding capacity of green-plant membrane at 5, 15, and 30 mg/mL relative to 100% bile acid binding by cholestyramine.
Table 2. In vitro pancreatic lipase inhibition by green-plant membrane at 0.1, 0.5, 1, 5, 15, and 30 mg/mL.
We have previously reported that the binding capacity of 30 mg/mL thylakoid or corn resistant starch for mixtures of bile acids is approximately 50 or 40%, respectively, relative to that of cholestyramine (100%) [Citation15,20]. Additionally, Kahlon and Smith [Citation21] showed that various fruits, such as bananas, peaches, and grapes, had a binding capacity of 5−9% on a dry basis, indicating the potential positive effects of these fruits on health. In the present study, green-plant membranes showed a higher binding capacity, particularly for deoxycholic acid and chenodeoxycholic acid, than the values in our previous reports and in various fruits. Therefore, the present binding capacities indicate the health-protective potential of green-plant membranes.
On the other hand, pancreatic lipase activity was not inhibited by a green-plant membrane dose of 0.1−30 mg/mL (Table ). In this study, 50% ethanol had no effect on the lipase activity (96.23 ± 2.60%). Additionally, we determined lipase activity using substrate suspension, which contained triolein, lecithin, and bile acids. Even in these detections, the ability to inhibit lipase activity was not obtained (data not shown). However, Albertsson et al. [Citation13] showed that chloroplast membranes (thylakoids), isolated from spinach, clover, and Arabidopsis thaliana powerfully suppressed the lipolytic activity in a dose-dependent manner during in vitro hydrolysis of an emulsion of triacylglycerol dispersed in bile salt. Furthermore, they found that one of the major intrinsic membrane proteins of thylakoids, LHC2, which constitutes approximately half of the protein mass of the thylakoid, alone inhibited lipase activity. Additionally, it was shown that three hydrophobic membrane-spanning α-helices that are contained within LHC2 contribute to this mechanism [Citation13,22]. Therefore, we hypothesized that LHC2 protein was degraded because the green-plant membranes used in our research were heat-treated for sterilization in the manufacturing process. As a result of calorimetric measurements of these membranes, calorimetric enthalpy of all transitions was ΣΔHJ 7.10 J/g protein (data not shown), and this value was much lower than Laczko−Dobos’s value, ΣΔHJ 67.79 J/g protein [Citation23]. These results showed that thylakoid may have different functional mechanisms when isolated from fresh plants and after being processed for trade. Several studies have reported the inhibition of lipase activity by food components. Specifically, in studies on the inhibition of lipase activity by phytochemicals, which are present at low concentrations in plant foods, effective concentrations of thylakoid were observed to be in the range of 0.1–10 mg/mL [Citation24,25]. In the present study, the inhibition of lipase activity at 0.1 mg/mL of green-plant membrane was not different.
However, it is possible that thylakoid has binding capacity for deoxycholic acid and chenodeoxycholic acid, which are categorized as hydrophobic bile acids, partly via the LHC remaining after processing.
Intake of green-plant membrane with dietary oil delays fat absorption and increases the fecal excretion of total lipids and bile acids in vivo
The concentration of green-plant membrane administered was adapted following Montelius et al. [Citation16]. and Otokozawa et al. [Citation20]. In agreement with previous work, serum triglyceride levels in rats that were fed only corn oil (control group) showed a clear peak at 4−6 h and returned to their initial level at 7−8 h after administration [Citation18]. Increases in serum triglyceride levels in rats were suppressed by 100 or 200 mg/kg bw green-plant membrane at 4 h after administration of corn oil (Figure ). However, the area under the curve for serum triglycerides was not different in these does groups compared to that in the control group (Figure ).
Figure 1. Fat tolerance in rats administered meals containing 0, 100, or 200 mg/mL green-plant membrane in corn oil. Bar represent means ± SE (n = 4); ○, corn oil (control); ▲, corn oil +100 mg/mL green-plant membrane; ■, corn oil +200 mg/mL green-plant membrane; *p < 0.05 vs. control.
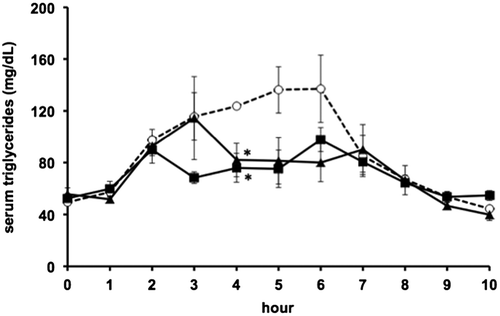
Figure 2. Area under the curve during oral fat tolerance tests. Bars represent means ±SE (n = 4). Open bars, corn oil group (control); black bar, green-plant membrane treatment groups.
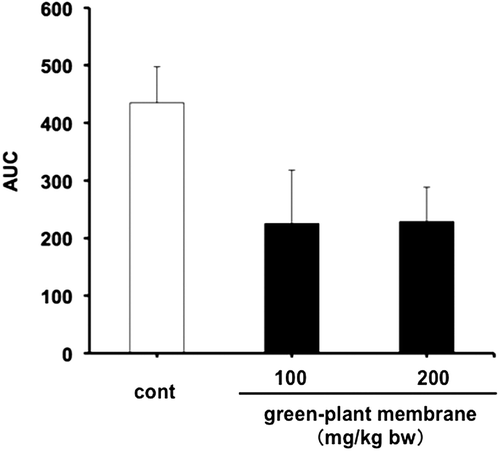
To confirm decreased dietary fat absorption, we measured fecal total lipid contents, such as triglyceride and cholesterol, following oral administration of corn oil containing 100 or 200 mg/kg bw green-plant membrane and showed significant increases in fecal lipid and bile acid levels in the 200 mg/kg bw green-plant membrane group but not in the 100 mg/kg bw green-plant membrane group (Figures and ). Moreover, fecal lipid and bile acid contents were significantly correlated (R = 0.62, p < 0.01; Figure ). On the other hand, thylakoid intake did not affect fecal wet or dry weights and moisture contents (Figure ). These results suggested the lipid absorption reduction effect when the green-plant membrane is low in concentration and suggested lipid absorption suppression effect when the concentration is high. Therefore, we found that the lipid absorption effect differs depending on the concentration of the green-plant membrane. Additionally, it was clearly that a single dietary administration of green-plant membranes inhibits the absorption of dietary fats. This phenomenon was mainly accomplished not by fecal elimination but by promotion of the excretion of bile acids.
Figure 3. Fecal total lipid contents in rats administered meals containing 0, 100, or 200 mg/mL green-plant membrane in corn oil. For the measurement, feces collected from individual rats were pooled and then lyophilized. Bars represent means ±SE (n = 4); Open bars, corn oil group (control); black bar, green-plant membrane treatment groups; *p < 0.05 vs. control.
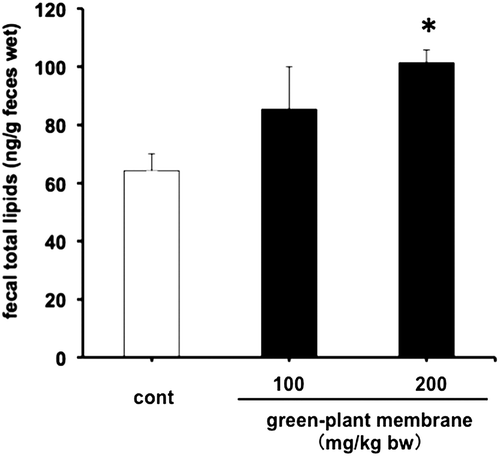
Figure 4. Fecal total bile acid contents in rats administered meals containing 0, 100, or 200 mg/mL green-plant membrane in corn oil. For the measurement, feces collected from individual rats were pooled and then lyophilized. Bars represent means ±SE (n = 4); Open bars, corn oil group (control); black bar, green-plant membrane treatment groups; *p < 0.05 vs. control.
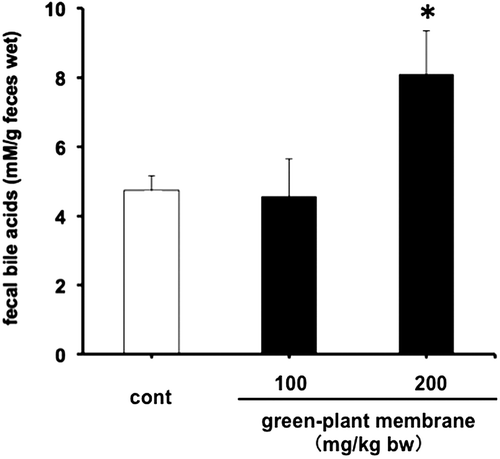
Figure 6. Wet and dry weights and moisture contents in rats administered meals containing 0, 100, or, 200 mg/mL green-plant membrane in corn oil. Feces collected from individual rats were pooled (for wet weight) and then lyophilized (for dry weight). Bars represent means ±SE (n = 4). Open bars, wet weight; black bars, dry weight; gray bars, moisture contents.
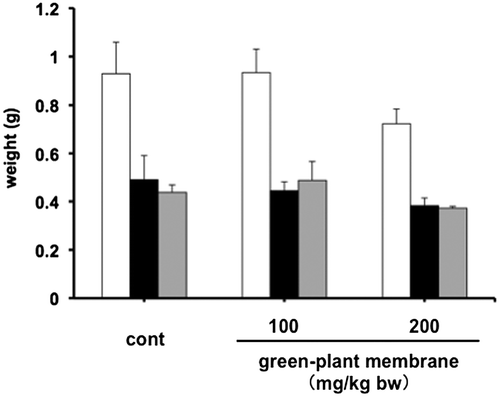
However, Stenblom et al. collected human fecal samples before and after thylakoid administration (5 g Appethyl®) or control dietary- supplementation (50 mL blueberry drink) every morning for 12 weeks and showed that thylakoid-supplementation has no influence on the total intestinal absorption of dietary lipids [Citation26]. In the present study, we applied a single dietary administration of green-plant membrane with dietary fat. Moreover, we have previously suggested that an intake of these membranes just before eating a lipid meal induces a suppressive effect on postprandial hypertriglyceridemia [Citation20]. Therefore, the results may differ depending on the time of administration (together with or just before meals) or separate limited administration, such as intake only in the morning. Since corn oil is administered as fat in this study, these effects may change when ingested at the same time with the actual high-fat diet and green-plant membrane.
In conclusion, our results presented provide evidence for the fats absorption inhibitory effects of once-daily intakes of green-plant membrane with dietary oil in rat. Specifically, fat absorption from dietary oil was decreased and fecal loss of bile acids was increased after supplementation with moderate green-plant membrane concentrations. Taking green-plant membrane with every meal provided the body with the lipid excretory function of these membranes and might further improved the effects of obesity management. Taken with previous studies on long-term green-plant membrane intake in mammals, including humans, these data confirm the health-promoting potential of once-daily intake of green-plant membrane with meals, by eating green leafy vegetables.
Author contributions
Hiroko Matsuda and Takaaki Shirai designed the experiments, analyzed the data, and wrote the manuscript. Shinpei Ooi, Ryo Otokozawa, Kodai Kumazaki, Eri Udagawa, Masaya Asakura, and Daisuke Suzuki perfumed the experiments and analyzed the data.
Disclosure statement
No potential conflict of interest was reported by the authors.
Funding
This work was supported by KENKO Mayonnaise Co., Ltd.
Acknowledgements
All the authors have reviewed the paper and approved the final version.
References
- Hill JO, Wyatt HR, Reed GW, et al. Obesity and the environment: where do we go from here? Science. 2003;299:853–855.10.1126/science.1079857
- Aslam M, Aggarwal S, Sharma KK, et al. Postprandial hypertriglyceridemia predicts development of insulin resistance glucose intolerance and type 2 diabetes. PLoS ONE. 2016;11:e0145730.10.1371/journal.pone.0145730
- Bernbäck S, Bläckberg L, Hernell O. The complete digestion of human milk triacylglycerol in vitro requires gastric lipase, pancreatic colipase-dependent lipase, and bile salt-stimulated lipase. J Clin Invest. 1990;85:1221–1226.10.1172/JCI114556
- Iqbal J, Hussain MM. Intestinal lipid absorption. Am J Physiol Endocrinol Metab. 2009;296:E1183–1194.10.1152/ajpendo.90899.2008
- Ramesa SB, Sooad AD. Phytochemical constituents and antibacterial activity of some green leafy vegetables. Asian Pac J Trop Biomed. 2014;4:189–193.
- Liu RH. Health benefits of fruit and vegetables are from additive and synergistic combinations of phytochemicals. Am J Clin Nutr. 2003;78:517S–520S.
- Krauss RM, Eckel RH, Howard B, et al. AHA dietary guidelines : revision 2000: a statement for healthcare professionals from the nutrition committee of the American heart association. Circulation. 2000;102:2284–2299.10.1161/01.CIR.102.18.2284
- Matsuda H, Gomi RT, Hirai S, et al. Effect of dietary phytol on the expression of α-amino-β-carboxymuconate-ε-semialdehyde decarboxylase, a key enzyme of tryptophan-niacin metabolism, in rats. Biosci Biotechnol Biochem. 2013;77:1416–1419.10.1271/bbb.130029
- Lomnitski L, Bergman M, Nyska A, et al. Composition, efficacy, and safety of spinach extracts. Nutr Cancer. 2003;46:222–231.10.1207/S15327914NC4602_16
- Köhnke R, Lindbo A, Larsson T, et al. Thylakoids promote release of the satiety hormone cholecystokinin while reducing insulin in healthy humans. Scand J Gastroenterol. 2009;44:712–719.10.1080/00365520902803499
- Köhnke R, Lindqvist A, Göransson N, et al. Thylakoids suppress appetite by increasing cholecystokinin resulting in lower food intake and body weight in high-fat fed mice. Phytother Res. 2009;23:1778–1783.10.1002/ptr.v23:12
- Emek SC, Szilagyi A, Akerlund HE, et al. A large scale method for preparation of plant thylakoids for use in body weight regulation. Prep Biochem Biotechnol. 2010;40:13–27.
- Albertsson PA, Köhnke R, Emek SC, et al. Chloroplast membranes retard fat digestion and induce satiety: effect of biological membranes on pancreatic lipase/co-lipase. Biochem J. 2007;401:727–733.10.1042/BJ20061463
- Montelius C, Gustafsson K, Weström B, et al. Chloroplast thylakoids reduce glucose uptake and decrease intestinal macromolecular permeability. Br J Nutr. 2011;106:836–844.10.1017/S0007114511001267
- Matsuda H, Kumazaki K, Otokozawa R, et al. Resistant starch suppresses postprandial hypertriglyceridemia in rats. Food Res Nutr. 2016;89:838–842.
- Montelius C, Szwiec K, Kardas M, et al. Dietary thylakoids suppress blood glucose and modulate appetite-regulating hormones in pigs exposed to oral glucose tolerance test. Clin Nutr. 2014;33:1122–1126.10.1016/j.clnu.2013.12.009
- Eastwood MA, Anderson R, Mitchell WD, et al. A method to measure the adsorption of bile salts to vegetable fiber of differing water holding capacity. J Nutr. 1976;106:1429–1432.
- Egashira Y, Kamohara T, Yamaguchi W, et al. Suppression of postprandial hypertriglyceridemia in rats by benifuuki tea extract. Nippon Shokuhin Kagaku Kougaku Kaishi. 2013;60:407–411.10.3136/nskkk.60.407
- Folch J, Lees M, Sloane Stanley GH. A simple method for the isolation and purification of total lipides from animal tissues. J Biol Chem. 1957;226:497–509.
- Otokozawa R, Matsuda H, Kumazaki K, et al. Effects of dietary thylakoid intake just before diet on postprandial serum hypertriglyceridemia in rats. J Integr Stud Diet Habits. 2016;27:237–242.
- Kalhon TS, Smith GE. In vitro binding of bile acids by bananas, peaches, pineapple, grapes, pears, apricots and nectarines. Food Chem. 2007;101:1046–1051.
- Liu Z, Yan H, Wang K, et al. Crystal structure of spinach major light-harvesting complex at 2.72 A resolution. Nature. 2004;428:287–292.10.1038/nature02373
- Laczkó-Dobos H, Todinova SJ, Sözer Ö, et al. Identification of thylakoid membrane thermal transitions in Synechocystis sp. PCC6803 photosynthetic mutants. Photosynth Res. 2011;107:237–246.10.1007/s11120-011-9627-3
- Boath AS, Grussu D, Stewart D, et al. Berry polyphenols inhibit digestive enzymes: a source of potential health benefits? Food Dig. 2012;3:1–7.10.1007/s13228-012-0022-0
- Han LK, Kimura Y, Kawashima M, et al. Anti-obesity effects in rodents of dietary teasaponin, a lipase inhibitor. Int J Obes Relat Metab Disord. 2001;25:1459–1464.10.1038/sj.ijo.0801747
- Stenblom EL, Weström B, Linninge C, et al. Dietary green-plant thylakoids decrease gastric emptying and gut transit, promote changes in the gut microbial , but does not cause steatorrhea. Nutr Metab (Lond). 2016;13:1–9.10.1186/s12986-016-0128-4