Abstract
The reduction pathway leading to the formation of dolichol was clarified in 2010 with the identification of SRD5A3, which is the polyprenol reductase. The finding inspired us to reanalyze the length of the major chain of polyprenol and dolichol from several plant leaves, including mangrove plants, as well as from animal and fish livers by 2D-TLC. Polyprenol- and dolichol-derived metabolites such as polyprenylacetone and epoxydolichol were found together with rubber-like prenol. This review focuses on analyses of polyprenol and its derivatives, including recently found epoxypolyprenol and polyprenylacetone. Attention has also been paid to the chromatographic behavior of rubber-like prenol on TLC.
This review focuses on the history and recent advances in research of polyprenol and its derivatives, including rubber-like prenol, epoxypolyprenol, epoxydolichol, and polyprenylacetone.
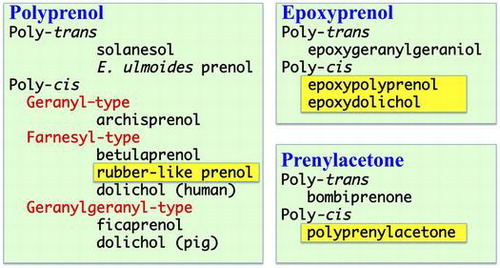
A naturally occurring isoprenoid alcohol with a long carbon chain length (dolichol) was found in human tissues in 1960 [Citation1], and its function has been elucidated as being a sugar carrier lipid during the biosynthesis of glycoproteins [Citation2]. Since then, a number of studies on its biosynthesis, distribution, and function have been carried out, the details of which were reviewed by Chojnacki and Dallner [Citation3] in 1988. At that time, however, the reduction pathway to form dolichol from polyprenol was still unclear. Members of the polyprenol family can be separated using reversed-phase chromatography, and it is possible to analyze the differences between the polyprenol and dolichol families by reversed-phase HPLC. The difference of the retention time between each family member is really small - they are eluted as partially overlapping doublet. Careful modification of the gradient results in an acceptable separation. Polyprenols (dehydrodolichols) are more abundant in plants than in animals, and their distribution in plants summarized by Swiezewska and Chojnacki in 1994 [Citation4]. In addition to reversed-phase HPLC, two-dimensional (normal-phase and reversed-phase) thin-layer chromatography (2D-TLC) has also been used to analyze various dolichols and polyprenols [Citation5]. The 2D-TLC method is superior to reversed-phase HPLC in that the polyprenols are completely separated from the dolichols: however, the sensitivity is lower than that of HPLC.
The reduction pathway leading to the formation of dolichol was clarified in 2010 with the identification of SRD5A3, which is the polyprenol reductase [Citation6–8]. The analysis of polyprenol and dolichol was done by using reversed-phase HPLC. These findings inspired us to reanalyze the length of the major chain of polyprenol and dolichol from several plant leaves, including perilla by 2D-TLC [Citation9]. When we used larger amounts of unsaponifiable lipids from perilla leaves to clearly visualize the separation between the polyprenol and dolichol, 2D-TLC revealed the occurrence of two families with chains longer than C80 (16 isoprene units) along two oblique lines, as shown in Figure . This surprising finding led us to further analyze the unsaponifiable lipids of various plant leaves, including mangrove plants as well as the unsaponifiable lipids of animal and fish livers by 2D-TLC [Citation10–13].
Figure 1. 2D-TLC of poly-cis prenol and dolichol from perilla leaves. Upper panel: the first, toluene:ethylacetate (4:1); the second, acetone. The acetone development was repeated 10 times. Lower panel: the first, toluene:ethylacetate (49:1); the second, acetone:methylethylketone (1:1). Rubber-like prenol is indicated with an arrow. Poly-cis, poly-cis prenol; Dol, dolichol. 16 = number of isoprene units. Ori., origin; S.F., solvent front (Sagami unpublished data).
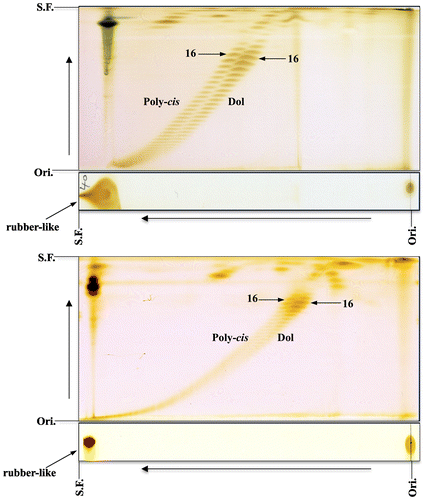
More recently, two kinds of polyprenol- and dolichol-derived metabolites were found by 2D-TLC. One is epoxydolichol [Citation13], which is found in fish liver, and the other is polyprenylacetone [Citation12], which is found in the leaves of mangrove plants. It is not clear at present if these two of metabolites, or any of the polyprenols that can be detected in plants, play important roles inside or outside of cells. The structure, biosynthesis, and function of polyisoprenoids and their cellular significance have recently been reviewed [Citation14–16], and recent studies on isoprenoid generating systems (MVA and MEP pathways) in plants have been summarized [Citation17–19]. Therefore, this review focuses on analyses of polyprenol and its derivatives, including epoxypolyprenol and polyprenylacetone, recently carried out using 2D-TLC. Attention has also been paid to the chromatographic behavior of rubber-like prenol on TLC. Their chemical structures are shown in Figure .
Materials and methods
Teprenone (a 3:2 mixture of geranylgeranyl acetone and geranylneryl acetone, Eizai Co.) was obtained from a local drug store. Polyprenols (C85–95) from Ginkgo biloba were provided by Kuraray Co. Polyprenols (C55–60) from silkworm feces were provided by Takasago Perfumery Co. Dry leaves Eucommia ulmoides leaves (tochu in Japanese) were purchased in a local herbal medicine shop. Leaves of perilla (Perilla frutescens) and apple mint (Mentha suaveolens) were obtained from a local super market and a campus field at Tohoku University, respectively. Lavender (Lavandula angustifolia) and laurel (Laurus nobilis) were purchased from a garden shop. Luminitzera racemosa leaves were obtained in Okinawa Prefecture, Japan.
Dry leaves of basil (Ocimum basilicum), rosemary (Rosmarinus officinalis), oregano (Origanum vulgare), winter savory (Satureja montana), marjoram (Origanum majorana), thyme (Thymus vulgaris), peppermint (Mentha x piperita), and common sage (Salvia officinalis); roots of dandelion (Taraxacum officinale) and echinacea (Echinacea purpurea); stems (leaves) of artichoke (Cynara scolymus); and roots (stems) of kutki (Picrorhiza kurroa) were used from collections of Prof. Yoshihiro Shidoji of University of Nagasaki. Livers of Pacific bluefin tuna (Thunnus orientalis, kuromaguro in Japanese) were a generous gift from the Aquaculture Research Institute of Kinki University with the help of Prof. Shigeru Shigeoka of Kinki University. Skipjack tuna liver was obtained from a local fish shop. Mass spectra of bombiprenone (potato leaves) and rubber-like compounds (apple mint leaves) were acquired at Tohoku University, at the Institute of Organic Chemistry PAS, and at the Institute of Biochemistry and Biophysics PAS.
Analysis of unsaponifiable lipids
Plant leaves were dried at 65 °C overnight and then extracted with chloroform:methanol (2:1). The extracts (less than 1.0 g) were saponified in KOH (0.45 g), water (2.0 mL), and ethanol (2.0 mL) at 65 °C 3 h ~ overnight and the saponified mixture was extracted with hexane (4.0 mL). In the case of fish livers [Citation13], Pacific bluefin tuna and skipjack tuna livers (8.51 g and 8.57 g, respectively) were saponified in KOH (1.125 g), water (5.0 mL), ethanol (5.0 mL), and pyrogallol (0.05 g) at 65 °C overnight and the saponified mixture was extracted with hexane (10 mL). In the case of both the plant and fish extracts, the hexane extracts were analyzed by two-dimensional thin-layer chromatography (2D-TLC) [Citation5]. A prenyl sample (between 0.1 and 1 μg) is required for detection with iodine vapor. The first silica-gel TLC was carried out with toluene:ethylacetate (4:1, 9:1, 19:1, or 49:1), and the transfer of polyprenols and dolichols to the second RP-C18 silica-gel plate was done with acetone or acetone:methylethylketone (1:1), followed by the second round of chromatography using acetone or acetone:methylethylketone (1:1). To reuse the reversed-phase plates, it is necessary to completely remove the iodine from the plate in the hood and develope the plate once with dichloromethane or chloroform.
Large quantities of rubber-like prenol of apple mint leaves for structural characterization were prepared using 865 g of leaves (wet weight). The dried leaves (178 g) were extracted with chloroform:methanol (2:1) (900 mL), and the extracts (7.1 g) were saponified in KOH (4.5 g), water (20 mL), and ethanol (20 mL) overnight at 65 °C and then the mixture was extracted with hexane (40 mL). The crude extracts (ca. 600 mg) was mixed with silica gel powder (ca. 6 g) and applied to a silica-gel column (4.7 × 12 cm) equilibrated with acetone. After acetone elution (3 mL, 37 frs.), the rubber-like compounds were eluted with chloroform. Fractions (55–73) were combined and dried, followed by analysis by NMR.
Polyprenol
Polyprenol is the generic name for 1,4-polyisoprene alcohols, and structurally classified into main two groups, poly-trans and poly-cis prenols, as shown in Figure .
Poly-trans prenol
Geraniol (C10), farnesol (C15), and geranylgeraniol (C20), which are acyclic mono, sesqui, and diterpene alcohols, respectively, are known as essential oils, and are examples of poly-trans prenol. Phytol (C20), another trans-prenol, is a side chain of the chlorophyll molecule. A triene, 12,13-dehydrogeranylgeraniol was isolated from the aquatic plant Saururus cernuus [Citation20]. Solanesol (C45), which was isolated from flue-cured tobacco leaves (Nicotiana tabacum) in 1956 [Citation21], is a typical poly-trans prenol, and its structure is shown in Figure . Recently, poly-trans prenol with chain lengths longer than C65 has been found in tissues of E. ulmoides [Citation22,23]. The trans isomers were found in the leaves, seed coats, and roots but not in the bark and seeds. The poly-trans prenol, poly-cis prenol, and dolichol in E. ulmoides were clearly separated by family using capillary column chromatography. 2D-TLC was also used to separate those polyisoprenoids into three groups along oblique lines consisting of each family as shown in Figure (upper). The poly-cis prenol and dolichol of perilla leaves are shown in Figure (lower). When the poly-cis prenol, dolichol, and poly-trans prenol of E. ulmoides were mixed with the poly-cis prenol and dolichol of perilla, the members of the three isoprenoid families were actually separated by family as shown in Figure (middle). It should be noted that compounds indicated with an arrow remained near the top (with the solvent front) on the first silica gel thin-layer plate.
Figure 3. 2D-TLC of polyisoprenoid from the leaves of E. ulmoides and perilla. Upper panel, E. ulmoides; middle panel, a mixture of E. ulmoides and perilla; lower panel, perilla. The first, toluene:ethylacetate (19:1); the second, acetone (once). Poly-cis, poly-cis prenol; Poly-trans, poly-trans prenol; Dol, dolichol. 16 = number of isoprene units. Rubber-like prenol is indicated with an arrow. Ori., origin; S.F., solvent front (Sagami unpublished data).
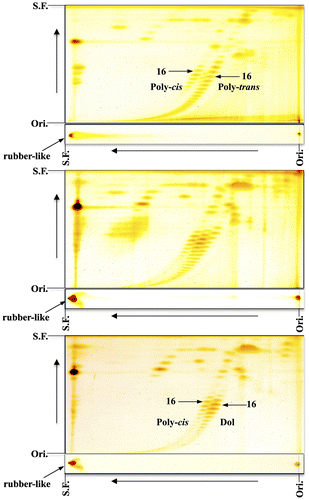
Poly-cis prenol
The family of poly-cis prenol is classified into three groups based on stereochemistry as shown in Figure . Geranyl-type prenol (mono-trans poly-cis) include arachisprenol of Arachis hypogaea [Citation24]. Farnesyl-type prenol (di-trans poly-cis) include betulaprenol of Betula verrucosa [Citation25,26], rubber-like poly-cis prenol of L. racemosa [Citation10], and undecaprenol of Lactobacillus plantarum [Citation27]. Geranygeranyl-type prenol (tri-trans poly-cis) include ficaprenol from Ficus elastica [Citation28], malloprenol from Mallotus japonicus [Citation29], moraprenol from Morus bombycis K. (mulberry) [Citation30,31], castaprenol from Aesculus hippocastanum (horse chestnut) [Citation32], and cleomeprenol from Cleome spinosa [Citation33]. Dolichol corresponds to poly-cis prenol with an α-saturated isoprene. Human C90–105 dolichol has a farnesyl-type structure, whereas pig liver C55 dolichol has a geranylgeranyl-type structure.
Acyclic poly-cis prenol (C25), with one cis-isoprene unit attached to phytyl group, was isolated from potato (Solanum tuberosum) leaves in 1969 [Citation34]. Poly-cis prenol (glycinoprenol C45, C50, and C55) from the leaves of soy bean (Glycine max Merill) possesses a phytyl residue, 4 to 6 internal cis-isoprene residues, and a cis α-terminal residue aligned in that order [Citation35].
So far, the polyprenols with the longest chains (up to C500) have been found in the leaves of a mangrove plant (L. racemosa) and were as rubber-like polyisoprenoid alcohols [Citation10]. Figure (upper) shows the 2D-TLC of the unsaponifiable lipids of L. racemosa. Two families separated along oblique lines corresponding to the poly-cis prenol and dolichol were observable on the second RP-C18 silica gel thin-layer plate, and, as expected, a strong spot corresponding to rubber-like prenol was detected on the first silica gel thin-layer plate. In the 2D-TLC of the unsaponifiable lipids of perilla leaves, there were compounds that remained on the first silica gel plate, as indicated with an arrow in Figure , implying the presence of rubber-like prenol in perilla. This is also the case for unsaponifiable lipids of apple mint shown in Figure (lower) and lavender leaves (figure not shown). The rubber-like prenol has also been detected in unsaponifiable lipids of dry leaves (basil, rosemary, oregano, winter savory, marjoram, thyme, peppermint, and common sage), roots (dandelion and echinacea), stems (artichoke), and roots (kutki) (Sagami and Shidoji unpublished data). To confirm the presence of rubber-like prenol, the lipids of apple mint leaves were purified by silica gel chromatography, and the purified compounds were analyzed by NMR spectroscopy. As expected, rubber-like signals (cis-isoprene) were detected although the intensities were low (Sagami and Swiezewska unpublished data).
Figure 4. 2D-TLC of rubber-like prenol from the leaves of L. racemosa and apple mint. Upper panel, L. racemosa; lower panel, applemint. The first, toluene:ethylacetate (4:1); the second, acetone (once). Poly-cis, poly-cis prenol; Dol, dolichol. 11, 16 = number of isoprene units. Bp C43, bombiprenone C43; Sol C45, solanesol. Rubber-like prenol is indicated with an arrow. Ori., origin; S.F., solvent front (Sagami unpublished data).
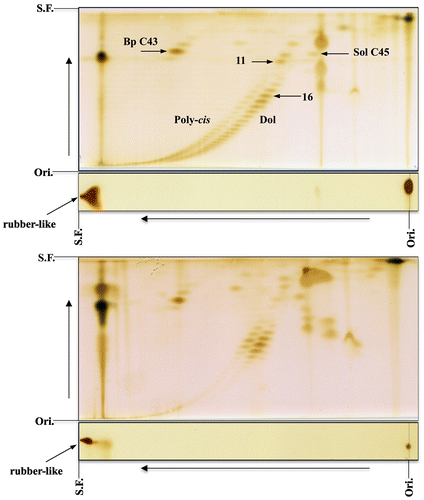
Two cis-prenyltransferase genes (RER2 and SRT1) have been found in yeast [Citation36–39].
The products of these two genes are responsible for the syntheses of the carbon-backbones of the C70–90 and C95–120 dolichols, respectively. During an analysis of the endogenous lipids of a mutant yeast (#64), a shorter-chain (C55–60) polyprenol was found along with a C70–80 dolichol by 2D-TLC [Citation40], though the stereochemistry of the shorter polyprenol remains unclear. Preliminary characterization of a C55 polyprenol detected at the stationary phase of growth of some yeast strains indicated the presence of internal two trans-isoprene residues [Citation41]. Pig liver also contains a family of shorter-chain polyprenols dominated by a C55-dolichol and a long chain (C95–100) dolichol [Citation42]. The number of internal trans isoprene residues of the former compounds was consistent with a geranylgeranyl-type dolichol. It might be that three polyprenol families (one shorter and two longer) occur in yeast and two polyprenol families (one shorter and one longer) occur in pig.
Epoxyprenol
The general chemical structure of epoxyprenol is shown in Figure . The epoxide is located on the ω-isoprene residue in poly-cis and poly-trans prenols.
Epoxy poly-trans prenol
All-trans 14,15-epoxygeranylgeraniol was identified as an active substance in the oil of the fruits from Pterodon pubescens Benth [Citation43]. It shows prophylactic activity against infections from cercariae of Schistosoma mansoni; however, all-trans geranylgeraniol is inactive. Later, the natural product was established to have the S configuration [Citation44].
Epoxy poly-cis prenol
Epoxydolichol with minor epoxypolyprenol was found by chance during an analysis of unsaponifiable lipids in fish livers. In the case of skipjack tuna liver a new family of compounds was detected on the second RP-C18 plate [Citation13]. The new family of compounds migrated faster than dolichoic acid (C95–100) and slower than endogenous dolichol in the normal-phase direction, implying that they are more polar than the alcohol. The NMR and mass analyses strongly supported the presence of epoxy functionality on the ω-isoprene residue. The epoxydolichol family was similarly detected in Pacific bluefin tuna (kuromaguro in Japanese) liver, as shown in Figure (upper), though the content was lower than that of skipjack tuna liver in Figure (lower).
Figure 5. 2D-TLC of epoxypolyprenol from livers of Pacific bluefin tuna (upper) and skipjack tuna (lower). The first, toluene:ethylacetate (9:1); the second, acetone (once). Dol, dolichol; Epoxy-dol, epoxydolichol. Ori., origin; S.F., solvent front (Sagami and Shidoji unpublished data).
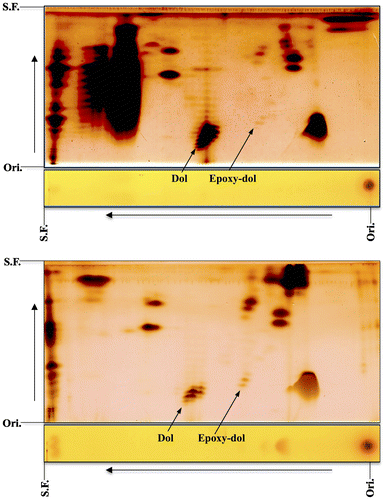
Ishinaga and Ueda reported the occurrence of novel dolichyl derivatives in rat spleen [Citation45]. The compounds were not dolichyl dolichoate [Citation46], which is present in bovine thyroid. They also described that these compounds were not found in young rats (4 months of age) but were found in old rats (approximately 12 months of age). The compounds might correspond to epoxydolichyl derivatives. Ward et al. recently reported the occurrence of a series of natural dolichol (Dol) and oxidized isoprenoid lipids in neuromelanins in various regions of the human brain by LC/MS analysis [Citation47]. The oxidized isoprenoid lipids include Dol (+16), Dol (+32), Dol (+48), Dol (+64), and dolichoic acid (+16), reflecting addition of oxygen (s) along the isoprenoid chain. Dol (+16) might be epoxydolichol.
Epoxydolichol has a farnesyl residue on the ω-isoprene group; this is also the case for epoxy squalene, which is a precursor for the formation of lanosterol. In this respect, it may be important to note whether the chirality at the third carbon from the ω terminal is the same as that of epoxy squalene (which is S) [Citation48]. In comparison, the chirality of epoxy poly-trans C20 prenol is S and that of the juvenile hormone (epoxy poly-trans C15 prenoic acid) was determined to be R [Citation40,49].
Hevea rubber has been reported to contain an epoxide group, and the concentration is estimated to be one epoxide group per approximately 190–320 isoprene units in Hevea rubber from fresh latex or a variety of its clones [Citation50,51]. It is uncertain if the epoxide is located on an internal isoprene unit or on the ω-isoprene unit. The discovery of the epoxydolichol (epoxypolyprenol) in fish liver suggests epoxypolyprenol (epoxy dolichol) and epoxy-rubber-like prenol may also be present in plants, especially on the ω-isoprene group. Since Tanaka presumed that the biosynthesis of natural rubber starts with an unidentified initiating species containing two trans-isoprene units and a peptide group [Citation52], it is possible to assume that one intermediate in the biosynthetic pathway of rubber might be an epoxy compound of a rubber-like polyprenol.
Prenylacetone
The general chemical structure of prenylacetone is shown in Figure . Polyprenyl derivatives are also found as poly-trans and poly-cis compounds.
Poly-trans prenylacetone
Shorter carbon-chain prenylacetone such as geranyl acetone (C13) or farnesyl acetone (C18) was found as a volatile compound in fruits such as tomatoes and watermelons [Citation53]. A 43-carbon ketone isolated from the unsaponifiable matter of silkworm (Bombyx mori L.) feces was identified as a polyisoprenoid compound (bombiprenone, C43) with 8 isoprene units with each unit having one trans double bond to which an acetonyl residue was attached at the terminal unit [Citation54]. The ketone was also found in the leaves of mulberry trees (M. bombycis K.) [Citation55], which comprises the entire diet of silkworm.
Apart from their natural occurrence, poly-trans prenylacetones (C18, C23, C28, C33, C43, and C48) have been chemically synthesized, and the antiulcer effects of those acyclic acetones on cold-restraint stress induced gastric ulcers in rats were reported [Citation56]. Geranylgeranyl acetone mixed with geranylneryl acetone showed a particularly potent antiulcer effect. A 3:2 mixture of geranylgeranyl and geranylneryl acetones (general name, teprenone) is, at present, known as an antiulcer pharmaceutical drug.
Through feeding experiments using protoplast of Botryococcus braunii B race strain, Inoue et al. identified farnesal and 3-hydroxy-2,3-dihydrofarnesal as metabolites from farnesol [Citation57]. The authors described the possibility that 3-hydroxy-2,3-dihydrofarnesal is readily decomposed into geranyl acetone and acetaldehyde. By analogy, solanesol (C45) (see the section of poly-trans prenol) may be converted to solanesal and then 3-hydroxy-2,3-dihydrosolanesal, which might be decomposed into bombiprenone (C43) and acetaldehyde (C2).
Poly-cis prenylacetone
Although bombiprenone (C43) is often detectable by 2D-TLC in the leaves of many plants, an isoprenoid family is sometimes found near a bombiprenone family. Figure (upper) shows the case of unsaponifiable lipids of laurel leaves. Figure (lower) shows the case of the laurel sample mixed with teprenone (a mixture of geranylgeranyl acetone and geranylneryl acetone). As expected, geranylneryl acetone ran faster than geranylgeranyl acetone. The right oblique spots directly below the geranylgeranyl acetone spot correspond to poly-trans prenylacetone (C28, C33, C38, C43 and C48) with the main spot being bombiprenone (C43). It is believed that the left oblique spots directly below the geranylneryl acetone spot correspond to poly-cis prenylacetone (C28, C33, C38, C43, C48, C53, and C58) with the main being C48 and C53. Since strong spots corresponding to ficaprenol (C50 and C55) are observed on the RP-C18 plate, their derivatives might be prenylacetone (C48 and C53). These poly-cis prenylacetones as well as poly-trans prenylacetone (bombiprenone) were detected in Allium tuberosum (nira in Japanese), Boehmeria nivea var. nipononivea (karamushi in Japanese), Laurus nobilis (gekkeiju in Japanese), and Euphorbia supina (konishikisou in Japanese) (Sagami unpublished data). More recently, Basyuni et al. analyzed the unsaponifiable lipids of North Sumatran mangrove leaves by 2D-TLC and found poly-cis prenylacetones ranging from C23 to C88 in Sonneratia caseolaris and Xylocarpus granatum and ranging from C23 to C108 in Aegiceras corniculatum [Citation12].
Figure 6. 2D-TLC of poly-cis and poly-trans acetones from laurel leaves. Upper panel, unsaponifiable lipids; lower panel, unsaponifiable lipids plus teprenone. The first, toluene:ethylacetate (9:1); the second, acetone (once). GN acetone, geranylneryl acetone; GG acetone, geranylgeranyl acetone. Sol C45, solanesol; Fica C55, ficaprenol C55; Bp C43, bombiprenone C43. Ori., origin; S.F., solvent front (Sagami unpublished data).
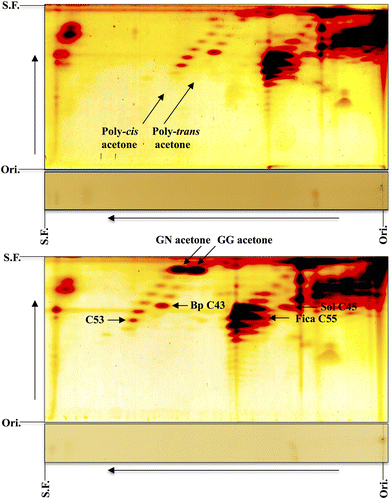
Polyisoprene [Citation58,59], both the cis-isomer [poly (cis-1,4-isoprene)] and the trans-isomer [poly (trans-1,4-isoprene)], are synthesized by more than 2500 plants, such as E. ulmoides, and some fungi. Although their biosynthetic pathways have not yet been established, their bacterial degradation pathways have recently been clarified. In the initial O2-dependent oxidation process shortened rubber oligomers with aldehyde and keto groups at their termini are generated after the oxidative cleavage of the double bonds of poly (cis-1,4-isoprene) and poly (trans-1,4-isoprene), and the aldehyde groups that are unstable toward oxidation should be oxidized to the corresponding acids outside of the bacterial cells. Since the keto moieties correspond to acetone groups, the initial microbial oxidative process may give us a clue toward understanding the formation of polyprenyl acetone from polyprenol in plants.
Perspectives
Analysis of polyprenyl compounds by 2D-TLC has allowed the identification of several new metabolites leading to the following new insights.
(1) | Rubber-like prenol may occur widely in the plant kingdom. It is possible to easily detect rubber-like prenol on a thin-layer silica gel plate (5 × 20 cm). The plate is firstly developed (up to 15 cm) with toluene:ethylacetate (9:1) and then developed (up to 2 cm) with acetone toward the second dimensional direction, followed by exposure of the plate to iodine vapor. Compounds that migrate up to the solvent front with toluene:ethylacetate (9:1) and that remain on the solvent front after development with acetone are expected to be rubber-like prenol. | ||||
(2) | The finding of epoxydolichol in fish livers suggests dolichyl proteins, which are presumably different from dolichyl-phospho proteins and dolichylthio proteins [Citation60,61], may also be present because the epoxy structure is easily associated with an amino group of peptides or proteins to form dolichyl- and polyprenyl proteins. Regarding polyprenyl acetones, more information is necessary to learn whether they are intermediates in a catabolic pathway and whether they are also found in both the animal kingdom and the plant kingdom. |
Disclosure statement
No potential conflict of interest was reported by the authors.
Acknowledgements
The authors thank Hiroyuki Momma of Tohoku University, Jacek Wojcik of Institute of Biochemistry and Biophysics, PAS, and Widold Danikiwicz of Organic Chemistry PAS for measurement of lipids by mass and NMR spectroscopy. The authors are also grateful to Adam Jozwiak and Katarzyna Gawarecka of Polish Academy of Sciences for their experimental support, to Prof. Hironori Oku of Ryukyu University Japan for collecting the mangrove plant samples, to Takeshi Nakamura and Prof. Norimasa Ohya of Yamagata University Japan, Prof. Masahiko Nagaki of Hirosaki University Japan for helpful discussions, and to Prof. Mohammad Basyuni of University of Sumatera Utera Indonesia for valuable discussion.
References
- Pennock JF, Hemming FW, Morton RA. Dolichol : a naturally occurring isoprenoid alcohol. Nature. 1960;186:470–472.10.1038/186470b0
- Behrens NH, Leloir LF. Dolichol monophosphate glucose: an intermediate in glucose transfer in liver. Proc Natl Acad Sci USA. 1970;66(1):153–159.10.1073/pnas.66.1.153
- Chojnacki T, Dallner G. The biological role of dolichol. Biochem J. 1988;251:1–9.10.1042/bj2510001
- Swiezewska E, Sasak W, Mankowski T, et al. The search for plant polyprenols. Acta Biochim Polon. 1994;41(3):221–260.
- Sagami H, Kurisaki A, Ogura K, et al. Separation of dolichol from dehydrodolichol by a simple two-plate thin-layer chromatography. J Lipid Res. 1992;33(12):1857–1862.
- Sagami H, Kurisaki A, Ogura K. Formation of dolichol from dehydrodolichol is catalyzed by NADPH-dependent reductase localized in microsomes of rat liver. J Biol Chem. 1993;268(14):10109–10113.
- Cantagrel V, Lefeber D, Ng BG, et al. SRD5A3 is required for converting polyprenol to dolichol and is mutated in a congenital glycosylation disorder. Cell. 2010;142:203–217.10.1016/j.cell.2010.06.001
- Jozwiak A, Gutkowska M, Gawarecka K, et al. Polyprenol REDUCTASE2 deficiency is lethal in arabidopsis due to male sterility. Plant Cell. 2015;27:3336–3353.
- Kurisaki A, Sagami H, Ogura K. Distribution of polyprenols and dolichols in soybean plant. Phytochemistry. 1997;44(1):45–50.10.1016/S0031-9422(96)00400-1
- Skoczylas E, Swiezewska E, Chojnacki T, et al. Long-chain rubber-like polyisoprenoid alcohols in leaves of Lumnitzera racemosa. Plant Physiol Biochem. 1994;32(6):825–829.
- Basyuni M, Sagami H, Baba S, et al. Diversity of polyisoprenoids in ten Okinawan mangroves. Dendrobiology. 2016;75:167–175.10.12657/denbio.075.016
- Basyuni M, Sagami H, Baba S, et al. Distribution, occurrence, and cluster analysis of new polyprenyl acetones and other polyisoprenoids from North Sumatran mangroves. Dendrobiology. 2017;78:18–31.10.12657/denbio.078.003
- Ishiguro T, Morita-Fujimura Y, Shidoji Y, et al. Dolichol biosynthesis: the occurrence of epoxy dolichol in skipjack tuna liver. Biochem Biophys Res Commun. 2014;453:277–281.
- Swiezewska E, Danikiewicz W. Polyisoprenoids: structure, biosynthesis and function. Prog Lipid Res. 2005;44:235–258.10.1016/j.plipres.2005.05.002
- Surmacz L, Swiezewska E. Polyisoprenoids – secondary metabolites or physiologically important superlipids? Biochem Biophys Res Commun. 2011;407:627–632.10.1016/j.bbrc.2011.03.059
- Akhtar TA, Surowiecki P, Siekierska H, et al. Polyprenols are synthesized by a plastidial cis-prenyltransferase and influence photosynthetic performance. Plant Cell. 2017;29(7):1709–1725.10.1105/tpc.16.00796
- Lipko A, Swiezewska E. Isoprenoid generating systems in plants – a handy toolbox how to assess contribution of the mevalonate and methylerythritol phosphate pathways to the biosynthetic process. Prog Lipid Res. 2016;63:70–92.
- Gawarecka K, Swiezewska E. Analysis of plant polyisoprenoids. Methods in Mol Biol. 2014;1153:135–147.10.1007/978-1-4939-0606-2
- Hemmerlin A, Harwood JL, Bach TJ. A raison d’être for two distinct pathways in the early steps of plant isoprenoid biosynthesis? Prog Lipid Res. 2012;51(2):95–148.10.1016/j.plipres.2011.12.001
- Rajbhandari I, Takamatsu S, Nagle DG. A new dehydrogeranylgeraniol antioxidant from Saururus cernuus that inhibits intracellular reactive oxygen species (ROS)-catalyzed oxidation within HL-60 cells. J Nat Prod. 2001;64(6):693–695.10.1021/np0101346
- Rowland RL, Latimer PH, Giles JA. Flue-cured tobacco. i. isolation of solanesol, an unsaturated alcohol. J Am Chem Soc. 1956;78(18):4680–4683.10.1021/ja01599a041
- Bamba T, Fukusaki E, Kajiyama S, et al. The occurrence of geometric polyprenol isomers in the rubber-producing plant, Eucommia ulmoides Oliver. Lipids. 2001;36:727–732.
- Bamba T, Fukusaki E, Minakuchi H, et al. Separation of polyprenol and dolichol by monolithic silica capillary column chromatography. J Lipid Res. 2005;46:2295–2298.10.1194/jlr.M500185-JLR200
- Aoki T, Matsuo K, Suga T, et al. Arachisprenols: polyprenols possessing a geranyl residue from arachis hypogaea. Phytochemistry. 1997;46(4):715–720.10.1016/S0031-9422(97)00206-9
- Gough DP, Hemming FW. The stereochemistry of betulaprenol biosynthesis. Biochem J. 1970;117:309–317.10.1042/bj1170309
- Ibata K, Mizuno M, Takigawa T, et al. Long-chain betulaprenol-type polyprenols from the leaves of Ginkgo biloba. Biochem J. 1983;213:305–311.10.1042/bj2130305
- Gough DP, Kirby AL, Richards JB, et al. The characterization of undecaprenol of Lactobacillus plantarum. Biochem J. 1970;118:167–170.10.1042/bj1180167
- Stone KJ, Wellburn AR, Hemming FW, et al. The characterization of ficaprenol-10, -11, and -12 from the leaves of Ficus elastica (decorative rubber plant). Biochem J. 1967;102:325–330.
- Suga T, Shishibori T, Nakaya K. Structure and biosynthesis of malloprenols from Mallotus japonicas. Phytochemistry. 1980;19(11):2327–2330.10.1016/S0031-9422(00)91020-3
- Fukawa H, Toyoda M, Shimizu T, et al. Isolation of new isoprenyl alcohols from silkworm feces. Tetrahedron Lett. 1966;7:6209–6213.10.1016/S0040-4039(00)70167-5
- Toyoda M, Fukawa H, Shimizu T. Studies on plant polyisoprenoids Part I. polyisoprenols of silkworm-feces and mulberry-leaf. J Agr Chem Soc Jpn. 1969;43(10):688–693.
- Wellburn AR, Stevenson J, Hemming FW, et al. The characterization and properties of castaprenol-11, -12, and -13 from the leaves of Aesculus hippocastanum (horse chesnut). Biochem J. 1967;102:313–324.10.1042/bj1020313
- Suga T, Shishibori T, Kosela S, et al. The structural elucidation and the biosynthetic study of cleomeprenols-9, -10, and -11 from the leaves of Cleome spinoza. Chem Lett. 1975;7:771–776.10.1246/cl.1975.771
- Toyoda M, Asahina M, Fukawa H, et al. Isolation of new acyclic C25-isoprenyl alcohol from potato leaves. Tetrahedron Lett. 1969;10:4879–4882.10.1016/S0040-4039(01)88836-5
- Suga T, Ohta S, Nakai A, et al. Glycinoprenols: novel polyprenols possessing a phytyl residue from the leaves of soybean. J Org Chem. 1989;54:3390–3393.10.1021/jo00275a026
- Sato M, Sato K, Nishikawa S, et al. The yeast RER2 gene, identified by endoplasmic reticulum protein localization mutations, encodes cis-prenyltransferase, a key enzyme in dolichol synthesis. Mol Cell Biol. 1999;19:471–483.10.1128/MCB.19.1.471
- Sato M, Fujisaki S, Sato K, et al. Yeast Saccharomyces cerevisiae has two cis-prenyltransferases with different properties and localization. Implication for their distinct physiological roles in dolichol synthesis. Genes Cells. 2001;6(6):495–506.10.1046/j.1365-2443.2001.00438.x
- Szkopinska A, Grabinska K, Delourme D, et al. Polyprenol formation in the yeast Saccharomyces cerevisiae: effect of farnesyl diphosphate synthase overexpression. J Lipid Res. 1997;38:962–968.
- Szkopinska A, Swiezewska E, Rytka J. Induction of the synthesis of an additional family of long-chain dolichols in the Saccharomyces cerevisiae. Effect of starvation and ageing. Acta Biochim. Polon. 2002;49(3):781–787.
- Tateyama S, Sagami H. Characterization of yeast mutant #64: occurrence of shorter-chain C55-60 polyprenol. Int Cong Ser. 2001;1223:65–71.
- Surmacz L, Wojcik J, Kania M, et al. Short-chain polyisoprenoids in the yeast Saccharomyces cerevisiae — new companions of the old guys. Biochim Biophys Acta. 2015;1851(10):1296–1303.10.1016/j.bbalip.2015.06.006
- Mankowski T, Jankowski W, Chojnacki T, et al. C55-dolichol: occurrence in pig liver and preparation by hydrogenation of plant undecaprenol. Biochemistry. 1976;15(10):2125–2130.10.1021/bi00655a015
- Mors WB, dos Santos Fo. MF, Monteiro HJ, et al. Chemoprophylactic agent in schistosomiasis: 14,15-epoxygeranylgeraniol. Science. 1967;157:950–951.
- Imai K, Katayama M, Marumo S. Chemical studies on fungal metabolism of isoprenoids. 19th International Symposium on the Chemical of Natural Products. Hiroshima; 1975. p. 17–24.
- Ishinaga M, Ueda A. Novel dolichyl derivatives in rat spleen. Biosci Biotechnol Biochem. 1999;63(2):449–451.10.1271/bbb.63.449
- Steen L, Van Dessel G, De Wolf M, et al. Identification and characterization of dolichyl dolichoate, a novel isoprenoic derivative in bovine thyroid. Biochim Biophys Acta. 1984;796:294–303.10.1016/0005-2760(84)90130-9
- Ward W, Zucca FA, Bellei C, et al. Neuromelanins in various regions of human brain are associated with native and oxidized isoprenoid lipids. Arch Biochem Biophys. 2009;484:94–99.10.1016/j.abb.2009.01.013
- Barton DHR, Jarman TR, Watson KG, et al. Assimilation of the antipodal forms of squalene 2,3-oxide by mammalian, yeast, and plant systems. J Chem Soc. 1974;21:861–862.
- Judy KJ, Schooley DA, Dunham LL, et al. Isolation, structure, and absolute configuration of a new natural insect juvenile hormone from Manduca sexta. Proc Natl Acad Sci USA, 1973;70(5):1509–1513.
- Burfield DR. Epoxy group responsible for crosslinking in natural rubber. Nature. 1974;249:29–30.10.1038/249029a0
- Tanaka Y. Structure and biosynthesis mechanism of natural polyisoprene. Prog Polym Sci. 1989;14:339–371.10.1016/0079-6700(89)90006-3
- Tanaka Y. Structural characterization of natural polyisoprenes: solve the mystery of natural rubber based on structural study. Rubber Chem Technol. 2001;74(3):355–375.10.5254/1.3547643
- Lewinsohn E, Sitrit Y, Bar E, et al. Carotenoid pigmentation affects the volatile composition of tomato and watermelon, as revealed by comparative genetic analyses. J Agric Food Chem. 2005;53(8):3142–3148.10.1021/jf047927t
- Toyoda M, Fukawa H, Shimizu T. Isolation of new polyisoprenyl ketone from silkworm feces. Tetrahedron Lett. 1968;9:3837–3840.10.1016/S0040-4039(01)99114-2
- Toyoda M, Fukawa H, Shimizu T. Studies on plant polyisoprenoids II. The polyisoprenoid ketone of silkworm-feces and mulberry-leaf. J Agr Chem Soc Jpn. 1970; 44(1): 40–45.
- Murakami M, Oketani K, Fujisaki H, et al. Effect of synthetic acyclic polyisoprenoids on the cold-restraint stress induced gastric ulcer in rats. Jpn J Pharmacol. 1983;33:549–556.
- Inoue H, Korenaga T, Sagami H, et al. Formation of farnesal and 3-hydroxy-2,3-dihydrofarnesal from farnesol by protoplasts of Botryococcus braunii. Biochem Biophys Res Commun. 1993;196(3):1401–1405.10.1006/bbrc.1993.2408
- Mooibroek H, Cornish K. Alternative sources of natural rubber. Appl Microbiol Biotechnol. 2000;53(4):355–365.10.1007/s002530051627
- Luo Q, Hiessl S, Poehlein A, et al. Insights into the microbial degradation of rubber and gutta-percha by analysis of the complete genome of Nocardia Nova SH22a. Appl Environ Microbiol. 2014;80(13):3895–3907.10.1128/AEM.00473-14
- Thelin A, Löw P, Chojnacki T, et al. Covalent binding of dolichyl phosphate to proteins in rat liver. Eur J Biochem. 1991;195:755–761.
- Hjerman M, Wejde J, Dricu A, et al. Evidence for protein dolichylation. FEBS Lett. 1997;416:235–238.10.1016/S0014-5793(97)01208-8