Abstract
Owing to their photosynthetic capabilities, there is increasing interest in utilizing cyanobacteria to convert solar energy into biomass. 2-Deoxy-scyllo-inosose (DOI) is a valuable starting material for the benzene-free synthesis of catechol and other benzenoids. DOI synthase (DOIS) is responsible for the formation of DOI from d-glucose-6-phosphate (G6P) in the biosynthesis of 2-deoxystreptamine-containing aminoglycoside antibiotics such as neomycin and butirosin. DOI fermentation using a recombinant Escherichia coli strain has been reported, although a carbon source is necessary for high-yield DOI production. We constructed DOI-producing cyanobacteria toward carbon-free and sustainable DOI production. A DOIS gene derived from the butirosin producer strain Bacillus circulans (btrC) was introduced and expressed in the cyanobacterium Synechococcus elongatus PCC 7942. We ultimately succeeded in producing 400 mg/L of DOI in S. elongatus without using a carbon source. DOI production by cyanobacteria represents a novel and efficient approach for producing benzenoids from G6P synthesized by photosynthesis.
Carbon-free production of 2-deoxy-scyllo-inosose (DOI) in cyanobacterium Synechococcus elongatus PCC 7942.
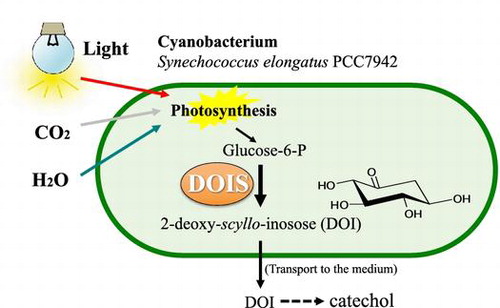
Cyanobacteria are photosynthetic microorganisms that are believed to be the ancestors of chloroplasts [Citation1]. Cyanobacteria thrive in various environments including the ocean, fresh water, deserts, and the polar regions [Citation2]. Recently, cyanobacteria have been studied as promising hosts for the production of biofuels and biomaterials because they can directly convert CO2 to biomass and beneficial metabolites by photosynthesis, and they grow more rapidly than plants [Citation3]. Therefore, production systems using cyanobacteria are expected to contribute to sustainable energy generation, and to mitigate the effects of global warming by the uptake of atmospheric CO2 [Citation4,5].
2-Deoxy-scyllo-inosose (DOI) is a six-membered carbocycle formed from d-glucose-6-phosphate (G6P) catalyzed by 2-deoxy-scyllo-inosose synthase (DOIS), a key enzyme in the biosynthesis of 2-deoxystreptamine-containing aminocyclitol antibiotics [Citation6–9]. DOI is valuable as a starting material for the benzene-free synthesis of catechol and other benzenoids [Citation10]. We demonstrated that DOI is reductively dehydrated to catechol in the presence of hydrogen iodide under acidic conditions [Citation10]. Catechol is important as a starting material for pharmaceuticals, flavorings, agrochemicals, polymerization inhibitors, and antioxidants. The preparation of hydroquinone from DOI in two chemical steps has also been described by Hansen and Frost [Citation11].
DOIS derived from the butirosin producer Bacillus circulans SANK 72073 was originally isolated as a heterodimer comprising two subunits: a 40-kDa subunit and a 20-kDa subunit [Citation9]. The genes encoding both subunits have been separately cloned and functionally characterized to reveal that only the large subunit (BtrC) is needed for the catalytic activity of DOIS [Citation12,13]. Kogure et al. reported effective DOI production by a recombinant Escherichia coli strain using the btrC gene [Citation14]. They achieved a DOI production yield of 99% from d-glucose by the disruption of pgi, zwf, and pgm genes, which encode phosphoglucose isomerase, G6P dehydrogenase, and phosphoglucomutase, respectively, although mannitol was required as a supplemental carbon source.
We constructed DOI-producing cyanobacteria toward carbon-free and sustainable DOI production. A DOIS gene derived from the butirosin producer strain Bacillus circulans (btrC) was introduced and expressed in the cyanobacterium Synechococcus elongatus PCC 7942. We ultimately succeeded in producing 400 mg/L of DOI in S. elongatus without using a carbon source. DOI production by cyanobacteria represents a novel and efficient approach to producing benzenoids from G6P synthesized by photosynthesis.
Materials and methods
Culture conditions
Synechococcus elongatus strain PCC 7942_TUA wild-type (our laboratory stock) [Citation15] and its derivatives were grown photoautotrophically at 30 °C in 800 mL of BG-11 medium; air was bubbled through the medium, which was illuminated continuously (40 μmol photon m−2 s−1) as a standard condition. When necessary, spectinomycin and chloramphenicol were added to the medium at final concentrations of 40 and 7.5 μg/mL, respectively. Isopropyl β-d-1-thiogalactopyranoside (IPTG; 1 mM final concentration) was added to induce the btrC gene in the chromosomal neutral site.
The light intensity was increased stepwise and 5% CO2 was bubbled through the medium to promote the growth of the strain carrying the pEX1-btrC plasmid. During the late exponential growth phase (optical density at 750 nm (OD750) = 1–3), the light intensity was increased to 135 μmol photon m−2 s−1. When the culture reached the stationary phase (more than OD750 = 3), the light intensity was increased to 400 μmol photon m−2 s−1.
Construction of plasmids for the expression of btrC
The DNA fragment encoding btrC was amplified by PCR from pDS4 [Citation9], which contains btrC gene in Bacillus circulans SANK 72073, with the primers listed in Table . These fragments were cloned into the integration vector pNSE1 [Citation16] by restriction enzyme digestion and ligation. The resulting plasmids were named pNSE1-btrC. For the construction of the pEX1-btrC plasmid, the fragment containing the trc promoter and the btrC gene were amplified from pNSE1-btrC by PCR with the primers (Table ) and cloned into the XbaI/XhoI site in pUC303 [Citation17]. All the plasmid constructions were confirmed by DNA sequencing. The resulting plasmids (pNSE1-btrC and pEX1-btrC) were used to transform the S. elongatus strain into a spectinomycin- or chloramphenicol-resistant strain.
Table 1. Oligonucleotide primers used in this study.
SDS-PAGE and western blotting analysis
Crude extracts from the Synechococcus were prepared as described previously [Citation18]. These proteins (10 μg) were investigated using SDS-PAGE. For the detection of BtrC proteins, rabbit antiserum against BtrC protein was used as the primary antibody [Citation19], and horseradish peroxidase-conjugated anti-rabbit immunoglobulin G antibody (GE) was used as the secondary antibody.
Measurements of DOI concentration
The DOI was analyzed by HPLC after derivatization with O-(4-nitrobenzyl) hydroxylamine, according to the method described in a previous report [Citation9]. The concentration of DOI was calculated based on the standard curve for pure synthetic DOI [Citation20].
Results and discussion
Construction of btrC-expressing strains
We constructed btrC-expressing strains of S. elongatus to produce DOI. The btrC gene under the control of the trc promoter was introduced into the chromosomal neutral site in S. elongatus (NS-btrC) (Figure (A)). The trc promoter, which is controlled by IPTG, exhibits strong transcriptional activity in cyanobacteria [Citation21]. BtrC expression was observed in the crude extract 24 h after induction with 1 mM IPTG (Figure (B)). Western blotting analysis using an anti-BtrC antiserum revealed an IPTG-dependent signal (Figure (C)). The amounts of DOI in the culture (OD750 = 1 and 2) were determined by HPLC. We achieved 8.9 and 10.0 mg/L DOI production in the supernatant of the culture. These results indicate that BtrC protein can function as a DOIS in S. elongatus PCC 7942 cells, and DOI is transported to the medium from the cell, as previously reported in E. coli [Citation14].
In E. coli, to yield a large amount of DOI, expression level of BtrC was enhanced by using a strong promoter and high-copy plasmid [Citation14]. Since the expression level in S. elongatus strain carrying NS-btrC is insignificant comparing to that in E. coli [Citation14], we next designed a pEX1-btrC plasmid to create a more effective system for producing DOI. The trc promoter and btrC gene were transferred to the multi-copy plasmid pUC303 in S. elongatus (Figure (A)). Constitutive and high-level expression of BtrC was expected because this construct did not contain the repressor gene lacIq. SDS-PAGE analysis exhibited clear BtrC bands, and 100 mg/L DOI production was achieved (Figures (B) and 3(A)). Compared with the control strain, which contained an empty vector, the strain carrying the pEX1-btrC plasmid exhibited severe growth deficiency (Figure (C)). We assumed that the growth defect was caused by insufficient levels of G6P, which is a substrate of BtrC. The G6P deprivation perturbs cellular metabolism because cyanobacteria utilize G6P for not only central carbon pathway, but also the intracellular carbon storage, such as sucrose and glycogen. Therefore, we next investigated DOI production under photosynthesis promoting conditions.
DOI production under high-intensity light and CO2 bubbling
To investigate the effect of photosynthesis activation on DOI production, we cultured a strain containing the pEX1-btrC plasmid under the optimized light condition while bubbling 5% CO2 through the culture medium. Because irradiation is limited by the self-shading of the cell, the light intensity was increased stepwise with cell growth, as described in the Materials and Methods section. As expected, the cell density increased continuously, and ultimately reached OD750 = 6 in the stationary phase culture. Under these conditions, the DOI level in the medium also increased by approximately fourfold compared with the standard growth condition (air bubbling in atmosphere (0.03% CO2); illumination: 40 μmol photon m−2 s−1). These results indicate that DOI production is correlated with photosynthesis in S. elongatus.
The high-level expression of BtrC protein using plasmid vector pEX1 resulted in the production of 400 mg/L of DOI (Figure (B)). It is still 75 times lower comparing to the maximum production in E. coli (29.6 g/L) [Citation14]. However, this E. coli strain harbors multiple mutations for metabolic engineering and requires for not only glucose, but also mannitol as an additional carbon source. In E. coli without these modification, the production rate is comparable level (1.5 g/L) to our transformant. Our DOI-producing system using cyanobacteria do not require the carbon source, because the DOI is produced entirely by photosynthesis. Thus, this is a cost-effective and ecological DOI production system. In addition, DOI production was significantly improved by optimizing the growth conditions (Figure (B)).
One strategy to increase product yield is to reduce carbon flow into competing pathways. An improvement in DOI production by shutting-off G6P metabolism has been reported in E. coli [Citation14]. In cyanobacterium Synechocystis sp. PCC 6803, the deletion of the glgC gene for ADP-glucose pyrophosphorylase, which synthesizes the glycogen precursor ADP-glucose, resulted in enhanced ethanol production, although reduced fitness and stress tolerance were reported [Citation22]. Recently, the S. elongatus strain UTEX 2973 has been described as the fastest-growing cyanobacterium [Citation23]. This is a promising candidate for DOI production among freshwater cyanobacteria. Culture and genetic engineering studies are necessary for the further production of DOI in cyanobacteria.
Author contribution
H.Y., T.E., and K.K. designed the concept and the experiments of this study; S.W., H.O., H.K., T.H. and F.K. performed the experiments; S.W., K.N. and F.K. analyzed and interpreted the data; S.W. and F.K. wrote the manuscript.
Disclosure statement
No potential conflict of interest was reported by the authors.
Funding
This work was supported by grants-in-aid [grant number 17H05451], [grant number 17H05434] from the Ministry of Education, Culture, Sports, Science, and Technology of Japan to S.W. and F. K.
Acknowledgments
We would like to thank Mayu Akagi for technical assistance.
References
- Hohmann-Marriott MF, Blankenship RE. Evolution of photosynthesis. Annu Rev Plant Biol. 2011;62:515–548.10.1146/annurev-arplant-042110-103811
- Herrero A, Flores F. The cyanobacteria: molecular biology, genomics, and evolution. Norfolk (VA): Caister Academic Press; 2008.
- Anemaet IG, Bekker M, Hellingwerf KJ. Algal photosynthesis as the primary driver for a sustainable development in energy, feed, and food production. Mar Biotechnol. 2010;12:619–629.10.1007/s10126-010-9311-1
- Angermayr SA, Gorchs Rovira A, Hellingwerf KJ. Metabolic engineering of cyanobacteria for the synthesis of commodity products. Trends Biotechnol. 2015;33:352–361.10.1016/j.tibtech.2015.03.009
- Hagemann M, Hess WR. Systems and synthetic biology for the biotechnological application of cyanobacteria. Curr Opin Biotechnol. 2017;49:94–99.
- Kudo F, Eguchi T. Biosynthetic genes for aminoglycoside antibiotics. J Antibiot. 2009;62:471–481.10.1038/ja.2009.76
- Kudo F, Eguchi T. Biosynthetic enzymes for the aminoglycosides butirosin and neomycin. Methods Enzymol. 2009;459:493–519.10.1016/S0076-6879(09)04620-5
- Kudo F, Eguchi T. Aminoglycoside antibiotics: new insights into the biosynthetic machinery of old drugs. Chem Record. 2016;16:4–18.10.1002/tcr.v16.1
- Kudo F, Hosomi Y, Tamegai H, et al. Purification and characterization of 2-deoxy-scyllo-inosose synthase derived from bacillus circulans. A crucial carbocyclization enzyme in the biosynthesis of 2-deoxystreptamine-containing aminoglycoside antibiotics. J Antibiot. 1999;52:81–88.10.7164/antibiotics.52.81
- Kakinuma K, Nango E, Kudo F, et al. An expeditious chemo-enzymatic route from glucose to catechol by the use of 2-deoxy-scyllo-inosose synthase. Tetrahedron Lett. 2000;41:1935–1938.10.1016/S0040-4039(00)00064-2
- Hansen CA, Frost JW. Deoxygenation of polyhydroxybenzenes: an alternative strategy for the benzene-free synthesis of aromatic chemicals. J Am Chem Soc. 2002;124:5926–5927.10.1021/ja0176346
- Kudo F, Tamegai H, Fujiwara T, et al. Molecular cloning of the gene for the key carbocycle-forming enzyme in the biosynthesis of 2-deoxystreptamine-containing aminocyclitol antibiotics and its comparison with dehydroquinate synthase. J Antibiot. 1999;52:559–571.10.7164/antibiotics.52.559
- Tamegai H, Nango E, Koike-Takeshita A, et al. Significance of the 20-kDa subunit of heterodimeric 2-deoxy-scyllo-inosose synthase for the biosynthesis of butirosin antibiotics in Bacillus circulans. Biosci Biotechnol Biochem. 2002;66:1538–1545.10.1271/bbb.66.1538
- Kogure T, Wakisaka N, Takaku H, et al. Efficient production of 2-deoxy-scyllo-inosose from d-glucose by metabolically engineered recombinant Escherichia coli. J Biotechnol. 2007;129:502–509.10.1016/j.jbiotec.2007.01.016
- Watanabe S, Ohbayashi R, Shiwa Y, et al. Light-dependent and asynchronous replication of cyanobacterial multi-copy chromosomes. Mol Microbiol. 2012;83:856–865.10.1111/j.1365-2958.2012.07971.x
- Kato H, Chibazakura T, Yoshikawa H. NblR is a novel one-component response regulator in the cyanobacterium Synechococcus elongatus PCC 7942. Biosci Biotechnol Biochem. 2008;72:1072–1079.10.1271/bbb.70816
- Kuhlemeier CJ, Thomas AA, van der Ende A, et al. A host-vector system for gene cloning in the cyanobacterium Anacystis nidulans R2. Plasmid. 1983;10:156–163.10.1016/0147-619X(83)90068-9
- Nimura K, Takahashi H, Yoshikawa H. Characterization of the dnaK multigene family in the cyanobacterium Synechococcus sp. strain PCC7942. J Bacteriol. 2001;183:1320–1328.10.1128/JB.183.4.1320-1328.2001
- Tamegai H, Sawada H, Nango E, et al. Roles of a 20 kDa protein associated with a carbocycle-forming enzyme involved in aminoglycoside Biosynthesis in primary and secondary metabolism. Biosci Biotechnol Biochem. 2010;74:1215–1219.10.1271/bbb.90961
- Yamauchi N, Kakinuma K. Biochemical studies on 2-deoxy-scyllo-inosose, an early intermediate in the biosynthesis of 2-deoxystreptamine. III. Confirmation of in vitro synthesis of 2-deoxy-scyllo-inosose, the earliest intermediate in the biosynthesis of 2-deoxystreptamine, using cell free preparations of Streptomyces fradiae. J Antibiot. 1992;45:774–780.10.7164/antibiotics.45.774
- Huang HH, Camsund D, Lindblad P, et al. Design and characterization of molecular tools for a synthetic Biology approach towards developing cyanobacterial biotechnology. Nucleic Acids Res. 2010;38:2577–2593.10.1093/nar/gkq164
- Namakoshi K, Nakajima T, Yoshikawa K, et al. Combinatorial deletions of glgC and phaCE enhance ethanol production in Synechocystis sp. PCC 6803. J Biotechnol. 2016;239:13–19.10.1016/j.jbiotec.2016.09.016
- Yu J, Liberton M, Cliften PF, et al. Synechococcus elongatus UTEX 2973, a fast growing cyanobacterial chassis for biosynthesis using light and CO2. Sci Rep. 2015;5:742.10.1038/srep08132