Abstract
Accumulating evidence indicates that skeletal muscle secrets proteins referred to as myokines and that exercise contributes to their regulation. In this study, we propose that chemokine (C-X-C motif) ligand 10 (CXCL10) functions as a novel myokine. Initially, we stimulated differentiated C2C12 myotubes with or without electrical pulse stimulation (EPS) to identify novel myokines. Cytokine array analysis revealed that CXCL10 secretion was significantly reduced by EPS, which was further confirmed by enzyme-linked immunosorbent assay and quantitative polymerase chain reaction analysis. Treadmill experiments in mice identified significant reduction of Cxcl10 gene expression in the soleus muscle. Additionally, contraction-dependent p38 MAPK activation appeared to be involved in this reduction. Furthermore, C2C12 conditioned medium obtained after applying EPS could induce survival of MSS31, a vascular endothelial cell model, which was partially attenuated by the addition of recombinant CXCL10. Overall, our findings suggest CXCL10 as a novel exercise-reducible myokine, to control endothelial cell viability.
CXCL10 as a novel exercise-reducible myokine.
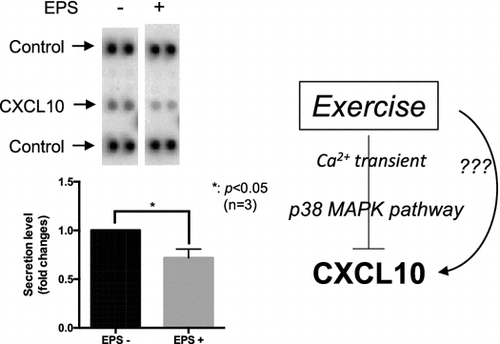
Keywords:
Abbreviations:
- AICAR: 5-aminoimidazole-4-carboxamide-1-β-D- ribofuranoside
- CS: calf serum
- CXCL10: chemokine (C-X-C motif) ligand 10
- EDL: extensor digitorum longus
- ELISA: enzyme-linked immunosorbent assay
- EPS: electrical pulse stimulation
- IP-10: interferon gamma-induced protein 10
- qPCR: quantitative polymerase chain reaction
- quad: quadriceps
Skeletal muscle possesses multiple functions to maintain homeostasis in the body. Recently, it has been widely recognized that skeletal muscle is an endocrine organ, producing numerous types of secreted proteins referred to as myokines that potentially transduce signals to other tissues/organs [Citation1]. Various efforts have been made to identify these myokines, resulting in the identification of over 600 secreted proteins as myokines to date [Citation2]. The precise roles of these myokines have been extensively studied in many laboratories; with skeletal muscle being recognized as an important tissue that produces orchestrated cell-cell communication via these myokine networks.
Homeostatic disruption of skeletal muscle by factors such as change in exercise level dramatically modifies the secretome of myokines [Citation3,4]. The majority of such exercise-dependent myokines are categorized as exercise-inducible myokines, the secretion of which is up-regulated by exercise. Conversely, these are few examples of exercise-reducible myokines with the exception that myostatin, known to inhibit skeletal muscle cell differentiation, is significantly reduced in response to exercise [Citation5,6]. Overall, discovery of these exercise-dependent myokines, whether up- or down-regulated by exercise, may serve as the missing links between skeletal muscle cell contraction and the physiological changes in the other tissues/organs during or after exercise.
Exercise involving skeletal muscle cell contraction exerts the activation of multiple signaling cascades [Citation7–9]. For example, physiologically, the contraction-dependent changes in membrane potentials mobilize intracellular calcium waves, termed the [Ca2+]i transient, followed by Ca2+-dependent signaling activation [Citation10]. Adenosine mono-phosphate kinase (AMPK) activation constitutes another well-known signaling mechanism that is activated by skeletal muscle cell contraction [Citation8]. Moreover, the stress-dependent mitogen-activated kinase (MAPK) activation is also reported to be modulated during cell contraction [Citation7]. Thus, a crucial question in myokine studies relates to which types of exercise-activated signaling mechanisms participate in myokine regulation.
Our initial screening indicated that chemokine (C-X-C motif) ligand 10 (CXCL10), also known as interferon gamma-induced protein 10 (IP-10), may represent another member of exercise-reducible myokines. CXCL10 is a multifunctional chemokine that exerts its biological actions through a seven-transmembrane G protein coupled receptor, CXCR3. CXCL10 has been known to employ homing functions to attract CXCR3-positive cells to the sites of inflammation [Citation11,12]. In addition, CXCL10 appears to induce cellular apoptosis in various cell types through activation of caspase-3; for example, treatment of fetal neurons by CXCL10 increased its apoptotic rates by activating the classical caspase cascade [Citation13,14]. Moreover, numerous lines of evidence indicate that CXCL10 also functions as an angiostatic factor, suppressing angiogenesis [Citation15–17], by antagonizing the angiogenic activities of other angiogenic factors, such as basic fibroblast growth factor and vascular endothelial growth factor (VEGF) [Citation18].
The main aim of the present study was to identify novel myokines by our established method, which is composed of mouse C2C12 myotubes and an electrical pulse stimulator. As our initial screening suggested CXCL10 as a candidate novel myokine whose expression was reduced by skeletal muscle cell contraction, we further confirmed this reduction by enzyme-linked immunosorbent assay (ELISA), quantitative polymerase chain reaction (qPCR), and animal experiments. Furthermore, we also analyzed the intracellular signaling pathways that are responsible for contraction-dependent CXCL10 reduction as well as its potential roles in exercise-dependent angiogenesis.
Materials and methods
Materials
The ECL PrimeTM western blotting kit was purchased from GE Healthcare Inc. (Rockford, IL, USA). Dulbecco’s modified Eagle medium (DMEM), penicillin/streptomycin, and trypsin-EDTA were purchased from Nacalai Tesque (Kyoto, Japan). Cell culture equipment was from Corning Inc. (Armonk, NY, USA). Fetal bovine serum (FBS) was obtained from BioWest (Nuaille, France). Unless otherwise specified, all chemicals were of the purest grade available and were purchased from Nacalai Tesque, Sigma Chemicals (St. Louis, MO, USA), or Wako Pure Chemical Industries, Ltd. (Osaka, Japan).
Animal experiments
All animal experiments were approved by the Animal Care Committee at Toyo University. Male C57B6/J mice (Charles-River Japan, Inc., Tokyo, Japan; 8 weeks of age at the beginning of the experimental protocol) were individually housed in a temperature- and humidity-controlled room. The animals were fed chow (CLEA Japan, Inc., Tokyo, Japan) and water ad libitum and were acclimatized to a 12 h light cycle (lights on between 0600 and 1800 h) for a period of 1 week before experimental manipulation. Mice were divided into two groups of which one group received treadmill training (15 cm/s, 30 min, 8% slope, once). Then, the mice were anesthetized and a heart blood sample was collected. Following this collection, mice were immediately sacrificed and the extensor digitorum longus (EDL), quadriceps (quad), and soleus muscles were removed and washed in phosphate buffered saline (−). Total RNAs were isolated using TRIzol reagents according to the manufacturer’s protocol (Invitrogen Corp., Carlsbad, CA, USA).
Cell culture
The mouse skeletal muscle cell line, C2C12, was maintained in DMEM containing 10% FBS, 30 μg/mL penicillin, and 100 μg/mL streptomycin at 37 °C under a 5% CO2 atmosphere. The medium was exchanged every 72 h. For the experiments, cells were grown in 8-well plates (Thermo Fisher Scientific Inc., Waltham, MA, USA) at a density of 5 × 104 cells/well in 3 mL growth medium, or in 6-well plates (Corning) at a density of 5 × 104 cells/well in 2 mL growth medium. At three days after plating, cells typically reached 100% confluence and the differentiation of cells was then induced by switching the medium to differentiation medium (DMEM supplemented with 2% calf serum (CS), 30 μg/mL penicillin, and 100 μg/mL streptomycin). The medium was exchanged every 24 h during differentiation induction.
MSS31 cells, which exhibit properties of endothelial cells [Citation19], were maintained in DMEM containing 10% FBS, 30 μg/mL penicillin, and 100 μg/mL streptomycin at 37 °C under a 5% CO2 atmosphere. The medium was exchanged every 72 h.
Electrical pulse stimulation (EPS)
Differentiated C2C12 myotubes were cultured in 8-well plates (Thermo Fisher Scientific) in differentiation medium and placed in a chamber for electrical stimulation (C-Dish; IonOptix, Milton, MA, USA). EPS (20 V/30 mm, 1 Hz, 2 ms) was then applied to the cells using the C-Pace pulse generator (C-Pace 100; IonOptix).
Cytokine array analysis
Cytokine antibody array was performed using a Proteome Profiler mouse cytokine array kit (Panel A; R&D Systems, Minneapolis, MN, USA), according to the manufacturer’s protocol. Briefly, C2C12 cell culture supernatants were collected and applied to a membrane precoated with capture antibodies. After washing, the detection antibody was added and the membrane was visualized after the addition of secondary streptavidin-horseradish peroxidase (HRP) and ECL reagents. The intensity of each spot was analyzed using ImageJ software (National Institutes of Health, Bethesda, MD, USA).
ELISA
Concentrations of CXCL10 in C2C12 culture supernatants or mouse serum were measured using commercially available ELISA kits (R&D Systems).
Western blotting
The expression and phosphorylation of each protein were analyzed by western blot analysis. The cells were seeded in 8-well plates at a density of 1 × 105 cells/well. Differentiation was induced as described above and the cells were subsequently subjected to EPS. The cell lysates were prepared using lysis buffer (2% sodium dodecyl sulfate (SDS), 1% 2-mercaptoethanol, 10% glycerol, 0.0033% bromophenol blue, and 50 mM Tris-Cl [pH 6.8]). The cell lysates were resolved using 12% SDS-polyacrylamide gel electrophoresis (1:30, bis:acrylamide). Proteins were transferred to a polyvinylidene difluoride membrane (Immobilon-P; Millipore Corp, Bedford, MA, USA), and the membranes were blocked by incubation with 3% bovine serum albumin in Tris buffered saline containing 0.1% Tween-20 for 30 min. Each protein was detected by 1-h incubation with primary anti-phospho AMPK (Thr172), anti-AMPK, anti-phospho p38, anti-p38, and anti-β-actin antibodies (dilution 1:1000; Cell Signaling Technology, Danvers, MA, USA). Specific proteins were visualized after subsequent incubation with HRP-conjugated anti-mouse or rabbit IgG (Cell Signaling Technology) and an ECL PrimeTM detection procedure (GE Healthcare). The intensity of protein bands was quantified using ImageJ software.
Reverse transcription-qPCR analysis
C2C12 cells were differentiated as described previously and cultured in the presence or absence of EPS for 0–24 h. Total RNA was isolated from the cells using a NucleoSpinTM RNA Isolation Kit (TaKaRa Bio Inc., Shiga, Japan) according to the manufacturer’s protocol. cDNAs were synthesized from total RNA using the ReverTra Ace qPCR RT Master Mix (Toyobo Co. Ltd., Osaka, Japan). Fluorescence real-time qPCR analysis was performed using a StepOne instrument (Life Technologies Corporation; Grand Island, NY, USA) and an SYBR Green detection kit (KAPA Biosystems Inc., Woburn, MA, USA). PCR primers for measuring each gene were: mouse Cxcl10, 5′- TCC CTC TCG CAA GGA C -3′ and 5′- TTG GCT AAA CGC TTT CAT -3′; and mouse Gapdh, 5′- TGT GTC CGT CGT GGA TCT GA -3′ and 5′- CGT GCT TCA CCA CCT TCT TGA -3′.
MTT assay
MSS31 cells were seeded on 96-well plates at a density of 5 × 103 cells/well and were cultured for 72 h in DMEM containing 10% FBS, 30 μg/mL penicillin, and 100 μg/mL streptomycin. After 24 h of incubation with serum-free DMEM, the cell culture medium was exchanged into C2C12 conditioned medium, which was obtained from differentiated C2C12 cell culture for 24 h with or without EPS in the presence or absence of recombinant CXCL10 (rCXCL10) (final concentration of CXCL10; 125 pg/mL). The cell viability was evaluated using the MTT Cell Count Kit (Nacalai Tesque) according to the manufacturer’s protocol.
Statistical analysis
Differences in treatment groups were tested using one-way ANOVA with Tukey’s post-tests or Student’s t-test. Differences with p < 0.05 were considered statistically significant.
Results
Identification of CXCL10/IP-10 as a potential novel myokine
To identify novel myokines, we used our in vitro contractile system, which consists of C2C12 myotubes and EPS. In detail, fully differentiated C2C12 myotubes were stimulated with or without EPS for 24 h, then the conditioned medium was collected for analyzing contraction-dependent secreted proteins by application to the Proteome Profiler mouse cytokine array (Panel A). As shown in Figure (a), the secretion of CXCL10, assessed by cytokine array, was significantly reduced by applying EPS (p < 0.05, n = 3). Because the cytokine array might not provide a completely quantitative measurement, we further performed ELISA to evaluate the exact amounts of CXCL10 released from C2C12 myotubes. In the absence of EPS, approximately 125 pg/mL CXCL10 was observed in our experimental condition, whereas only approximately 100 pg /mL was detected in the presence of EPS (p < 0.05, n = 4) (Figure (b)). These results clearly indicated that the secretion of CXCL10 from C2C12 myotubes was significantly inhibited by EPS. Furthermore, we found that the gene expression of CXCL10 in C2C12 myotubes was also significantly attenuated by applying EPS (p < 0.05, n = 3) (Figure (c)). These findings suggested that CXCL10 constitutes a contraction-reducible myokine in C2C12 myotubes and that the reduced secretion of CXCL10 was, at least in part, explained by its regulation at the level of transcription.
Figure 1. Identification of CXCL10 as a contraction-reducible myokine in the C2C12 cell model. (a) and (b) Differentiated C2C12 myotubes were cultured in differentiation medium in the presence or absence of EPS (20 V, 1 Hz, 2 ms). (a) After 24 h, culture supernatants were subjected to cytokine array analysis. Three independent experiments were performed, and the intensity of each spot was measured using ImageJ software. The graph represents the means ± SEM obtained from these experiments (*p < 0.05, n = 3, t-test). (b) After 24 h, culture supernatants were collected and subjected to ELISA for evaluating CXCL10 concentration. The graph represents the means ± SEM (*p < 0.05, n = 4, t-test). (c) After 24 h, total RNA was isolated and Cxcl10 gene expression was measured by qPCR analysis (*p < 0.05, n = 3, t-test).
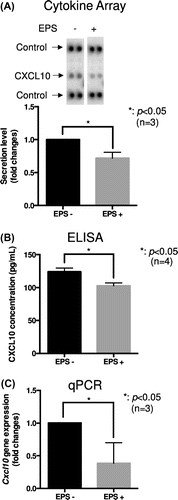
Figure 2. Treadmill running in mice reduced Cxcl10 gene expression in the soleus muscle. (a)–(d) Male C57B6/J mice were subjected to forced treadmill exercise (15 cm/s, 30 min, 8% slope, once). The exercised and control mice were anesthetized and a heart blood sample was collected. The mice were then immediately sacrificed and extensor digitorum longus (EDL), quadriceps (quad), and soleus muscles were removed. (a)–(c) Cxcl10 gene expression in the soleus (a), quad (b), and EDL (c) muscles was determined by qPCR analysis. The graph represents the means ± SEM (**p < 0.01, n = 4, t-test). (d) Serum CXCL10 concentration was determined by ELISA. The graph represents the means ± SEM (n = 4).
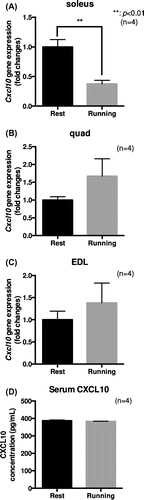
Forced treadmill running in mice decreases CXCL10 gene expression in the soleus
To confirm whether exercise in mice alters serum CXCL10 concentration and/or Cxcl10 gene expression in skeletal muscle, we utilized a forced treadmill exercise model that we reported previously [Citation20]. We first compared the gene expression of Cxcl10 in the quad, EDL, and soleus muscle. Cxcl10 gene expression, normalized by Gapdh gene expression, was predominantly observed in the soleus, even though lesser expression was observed in the other muscles such as the quad and EDL muscle (Figure S1). Forced treadmill running significantly decreased Cxcl10 gene expression in the soleus (p < 0.01, n = 4) (Figure (a)). On the other hand, Cxcl10 gene expression in the quad and EDL muscle tended to be increased; however, these differences were not significant statistically (n = 4) (Figure (b) and (c)). Although serum CXCL10 concentration was not altered by treadmill running (n = 4) (Figure (d)), we concluded that our observation in C2C12 myotubes of decreased Cxcl10 gene expression by EPS is indeed physiologically relevant because similar gene expression control was observed in mouse soleus muscle during treadmill running experiments.
Potential mechanisms of EPS-dependent CXCL10 reduction
We next analyzed the molecular mechanism(s) by which Cxcl10 gene reduction is regulated by cell contraction in C2C12 myotubes. We previously reported that EPS-induced contraction in C2C12 myotubes is dependent on increases in [Ca2+]i [Citation21]. Accordingly, the addition of 100 μM verapamil, a calcium channel blocker, completely abolished EPS-dependent Cxcl10 gene reduction (Figure (a)) as well as C2C12 cell contraction (data not shown). For further confirmation of the involvement of Ca2+ transients on Cxcl10 gene reduction, we tested the effects of several other calcium chelators such as BAPTA-AM and EGTA. When EPS was applied on C2C12 myotubes in the presence of 2 mM EGTA or 10 μM BAPTA-AM, contraction-dependent Cxcl10 gene reduction was completely abolished (Figure S2A and B). This series of experiments clearly indicated that Ca2+ transients generated by EPS play fundamental roles in Cxcl10 gene reduction. We also tested the effects of the AMPK activator 5-aminoimidazole-4-carboxamide-1-β-D-ribofuranoside (AICAR) on Cxcl10 gene expression. EPS significantly induced phosphorylation of AMPK (p < 0.05, n = 6) (Figure S3A); however, conversely, AICAR treatment instead enhanced Cxcl10 gene expression (p < 0.05, n = 5) (Figure S3B), which suggested that the AMPK activation induced by cell contraction potentially increased this gene expression. Moreover, because we observed significant induction of p38 phosphorylation by EPS up to 6 h in C2C12 myotubes (Figure (b)), which was abolished by the addition of 100 μM verapamil (Figure (c)), the effects of a p38 MAP kinase inhibitor on EPS-dependent reduction of Cxcl10 gene expression were also examined. As shown in Figure (d), administration of a specific p38 MAP kinase inhibitor, SB203580 (5 μM), partially attenuated the EPS-dependent reduction of Cxcl10 gene expression (p < 0.01, n = 4–6) (Figure (d)). Overall, these findings suggest that a Ca2+ transient followed by p38 MAP kinase activation by EPS may be important for Cxcl10 gene expression control in C2C12 myotubes.
Figure 3. Potential involvement of Ca2 + transients and p38 MAPK activation in contraction-induced CXCL10 reduction. (a) Differentiated C2C12 myotubes were cultured in differentiation medium with or without EPS for 24 h in the presence or absence of 100 μM verapamil. Cxcl10 gene expression was determined by qPCR analysis. The graph represents the means ± SEM (*p < 0.05, N.S.; not significant, n = 4, one-way ANOVA). (b) Differentiated C2C12 myotubes were stimulated by EPS for the indicated times. After harvesting cells, equal amounts of proteins from each sample were subjected to western blotting analysis. The experiment was repeated four times, and a representative result is shown. (c) Differentiated C2C12 myotubes were stimulated by EPS for 6 h in the presence or absence of 100 μM verapamil. Phosphorylation p38 were detected by western blotting analysis. The graph represents the means ± SEM (*p < 0.05, n = 3, one-way ANOVA). (D) Differentiated C2C12 myotubes were cultured in differentiation medium with or without EPS for 24 h in the presence or absence of 5 μM SB203580 (SB). Total RNA was isolated and Cxcl10 gene expression was determined by qPCR analysis. The graph represents the means ± SEM (**p < 0.01, N.S.; not significant, n = 4–6, one-way ANOVA).
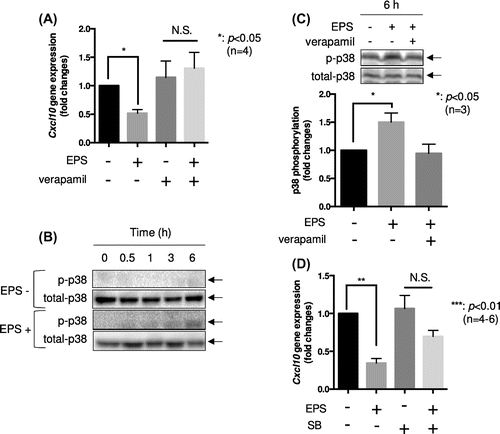
EPS-conditioned medium enhances MSS31 cell viability whereas recombinant CXCL10/IP-10 administration decreases it
To understand the potential roles of CXCL10 reduction by exercise, especially on exercise-dependent angiogenesis, we explored the effects of C2C12 conditioned medium after applying EPS (EPS conditioned medium; EPS-CM). As one of the major characteristics CXCL10 is its angiostatic function [Citation17], we hence analyzed the effects of EPS-CM on cell viability of the MSS31 vascular endothelial cell model. Prior to performing the experiments, we confirmed that CXCL10 exhibits the potential to reduce MSS31 cell viability. Upon increasing concentrations of CXCL10, MSS31 cell viability gradually decreased (Figure S4A). This could in part be explained by the induction of apoptosis, as the amounts of cleaved caspase-3 also increased (Figure S4B). To elucidate the effects of EPS-CM, the MSS31 cells were cultured under serum-free DMEM for 24 h, with subsequent change of the medium to either control-CM (Cont-CM), which was obtained from 24 h of C2C12 myotube culture supernatant, or EPS-CM. After 24 h, cell viability was monitored by using the MTT assay. As shown in Figure , the administration of EPS-CM significantly enhanced cell viability compared to that obtained with Cont-CM by 1.5-fold (p < 0.01, n = 3); this effect was partially abolished by the addition of recombinant CXCL10 (rCXCL10) (final concentration of total CXCL10; 125 pg/mL) (Figure ). Because the amounts of administrated rCXCL10 served to compensate for the EPS-dependent CXCL10 reduction, these results suggested that the decreases of CXCL10 in EPS-CM contributed, at least partially, to the enhancement of MSS31 cell viability.
Figure 4. EPS-conditioned medium enhances the viability of MSS31, an endothelial cell line, which is blocked by CXCL10 administration. Differentiated C2C12 myotubes were stimulated with or without EPS for 24 h, and the cell culture supernatants were collected. Conditioned medium (CM) of either EPS treated cells (EPS-CM) or non-treated cells (Cont-CM) was then added to quiescent MSS31 cell culture with or without recombinant CXCL10 (rCXCL10) (final concentration of total CXCL10; 125 pg/mL). MSS31 cell viability was evaluated with the MTT assay as described. The graph represents the means ± SEM (**p < 0.01, n = 3, N.S.; not significant, one-way ANOVA).
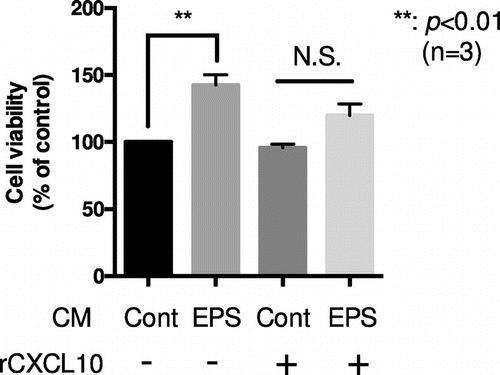
Discussion
Myokines serve as important mediators that transduce exercise signals in an autocrine, paracrine, or endocrine manner. Although the great majority of exercise-regulated myokines are exercise-inducible [Citation3,4], recent global RNA sequencing analysis suggests the reduction of some secreted factor transcripts in response to exercise in human skeletal muscle [Citation22]; however, little is known regarding such exercise-reducible myokines. To identify novel exercise-regulated myokines, we utilized our in vitro contractile system, consisting of C2C12 myotubes and EPS, which we have previously demonstrated as being suitable for studying exercise-dependent physiological changes [Citation20]. In the present study, we attempted to combine the in vitro contractile system and cytokine array analysis, and identified CXCL10 as a candidate novel myokine whose expression is suppressed by exercise.
Our animal experiments also suggested that moderate exercise in mice decreases Cxcl10 gene expression specifically in the soleus muscle (Figure (a)). Quad and EDL muscles in mice are largely composed of glycolytic fast-twitch (type II) fibers [Citation23], whereas the soleus muscle is predominantly composed of aerobic slow-twitch (type I) fibers [Citation24]. Hence, the decreases in Cxcl10 gene expression induced by our exercise condition appeared mainly in slow-twitch fibers. Furthermore, although we previously reported that total myosin heavy chain (MHC) amounts were not apparently affected by EPS in C2C12 myotubes; we note that the fast-type MHC decreased, whereas slow-type MHC increased by applying EPS for 24 h [Citation20].
By utilizing our in vitro contractile model, we also found that p38 MAPK activation by EPS participated, at least in a part, in the reduction of Cxcl10 gene expression. p38 MAPK signaling is known to play crucial roles in exercise-dependent mitochondrial biogenesis and PGC-1α transcription [Citation25,26]. Given that the Cxcl10 promoter contains responsive elements for interferon regulatory factor 1, nuclear factor-kappa B, and CCAAT-enhancer-binding proteins [Citation27,28], the transcription factor(s) that are activated by p38 MAPK and are responsible for Cxcl10 gene reduction are currently under investigation in our laboratory.
The microvascular system surrounding the skeletal muscle affords this tissue with a highly adaptive capacity. Endurance training is known to induce the numerical density of the capillaries, referred to as angiogenesis, which contributes to enhancing the performance of skeletal muscle by means such as nutrient delivery and O2 exchange [Citation29]. Although the precise mechanisms regarding how endurance training triggers this process have not been completely elucidated, several factors appear to be involved. In particular, VEGF is one such factor that functions through its specific receptors, VEGFR-1, -2, and -3, which are specifically expressed in endothelial cells [Citation30]. It has been demonstrated that the expression of VEGF in skeletal muscle was increased after endurance exercise [Citation31], as well as following resistance exercise [Citation32]. This exercise-induced increase in VEGF transcript levels appears to be specifically observed in type IIb, fast-twitch fibers, in Wistar rats [Citation33]. In addition, the expression levels of angiopoietin-1 (Ang-1) and Ang-2 in skeletal muscle are also implicated in exercise-dependent angiogenesis. These angiopoietins bind to their common receptor Tie-2 to regulate vascular development and remodeling; specifically, Ang-1 increases vessel stability, whereas Ang-2 decreases it [Citation34]. Notably, Lloyd et al. showed that the expression ratio of Ang-2/Ang-1 is increased after exercise [Citation35]; therefore, exercise-dependent expression control of angiopoietins in skeletal muscle likely also contributes to the remodeling of vessels.
In addition to these reported factors, our present study suggested that CXCL10 was also involved in exercise-dependent angiogenic control. Our initial observation that EPS-CM enhanced MSS31 cell viability might be predictable, because we previously reported that EPS-CM contained higher levels of angiogenic CXC chemokines, such as CXCL1 and CXCL5, compared to Cont-CM [Citation20]. Moreover, our preliminary experiments suggested that the levels of VEGF were also increased in EPS-CM (data not shown). However, notably, the viability-enhancing effect of EPS-CM on MSS31 cells was significantly reduced by the addition of rCXCL10, as this was apparently sufficient to compensate for the contraction-dependent reduction of endogenous CXCL10 (Figure ). Overall, although we cannot exclude the possibility that the induction of known angiogenic factors (i.e. CXCL1, CXCL5, VEGF, etc.) in EPS-CM contributes on the enhancement of MSS31 endothelial cell viability, our findings suggested that the reduction of CXCL10 in EPS-CM also plays a role in this enhancement.
Generally, the CXC chemokines have dual effects on angiogenesis, which depends on the presence or absence of the Glu–Leu–Arg (ELR) motif [Citation36]. In these two groups, ELR-negative chemokines, such as CXCL10, exhibit an angiostatic characteristic, whereas ELR-positive chemokines are angiogenic [Citation36]. Fall et al. previously reported that patients with juvenile dermatomyositis (DM), who lack angiogenic response in muscle tissue, displayed high expression of angiostatic ELR-negative CXC chemokines [Citation37]. Particularly, the gene expression of CXCL10 in juvenile DM muscle was increased by approximately 270-fold compared to that in normal muscle [Citation37], which is consistent with our model and further supports our speculation of a strong relationship between CXCL10 expression levels and angiogenesis.
Conclusion
In skeletal muscles, it has been well documented that slow-twitch muscles have greater capillary density compared to fast-twitch muscles [Citation38,39], with the enhanced angiogenesis mediated by endurance exercise apparently observed in soleus muscle, which is mainly composed of slow-twitch fibers [Citation40]. Moreover, whereas it has been widely accepted that VEGF transcription is increased by endurance exercise [Citation33], the angiogenic activities of VEGF can be antagonized by CXCL10 [Citation18]. Consistent with this, although further studies are required, our present results suggest that as a novel myokine, CXCL10 is exercise-reducible at least in slow-twitch muscle, and potentially controls endothelial cell viability. In turn, additional myokine studies will likely reveal the precise cell-cell communications between skeletal muscle cells and vascular endothelial cells that regulate exercise-dependent angiogenesis.
Author contributions
T.N. and Y.I. mainly constructed the concept for this research and designed the experiments. H.K., T.M., K.Y. and M.N. helped design and implement the study. Y.I., H.S., K.T. and T.N. performed the experiments, analyzed the data and contributed to the literature search. T.N. wrote the paper with critical revision for important intellectual content from H.K., T.M., K.Y. and M.N. All authors read and approved the manuscript.
Disclosure statement
No potential conflict of interest was reported by the authors.
Funding
This work was supported by a Grant-in-Aid for Scientific Research from the Japan Society for the Promotion of Science [grant number C-16K08077 to T.N.].
Supplemental data
Supplemental data for this article can be accessed https://doi.org/10.1080/09168451.2017.1411778.
Ishiuchi_Supplementary_revise_Final_version-h.pdf
Download PDF (319.8 KB)Acknowledgements
The authors are grateful to Dr. Anna Oue (Faculty of Food and Nutritional Sciences, Toyo University) for many helpful comments. The authors would like to thank Takuya Shibagaki, Chiho Miyashita and Narumi Komatsu (Faculty of Life Sciences, Toyo University) for technical assistance.
References
- Giudice J, Taylor JM. Muscle as a paracrine and endocrine organ. Curr Opin Pharmacol. 2017;34:49–55.10.1016/j.coph.2017.05.005
- Chan XC, McDermott JC, Siu KW. Identification of secreted proteins during skeletal muscle development. J Proteome Res. 2007;6:698–710.10.1021/pr060448 k
- Pedersen BK. Muscle as a secretory organ. Compr Physiol. 2013;3:1337–1362.
- Pedersen BK, Febbraio MA. Muscles, exercise and obesity: skeletal muscle as a secretory organ. Nat Rev Endocrinol. 2012;8:457–465.10.1038/nrendo.2012.49
- Louis E, Raue U, Yang Y, et al. Time course of proteolytic, cytokine, and myostatin gene expression after acute exercise in human skeletal muscle. J Appl Physiol. 1985;2007(103):1744–1751.
- Hittel DS, Axelson M, Sarna N, et al. Myostatin decreases with aerobic exercise and associates with insulin resistance. Med Sci Sports Exerc. 2010;42:2023–2029.10.1249/MSS.0b013e3181e0b9a8
- Goodyear LJ, Chang PY, Sherwood DJ, et al. Effects of exercise and insulin on mitogen-activated protein kinase signaling pathways in rat skeletal muscle. Am J Physiol Endocrinol Metab. 1996;271:E403–E408.
- Vavvas D, Apazidis A, Saha AK, et al. Contraction-induced changes in acetyl-CoA carboxylase and 5′-AMP-activated kinase in skeletal muscle. J Biol Chem. 1997;272:13255–13261.10.1074/jbc.272.20.13255
- Krook A, Widegren U, Jiang XJ, et al. Effects of exercise on mitogen- and stress-activated kinase signal transduction in human skeletal muscle. Am J Physiol Regul Integr Comp Physiol. 2000;279:R1716–R1721.
- Gehlert S, Bloch W, Suhr F. Ca2+-dependent regulations and signaling in skeletal muscle: from electro-mechanical coupling to adaptation. Int J Mol Sci. 2015;16:1066–1095.10.3390/ijms16011066
- Neville LF, Mathiak G, Bagasra O. The immunobiology of interferon-gamma inducible protein 10 kDa (IP-10): a novel, pleiotropic member of the C-X-C chemokine superfamily. Cytokine Growth Factor Rev. 1997;8:207–219.10.1016/S1359-6101(97)00015-4
- Liu M, Guo S, Stiles JK. The emerging role of CXCL10 in cancer (Review). Oncol Lett. 2011;2:583–589.
- van Marle G, Henry S, Todoruk T, et al. Human immunodeficiency virus type 1 Nef protein mediates neural cell death: a neurotoxic role for IP-10. Virology. 2004;329:302–318.10.1016/j.virol.2004.08.024
- Sui Y, Potula R, Dhillon N, et al. Neuronal apoptosis is mediated by CXCL10 overexpression in simian human immunodeficiency virus encephalitis. Am J Pathol. 2004;164:1557–1566.10.1016/S0002-9440(10)63714-5
- Strieter RM, Kunkel SL, Arenberg DA, et al. Interferon gamma-inducible protein 10 (IP-10), a member of the C-X-C chemokine family, is an inhibitor of angiogenesis. Biochem Biophys Res Commun. 1995;210:51–57.10.1006/bbrc.1995.1626
- Angiolillo AL, Sgadari C, Taub DD, et al. Human interferon-inducible protein 10 is a potent inhibitor of angiogenesis in vivo. J Exp Med. 1995;182:155–162.10.1084/jem.182.1.155
- Belperio JA, Keane MP, Arenberg DA, et al. CXC chemokines in angiogenesis. J Leukoc Biol. 2000;68:1–8.
- Moore BB, Arenberg DA, Stoy K, et al. Distinct CXC chemokines mediate tumorigenicity of prostate cancer cells. Am J Pathol. 1999;154:1503–1512.10.1016/S0002-9440(10)65404-1
- Yanai N, Satoh T, Obinata M. Endothelial cells create a hematopoietic inductive microenvironment preferential to erythropoiesis in the mouse spleen. Cell Struct Funct. 1991;16:87–93.10.1247/csf.16.87
- Nedachi T, Fujita H, Kanzaki M. Contractile C2C12 myotube model for studying exercise-inducible responses in skeletal muscle. Am J Physiol Endocrinol Metab. 2008;295:E1191–E1204.10.1152/ajpendo.90280.2008
- Fujita H, Nedachi T, Kanzaki M. Accelerated de novo sarcomere assembly by electric pulse stimulation in C2C12 myotubes. Exp Cell Res. 2007;313:1853–1865.10.1016/j.yexcr.2007.03.002
- Pourteymour S, Eckardt K, Holen T, et al. Global mRNA sequencing of human skeletal muscle: Search for novel exercise-regulated myokines. Mol Metab. 2017;6:352–365.10.1016/j.molmet.2017.01.007
- Klover P, Chen W, Zhu BM, Hennighausen L. Skeletal muscle growth and fiber composition in mice are regulated through the transcription factors STAT5a/b: linking growth hormone to the androgen receptor. FASEB J. 2009;23:3140–3148.10.1096/fj.08-128215
- Takekura H, Yoshioka T. Determination of metabolic profiles on single muscle fibres of different types. J Muscle Res Cell Motil. 1987;8:342–348.10.1007/BF01568890
- Sen P, Chakraborty PK, Raha S. p38 mitogen-activated protein kinase (p38MAPK) upregulates catalase levels in response to low dose H2O2 treatment through enhancement of mRNA stability. FEBS Lett. 2005;579:4402–4406.10.1016/j.febslet.2005.06.081
- Akimoto T, Pohnert SC, Li P, et al. Exercise stimulates Pgc-1alpha transcription in skeletal muscle through activation of the p38 MAPK pathway. J Biol Chem. 2005;280:19587–19593.10.1074/jbc.M408862200
- Liu M, Guo S, Hibbert JM, et al. CXCL10/IP-10 in infectious diseases pathogenesis and potential therapeutic implications. Cytokine Growth Factor Rev. 2011;22:121–130.
- Clarke DL, Clifford RL, Jindarat S, et al. TNFα and IFNγ synergistically enhance transcriptional activation of CXCL10 in human airway smooth muscle cells via STAT-1, NF-κB, and the transcriptional coactivator CREB-binding protein. J Biol Chem. 2010;285:29101–29110.10.1074/jbc.M109.099952
- Olfert IM, Baum O, Hellsten Y, et al. Advances and challenges in skeletal muscle angiogenesis. Am J Physiol Heart Circ Physiol. 2016;310:H326–H336.10.1152/ajpheart.00635.2015
- Bloor CM. Angiogenesis during exercise and training. Angiogenesis. 2005;8:263–271.10.1007/s10456-005-9013-x
- Richardson RS, Wagner H, Mudaliar SR, et al. Human VEGF gene expression in skeletal muscle: effect of acute normoxic and hypoxic exercise. Am J Physiol. 1999;277:H2247–H2252.
- Gavin TP, Drew JL, Kubik CJ, et al. Acute resistance exercise increases skeletal muscle angiogenic growth factor expression. Acta Physiol (Oxf). 2007;191:139–146.10.1111/aps.2007.191.issue-2
- Birot OJ, Koulmann N, Peinnequin A, Bigard XA. Exercise-induced expression of vascular endothelial growth factor mRNA in rat skeletal muscle is dependent on fibre type. J Physiol. 2003;552:213–221.10.1113/jphysiol.2003.043026
- Maisonpierre PC, Suri C, Jones PF, et al. Angiopoietin-2, a natural antagonist for Tie2 that disrupts in vivo angiogenesis. Science. 1997;277:55–60.10.1126/science.277.5322.55
- Lloyd PG, Prior BM, Yang HT, et al. Angiogenic growth factor expression in rat skeletal muscle in response to exercise training. Am J Physiol Heart Circ Physiol. 2003;284:H1668–H1678.10.1152/ajpheart.00743.2002
- Rosenkilde MM, Schwartz TW. The chemokine system – a major regulator of angiogenesis in health and disease. APMIS. 2004;112:481–95.10.1111/apm.2004.112.issue-7-8
- Fall N, Bove KE, Stringer K, et al. Association between lack of angiogenic response in muscle tissue and high expression of angiostatic ELR-negative CXC chemokines in patients with juvenile dermatomyositis: possible link to vasculopathy. Arthritis Rheum. 2005;52:3175–3180.10.1002/(ISSN)1529-0131
- Annex BH, Torgan CE, Lin P, et al. Induction and maintenance of increased VEGF protein by chronic motor nerve stimulation in skeletal muscle. Am J Physiol. 1998;274:H860–H867.
- Cherwek DH, Hopkins MB, Thompson MJ, et al. Fiber type-specific differential expression of angiogenic factors in response to chronic hindlimb ischemia. Am J Physiol Heart Circ Physiol. 2000;279:H932–H938.
- Kondo H, Fujino H, Murakami S, et al. Low-intensity running exercise enhances the capillary volume and pro-angiogenic factors in the soleus muscle of type 2 diabetic rats. Muscle Nerve. 2015;51:391–399.10.1002/mus.v51.3