Abstract
Our primary focus in this research was to identify and characterize its bioactive compounds for potential therapeutic use. Twenty-seven metabolites of Polygonum orientale were identified using LC-QTOF tandem mass spectrometry. Interestingly, P. orientale extracts included several highly oxygenated flavonoids were isolated from P. orientale by column chromatography. 13C NMR data of highly oxygenated flavonoids (1–7) are reported here for the first time. In addition, nitric oxide, 1,1-diphenyl-2-picrylhydrazyl, and water-soluble tetrazolium salt assays were carried out on the isolated compounds to investigate their anti-inflammatory, anti-oxidant, and neuroprotective activities, respectively. Compounds 1, 2, 3, 5, 7, and 8 significantly attenuated lipopolysaccharide-stimulated NO production in BV2 cells without affecting cell viability. Compounds 9–12 exhibited significant antioxidant activity, while compounds 8, 9, and 12 exhibited protective effects against glutamate-induced neurotoxicity in HT22 cells. Our results indicate that P. orientale is a promising source of natural agents for the potential treatment of inflammation and neurodegenerative diseases.
Graphical abstract
Chemical profile of Polygonum orientale.
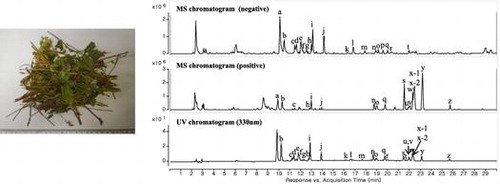
Plants produce a wide range of chemically and biologically diverse secondary metabolites, which continue to play a highly significant role in drug discovery and development [Citation1,2]. However, the identification of potentially bioactive constituents of natural products is challenging because of limitations in quantifying the compounds present in these products [Citation3]. The coupling of high-performance liquid chromatography (HPLC) with high-resolution mass spectrometry (MS), however, has produced a system that can identify the molecular weight and structure of unknown constituents of interesting mixtures [Citation4–6].
Polygonum orientale (Polygonaceae), an annual plant, has a cosmopolitan distribution and is cultivated domestically as well as grows at roadsides and on wastelands. P. orientale is also a well-known traditional Chinese medicine [Citation7,8], and the whole planth as been used in the treatment of conditions such as muscle injuries [Citation9], rheumatoid arthritis [Citation7,9], coronary heart disease [Citation10], and hypertension [Citation11]. Several studies have reported various pharmacological effects of P. orientale, including antimicrobial [Citation12], antimutagenic [Citation7], antithrombotic [Citation8], anti-inflammatory [Citation13], and anti-oxidative activities [Citation8,11]. Liang et al. investigated the anti-inflammatory effect of P. orientale extract (POE) in vivo, and found that it attenuated xylene-induced ear edema in mice and egg white-induced paw edema in rats. Moreover, POE inhibited cotton pellet-induced granuloma in a rat model of chronic inflammation [Citation13].
The main constituents of P. orientale are the flavonoids orientin, vitexin, cynaroside, and quercitrin, and lignan and saponin have also been reported as components [Citation10]. However, little has been reported to date on any direct correlation between the chemical composition and pharmacological effects of P. orientale. Therefore, we performed chemical profiling of P. orientale using liquid chromatography-quadrupole time-of-flight (LC-QTOF) MS. Biological activities of isolated compounds were investigated. Anti-inflammatory effects were tested via the measurement of the produced nitric oxide (NO) levels in lipopolysaccharide (LPS)-stimulated BV2 cells. Anti-oxidant activity was investigated by 1,1-diphenyl-2-picrylhydrazyl (DPPH) scavenging assay. DPPH assay is based on the principle that DPPH, free radical, is reduced and scavenged by accepting hydrogen atom from antioxidant. Neuroprotective activities against L-glutamate induced neurotoxicity in HT22 cells were evaluated with water-soluble tetrazolium salt (WST) assay. WST assay is used for measurement of cell viability by reductive reaction from succinate-tetrazolium reductase of mitochondria of live cells.
Materials and methods
General experimental procedures
NMR spectra were recorded on Bruker Avance DPX 300 and Bruker Avance III 600 spectrometers (Bruker, Billerica, MA, USA). ESI-MS spectra were measured using an Agilent 6530 Q-TOF mass spectrometer (Agilent, Santa Clara, CA, USA). Dimethyl sulfoxide (DMSO) and LPS were purchased from Sigma-Aldrich (St. Louis, MO, USA). Phosphoric acid was purchased from Wako Pure Chemical Industries, Ltd. (Osaka, Japan). Dulbecco’s modified Eagle’s medium (DMEM) was purchased from Hyclone Laboratories Inc. (Logan, UT, USA). Fetal bovine serum (FBS), penicillin-streptomycin, and trypsin-EDTA were purchased from Gibco Industries Inc. (Auckland, NZ). HPLC-grade water and acetonitrile were purchased from Fisher Scientific Korea Ltd. (Seoul, Korea). Chloroform-d, DMSO-d6, and methanol-d4 were purchased from Cambridge Isotope Laboratories, Inc. (Andover, MA, USA). A Griess reagent for measurement of NO production was manufactured with 1% sulfanilamide (Sigma-Aldrich, St. Louis, MO, USA), 0.1% naphtylethylenediamine dihydrochloride (Sigma-Aldrich, St. Louis, MO, USA), and 2% phosphoric acid (Wako Pure Chemical Industries, Ltd., Osaka, Japan). A WST kit (EZ-cytox) for cell viability assay was purchased from Daeil Lab Service Co., Ltd. (Seoul, Korea). Antioxidant activity was assessed by DPPH radical scavenging capacity. DPPH was purchased from Sigma-Aldrich and made with 15 μM DPPH solution in ethanol (EtOH). ESI-L Low Concentration Tuning Mix and Biopolymer Reference Mass Standard Kit for mass calibration were purchased from Agilent Technologies (Santa Clara, CA, USA).
Plant material
P. orientale was purchased from Kyungdong Oriental Herbal Market, Seoul, Korea and identified by Dr. Ki Yong Lee, a professor at the College of Pharmacy, Korea University (Sejong, Republic of Korea). The voucher specimen (KUP-HD053) has been deposited at the laboratory of pharmacognosy, College of Pharmacy, Korea University.
Extraction and isolation
Dried whole plants of P. orientale (1.6 kg) were cut into pieces and extracted three times (each for 1.5 h) with 8 L of 80% MeOH under ultrasonic conditions. After removing the solvent in vacuo, the obtained residue (46.6 g) was suspended in distilled water (1.5 L) and partitioned successively with n-hexane (1.5 L × 3), EtOAc (1.5 L × 3), and n-BuOH (1.5 L × 3) to yield ann-hexane fraction (2.6 g), an EtOAc fraction (4.6 g), and ann-BuOH fraction (18.4 g).
The n-hexane fraction was subjected to medium-pressure liquid chromatography (MPLC) using an Isolera One system (Biotage, Uppsala, Sweden) on a silica gel column (Biotage SNAPcartridge, KP-Sil 100 g) and eluted with an n-hexane/EtOAc gradient (95:5, 60:40) to yield 11 fractions (H1–H11). Compounds 1 (21.3 mg) and 2 (42.7 mg) were purified from H7 and H8 by recrystallization. Fraction H9 was re-chromatographed by MPLC using a Biotage SNAP cartridge, KP-Sil 10 g (CH2Cl2/MeOH, 100:0, 92:8), to afford 8 fractions (H9–1 to H9–8). Compound 3 (19.2 mg) was obtained from H9–3. The EtOAc fraction was subjected to silica gel (0.040–0.063 mm, 230–400 mesh)column chromatography (CC) and eluted with a gradient of n-hexane/EtOAc/MeOH (1:0:0, 20:1:0, 10:1:0, 5:1:0, 2:1:0, 1:1:0, 0:1:0, 0:20:1, 0:10:1, 0:5:1, 0:2:1,1 L of each solvent) to yield 20 fractions (EA1–EA20). Compounds 4 (6 mg), 5 (22 mg), 6 (5 mg), and 7 (7 mg) were purified by recrystallization from EA5, EA7, EA10, and EA11, respectively. EA15 was separated by Sephadex LH-20 (18–111 μm) CC (MeOH, isocratic, 2L) to yield 4 subfractions (EA15-1 to EA15-4). EA15-2 was purified by recrystallization to yield compound 8 (32 mg). EA16 was subjected to silica gel CC (CHCl3/MeOH/H2O, 50:4:0.1, 25:4:1, 15:4:0.4, 1 L of each solvent) to yield 9 fractions (EA16-1 to EA16-9). EA16-7 was further purified by recrystallization to yield compound 9 (57 mg). The n-BuOH fraction was subjected to adsorptive macroporous resin HP-20 (250–850 μm) CC and eluted successively with water, 20% aqueous MeOH, 40% aqueous MeOH, 60% aqueous MeOH, 80% aqueous MeOH, and 100% MeOH (2 L of each solvent) to yield 6 fractions (B1–B6). B3 and B4 were combined and further subjected to reversed-phase (RP)-C18 (30–50 μm) CC (MeOH/H2O, 1:4, 1:1.5, 2 L of each solvent) to afford 8 subfractions (B3-1 to B3-8). Compound 10 (47 mg) was obtained by the separation of B3-5 on a silica gel column (CH2Cl2/MeOH/H2O, 5:1:0.1, 4:1:0.1, 1 L of each solvent). Fraction B3-6 was subjected to silica gel CC (CHCl3/MeOH/H2O, 3.5:1:0.1, 3:1:0.1, 1 L of each solvent) to yield two subfractions (B3-6-1 and B3-6-2). B3-6-2 was recrystallized to provide compound 11 (142 mg). B3-8 was separated by silica gel CC (CHCl3/MeOH/H2O, 4.5:1:0.1, 3.5:1:0.1, 1 L of each solvent) to yield compound 12 (27 mg).
5,5′-dihydroxy-3,3′,4′,6,7,8-hexamethoxyflavone (digicitrin, 1):Yellow powder;C21H22O10; ESI-MS m/z435 [M + H]+; 1H and 13C NMR, see Table .
3,3′,5,6,7,8-hexamethoxy-4′,5′-methylenedioxyflavone (2): Pale yellow powder;C22H22O10; ESI-MS m/z447[M + H]+; 1H and 13C NMR, see Table .
3,3′,4′,5,5′,6,7,8-octamethoxyflavone (exoticin, 3):Yellow powder;C23H26O10; ESI-MS m/z463 [M + H]+; 1H and 13C NMR, see Table .
5-hydroxy-3,3′,6,7,8-pentamethoxy-4′,5′-methylenedioxyflavone(4): Yellow powder; C21H20O10; ESI-MS m/z433 [M + H]+; 1H and 13C NMR, see Table .
5,5′-dihydroxy-3,3′,4′,8-tetramethoxy-6,7-methylenedioxyflavone(5): Yellow powder; C20H18O10; ESI-MS 419 [M + H]+; 1H and 13C NMR, see Table .
3,3′,5,8-tetramethoxy-4′,5′,6,7-bis(methylenedioxy)flavone (6): Yellow powder; C21H18O10; ESI-MS m/z431[M + H]+; 1H and 13C NMR, see Table .
3,3′,4′,5,5′,8-hexamethoxy-6,7-methylenedioxyflavone (7): Yellow powder; C22H22O10; ESI-MS m/z447 [M + H]+; 1H and 13C NMR, see Table .
LC-Q-TOF MS/MS conditions
HPLC analysis was performed on an Agilent 1260 series system (Agilent, Santa Clara, CA, USA) with an auto-sampler, binary pump, degasser, and diode array detector. The P. orientale extracts were separated using a Kinetex C18 column (150 × 4.6 mm, 5 μm) with a C18 guard column (4.00 × 3.00 mm; Phenomenex, USA). The mobile phase consisted of water (solvent A) and acetonitrile (solvent B), each containing 0.1% formic acid. The elution gradient system was as follows: 15% B (0–5 min), and 90% B (5–30 min). The flow rate of the mobile phase was 0.6 mL/min and the injection volume was 10.0 μL. An Agilent 6530 Q-TOF mass spectrometer (Agilent, Santa Clara, CA, USA) was used for mass chromatography analysis with electrospray ionization (ESI) in the positive and negative mode. Before analysis, positive and negative ion mass calibration was performed using the tuning solution. Tuning solution was prepared by ESI-L Low Concentration Tuning Mix diluted with HPLC-grade water and acetonitrile. 0.1 mM HP-0321 which is included in the Biopolymer Reference Mass Standard Kit was mixed at positive tuning solution, additionally. The mass parameters were as follows: nebulizer pressure, 40 psi; capillary voltage, 4000 V; fragmentor voltage, 175 V; skimmer voltage, 65 V; drying gas temperature, 325 °C; flow rate of drying gas, 12.0 L/min. The MS/MS chromatogram of the EtOAc fraction and compounds from P. orientale was obtained using a collision energy of 10, 20, and 30 V. The mass scan range was set at 50–1700 m/z. Mass Hunter Workstation software LC/MS Data Acquisition for 6530 series Q-TOF (version B.05.00) was used to set all acquisition parameters for obtaining data.
NO production inhibition and cell viability assay
BV2 mouse microglia cells were obtained from the College of Pharmacy and Research Institute of Pharmaceutical Science, Seoul National University, Seoul, Korea. BV2 cells were grown in DMEM containing 10% FBS and 100 U/mL penicillin-streptomycin at 37 °C in a humidified atmosphere of 5% CO2 and 95% air. For NO production assay, cells were seeded at 2 × 10Citation5 cells/mL into 48-well plates for 24 h. Medium wasreplaced with DMEM without FBS and containing the experimental compounds for 1 h before exposure to 50 ng/mL of LPS. After 24 h, 100 μL of Griess reagent and 100 μL of supernatants in each well were reacted for 10 min at room temperature. Optical density (OD) was measured at 550 nm using ELISA reader, and the NO inhibition rate was calculated from the following: % NO inhibition = 100 × (OD of LPS treated cultures − OD of LPS-sample treated cultures)/(OD of LPS treated cultures − OD of control cultures).
100 μL of the remaining supernatants from the NO inhibition assay was used to determine cell viability by WST assay. 20 μL of WST solution was added to supernatants in a 96-well plate and incubated for 2 h. OD values were obtained at 450 nm. Percent cell viability was calculated as 100×(OD of LPS-sample treated cultures)/(OD of control cultures).
DPPH radical-scavenging assay
A DPPH assay was used to measurement of stable radical DPPH scavenging ability. Both a DPPH assay of the extract and compounds isolated from P. orientale and a time-based DPPH assay were performed as previously reported [Citation6].
Neuroprotective assay
Mouse hippocampal HT22 cells were grown in DMEM (Hyclone) containing 10% FBS and 100 U/mL penicillin-streptomycin at 37 °C in a humidified atmosphere of 5% CO2 and 95% air. HT22 cells were seeded at a density of 2 × 104 cells/mL in 96-well plates and cultured for 24 h. Various concentrations of compounds were used to pretreat cells for 1 h before the addition of 6 mM glutamate. Trolox (Sigma) was used as a positive control. After 24 h, cell viability was measured by WST assay. 10 μL of WST solution was added to each well, and cells were incubated for 2 h. OD values were obtained at 450 nm, and cell viability was calculated from the following equation: % protection = 100 × (OD of glutamate-sample treated cultures or OD of glutamate treated cultures)/(OD of control cultures).
Results and discussion
Chemical profile of P. orientale
We identified the metabolites contained in P. orientale extract using LC-QTOF-MS. The structures of the major mass chromatogram peaks were predicted using the UV spectrum, molecular weight, MS/MS fragmentation, and previously reported data (Figure , Table ). Twenty-seven peaks were identified from the mass chromatograms using electrospray ionization (ESI) in the positive and negative mode. Almost all peaks had maximum UV absorption (λmax) at 260 and 330 nm, indicating that the structures potentially were flavonoids. Peaks m, n, and q (vanicosides) were previously identified in other members of the Polygonum genus such as P. lapathifolium [Citation14], P. hydropiper [Citation15], and P. pensylvanicum [Citation16]. However, the present study is the first to identify vanicoside in P. orientale. Peaks p and s~z were found to be highly oxygenated flavonoids. Although flavonoids having eight oxygen functional groups (at C-3, 3′, 4′, 5, 5′, 6, 7 and 8) have previously been reported in P. orientale [Citation17], it has not previously been shown to contain highly oxygenated flavonoids.
Figure 1. MS and UV chromatograms of P. orientale extracts. (A) negative ionization mode; (B) positive ionization mode; (C) 330 nm.
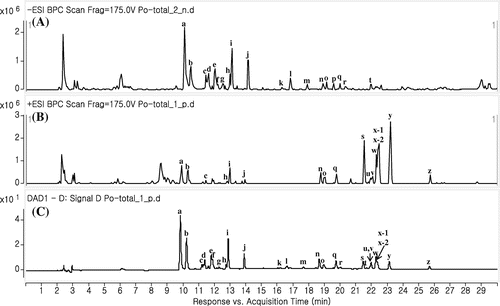
Table 1. Peak identification of P. orientale extracts on chromatographic profiles in Figure 1.
Isolation of compounds from P. orientale
Twelve flavonoids (1–12) were isolated from P. orientale extracts (Figure ), and were identified as 5,5′-dihydroxy-3,3′,4′,6,7,8-hexamethoxyflavone (digicitrin,1) [Citation17], 3,3′,5,6,7,8-hexamethoxy-4′,5′-methylenedioxyflavone (2) [Citation17], 3,3′,4′,5,5′,6,7,8-octamethoxyflavone (exoticin, 3) [Citation17], 5-hydroxy-3,3′,6,7,8-pentamethoxy-4′,5′-methylenedioxyflavone (4) [Citation17], 5,5′-dihydroxy-3,3′,4′,8-tetramethoxy-6,7-methylenedioxyflavone (5) [Citation17], 3,3′,5,8-tetramethoxy-4′,5′,6,7-bis(methylenedioxy)flavone (6) [Citation17], 3,3′,4′,5,5′,8-hexamethoxy-6,7-methylenedioxyflavone (7) [Citation17], kaempferol 3-O-α-L-rhamnopyranoside (afzelin, 8) [Citation18], quercetin 3-O-α-L-rhamnopyranoside (quercitrin, 9) [Citation19], luteolin 8-C-β-D-glucopyranoside (orientin, 10) [Citation20], luteolin 6-C-β-D-glucopyranoside (isoorientin, 11) [Citation20], and luteolin 7-O-β-D-glucopyranoside (cynaroside, 12) [Citation21] by comparing their physical and spectroscopic data with those reported previously. Interestingly, all proton positions of compounds 1–7 structures are substituted oxygen molecules, producing the unusual compounds that are highly oxygenated flavonoids. The present study is the first to report 13C- NMR data for these compounds. 1H- and 13C NMR data of compounds 1–7 were displayed in Table .
Table 2. 1H- and 13C NMR spectroscopic data for compounds 1–7 (δ in ppm).
Biological activities of isolated compounds
We evaluated the inhibitory effect on NO production of an 80% MeOH extract of P. orientale, as well as of each fraction, using lipopolysaccharide (LPS)-stimulated BV2 cells. The ethyl acetate (EtOAc) and n-butanol (BuOH) fractions significantly inhibited NO production and did not influence cell viability at a concentration range of 10–100 μg/ml (S1). Compounds 1–12 of P. orientale were subjected to a Griess assay at concentrations of 1, 5, 15, 30, and 50 μM (Table ), and the NO production inhibitory effect was demonstrated by the relative nitric oxide inhibition rate. Among the compounds, 1, 2, 3, 5, 7, and 8 exhibited the highest NO scavenging effect on LPS-induced BV2 cells (Table ). Cell viability was not affected by the compounds at a concentration of 50 μM (S2). Compound 8 (afzelin) was shown in a previous study to inhibit NO production in LPS-stimulated RAW 264.7 cells with an IC50 value of 42.8 μg/mL [Citation22]. Compounds 1, 2, 3, 5, and 7 thus showed potential as novel candidates with NO inhibitory activity. The structure of these five compounds is related to their anti-inflammatory effect, as flavonoids having a 3′,4′-hydroxyl substituent on the B ring or with additional methoxy substituent have been shown to possess high anti-inflammatory activity [Citation23] (see Figure ).
Table 3. Effect of isolated compounds on NO production in BV2 cells, DPPH assay, and glutamate-induced toxicity in HT22 cells.
The 80% MeOH extract and fractions of P. orientale were screened to identify free radical scavenging activity using a DPPH radical-scavenging assay at concentrations of 5, 25, 50, and 250 μg/mL. The EtOAc, n-BuOH, and water fractions showed a significant antioxidant effect (S3). In the DPPH radical-scavenging assay, compounds 9–12 showed strong antioxidant activity with IC50values of 11.5 ± 0.4, 9.7 ± 0.9, 8.6 ± 0.1, and 14.5 ± 0.6 μm, respectively, compared with that of curcumin. DPPH radical-scavenging activity is dependent on the position of hydroxyl groups in the aromatic ring [Citation24]. Compounds 9–12, with hydroxyl groups in the ortho position in the aromatic ring, exhibited a strong antioxidant activity.
The neuroprotective activity of the 80% MeOH extract, each fraction, and isolated compounds 1–12 of P. orientale was evaluated in glutamate-induced HT22 cells. Neither the extract nor the fractions showed a neuroprotective effect or influenced cell viability at 50 and 100 μg/mL (S4). However, compounds 8, 9, and 12 significantly attenuated the induced glutamate neurotoxicity (Table ). The result exhibited that O-glycoside flavonoids have more neuroprotective activity than C-glycoside flavonoids. Compound 9 has previously been shown to exert a significant neuroprotective effect in HT22 cells induced by glutamate [Citation25].
In conclusion, our present study was certified that LC-QTOF-MS analysis is a useful technology to identify the metabolites of plants. The twelve compounds were isolated from whole plant of P. orientale. All of them were flavonoids, some of them had a highly oxygenated structure uncommonly. The isolated constituents of P. orientale exhibited anti-inflammatory, anti-oxidant, and neuroprotective activities. Taken together, our findings indicate a potential role for isolated compounds of P. orientale as novel candidates for the prevention and/or treatment of inflammatory and neurodegenerative diseases.
Author contributions
H. Shin, Y. Park, Y.H. Jeon, X. Yan, and K. Y. Lee conceived and designed the experiments. H. Shin, Y. Park, and X. Yan performed all experiments and analyzed all the data. H. Shin, Y.H. Jeon, and K. Y. Lee wrote the manuscript. All the authors reviewed and approved the final manuscript.
Disclosure statement
No potential conflict of interest was reported by the authors.
Funding
This work was supported by a National Research Foundation of Korea grant funded by the Korean Government [NRF-2014R1A1A1003605], [NRF-2014R1A4A1007304], [NRF-2017R1A2B4003403].
Supplemental data
Supplemental material for this paper is available at https://doi.org/10.1080/09168451.2017.1415124.
Suppl_revised.pdf
Download PDF (1.9 MB)References
- Newman DJ, Cragg GM. Natural products as sources of new drugs over the 30 years from 1981 to 2010. J Nat Prod. 2012;75:311–335.10.1021/np200906s
- Saito K, Matsuda F. Metabolomics for functional genomics, systems biology, and biotechnology. Annu Rev Plant Biol. 2010;61:463–489.10.1146/annurev.arplant.043008.092035
- Qu F-N, Qi L-W, Wei Y-J, et al. Multiple target cell extraction and LC-MS analysis for predicting bioactive components in Radix Salviae miltiorrhizae. Biol Pharm Bull. 2008;31:501–506.10.1248/bpb.31.501
- Li D, Wang T, Guo Y, et al. Online screening of nitric oxide scavengers in natural products using high performance liquid chromatography coupled with tandem diode array and fluorescence detection. J Chromatogr A. 2015;1425:106–115.10.1016/j.chroma.2015.10.095
- Nuengchamnong N, Krittasilp K, Ingkaninan K. Characterisation of phenolic antioxidants in aqueous extract of Orthosiphon grandiflorus tea by LC–ESI-MS/MS coupled to DPPH assay. Food Chem. 2011;127:1287–1293.10.1016/j.foodchem.2011.01.085
- Shin H, Chung H, Park B, et al. Identification of antioxidative constituents from Polygonum aviculare using LC-MS coupled with DPPH assay. Nat Prod Sci. 2016;22:64–69.10.20307/nps.2016.22.1.64
- Liao SG, Li YT, Zhang LJ, et al. UPLC–PDA–ESI–MS/MS analysis of compounds extracted by cardiac h9c2 cell from Polygonum orientale. Phytochem Anal. 2013;24:25–35.10.1002/pca.v24.1
- Jiang X, Chen X, Wei Y. Free radical-scavenging activity and flavonoid contents of Polygonum orientale leaf, stem, and seed extracts. Arch Biol Sci. 2009;61:79–83.10.2298/ABS0901079 J
- Xiang M-x, Xu L, Liu Y, et al. In vitro evaluation of the effects of Polygonum orientale L. on proliferation and differentiation of osteoblastic MC3T3-E1 cell. J Med Plant Res. 2011;5:231–236.
- Huang Y, Chen H, He F, et al. Simultaneous determination of human plasma protein binding of bioactive flavonoids in Polygonum orientale by equilibrium dialysis combined with UPLC–MS/MS. J Pharm Anal. 2013;3:376–381.10.1016/j.jpha.2013.04.001
- Wei Y, Chen X, Jiang X, et al. Determination of taxifolin in Polygonum orientale and study on its antioxidant activity. J Food Compost Anal. 2009;22:154–157.10.1016/j.jfca.2008.08.006
- Cai J, Xie S, Feng J, et al. Protective effect of Polygonum orientale L. Extracts against Clavibater michiganense subsp. sepedonicum, the causal agent of bacterial ring rot of potato. PLoS ONE. 2013;8:e68480.10.1371/journal.pone.0068480
- Liang S-L, Liang Q, Zhong W-H, et al. Anti-inflammatory and analgesic effects of Polygonum orientale extract. Zhong Cao Yao. 2014;45:3131–3135.
- Takasaki M, Konoshima T, Kuroki S, et al. Cancer chemopreventive activity of phenylpropanoid esters of sucrose, vanicoside B and lapathoside A, from Polygonum lapathifolium. Cancer Lett. 2001;173:133–138.10.1016/S0304-3835(01)00670-X
- Van Kiem P, Nhiem NX, Cuong NX, et al. New phenylpropanoid esters of sucrose from Polygonum hydropiper and their antioxidant activity. Arch Pharm Res. 2008;31:1477–1482.10.1007/s12272-001-2133-y
- Zimmermann ML, Sneden AT. Vanicosides A and B, protein kinase C inhibitors from Polygonum pensylvanicum. J Nat Prod. 1994;57:236–242.10.1021/np50104a007
- Kuroyanagi M, Fukushima S. Highly oxygenated flavonoids from Polygonum orientale. Chem Pharm Bull. 1982;30:1163–1168.10.1248/cpb.30.1163
- Chen C-Y, Hsieh S-L, Hsieh M-M, et al. Substituent chemical shift of rhamnosides from the stems of Cinnamomum osmophleum. Chin Pharm J. 2004;56:141–146.
- Markham KR, Ternai B, Stanley R, et al. Carbon-13 NMR studies of flavonoids—III: naturally occurring flavonoid glycosides and their acylated derivatives. Tetrahedron. 1978;34:1389–1397.10.1016/0040-4020(78)88336-7
- Kato T, Morita Y. C-glycosylflavones with acetyl substitution from Rumex acetosa L. Chem Pharm Bull. 1990;38:2277–2280.10.1248/cpb.38.2277
- Lu Y, Foo LY. Flavonoid and phenolic glycosides from Salvia officinalis. Phytochemistry. 2000;55:263–267.10.1016/S0031-9422(00)00309-5
- Kim SK, Kim HJ, Choi SE, et al. Anti-oxidative and inhibitory activities on nitric oxide (NO) and prostaglandin E 2 (COX-2) production of flavonoids from seeds of Prunus tomentosa Thunberg. Arch Pharm Res. 2008;31:424–428.10.1007/s12272-001-1174-9
- Chen X-M, Tait AR, Kitts DD. Flavonoid composition of orange peel and its association with antioxidant and anti-inflammatory activities. Food Chem. 2017;218:15–21.10.1016/j.foodchem.2016.09.016
- Sroka Z, Cisowski W. Hydrogen peroxide scavenging, antioxidant and anti-radical activity of some phenolic acids. Food Chem Toxicol. 2003;41:753–758.10.1016/S0278-6915(02)00329-0
- Lee KY, Hwang L, Jeong EJ, et al. Effect of neuroprotective flavonoids of Agrimonia eupatoria on glutamate-induced oxidative injury to HT22 hippocampal cells. Bioscience, Biotechnology, and Biochemistry. 2010;74:1704–1706.10.1271/bbb.100200