Abstract
Tyrosinase is the key enzyme that controls melanin formation in the human skin. We performed a screening of 96 extracts of mushroom cultures and fruiting bodies for examining their inhibitory activity against mushroom tyrosinase. The ethyl acetate extracts of culture filtrate of Neolentinus lepideus exhibited the strongest inhibitory activity. The active compounds 1 and 2 were purified by repeated chromatographic separations from the extract. On the basis of spectroscopic analyses, 1 and 2 were identified to be 1,3-dihydroisobenzofuran-4,5,7-triol and 5-methoxy-1,3-dihydroisobenzofuran-4,7-diol, respectively. Lineweaver-Burk plot of the enzyme reaction in the presence of 1 indicated that 1 was a potent competitive inhibitor. The respective IC50 values of 1 and 2 were 173 and 263 μg/mL. Compound 1 at 15 μg/mL suppressed melanin accumulation stimulated by α-MSH in the murine melanoma B16 cells, as well as the induced accumulation of both tyrosinase transcript and protein without inhibiting cell proliferation.
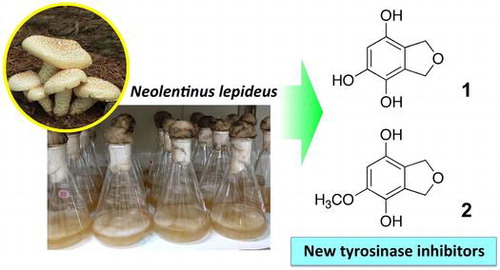
We identified new tyrosinase inhibitors in the extract of culture filtrate of Neolentinus lepideus.
In kingdom Fungi, the species that produce visible fruiting bodies are regarded as mushrooms. Numerous bioactive compounds, such as medicines, pesticides, and neuroactive substances, have been identified from mushrooms. However, mushrooms have not been extensively investigated phylogenetically, physiologically, and chemically. Thus, mushrooms are unique and promising bioresources that should be investigated further.
Our work is focused on the inhibitors of melanin biosynthesis. Melanin is a pigment that is produced and deposited in the melanocytes present in the stratum basale of the epidermal layer of skin. The inhibition of melanin deposition is important for maintaining healthy skin, since the formation of mottles and freckles is caused by hypermelanogenesis. Melanin is biosynthesized from l-tyrosine. Tyrosinase (EC 1.14.18.1) catalyzes the conversion of l-tyrosine to l-3,4-dihydroxyphenylalanine (l-DOPA) and from l-DOPA to l-dopaquinone, and is the rate-limiting enzyme in this pathway [Citation1]. In addition to tyrosinase, tyrosinase-related proteins 1 and 2 (TRP-1 and TRP-2) have been shown to be involved in melanin biosynthesis. TRP-2 serves as the dopachrome tautomerase that catalyzes the tautomeric conversion of dopachrome to 5,6-dihydroxyindole-2-carboxylic acid (DHICA) [Citation2], and TRP-1 catalyzes the oxidation of DHICA to indole-5,6-hydroquinone-2-carboxylic acid [Citation3].
Melanocytes are regulated by various endogenous and exogenous factors from the environment and from neighboring cells in the skin, including UV light, melanocyte stimulating hormone (MSH), agouti signal protein, endothelin 1, dickkopf 1, a wide variety of growth factors, and cytokines [Citation4,5]. Melanogenesis-associated transcription factor (MiTF) is suggested to play a pivotal role in the regulation of tyrosinase gene (TYR) expression downstream of the intrinsic and environment-induced signaling pathways. MiTF is also involved in the proliferation and survival of melanocytes [Citation6,7].
The compounds currently used for preventing melanin deposition are exclusively inhibitors of tyrosinase, such as hydroquinone [Citation8,9], arbutin [Citation10], kojic acid (5-hydroxy-2-hydroxymethyl-4-pyrone [Citation11], and glutathione [Citation12]. Recently, rhododendrol (4-(4-hydroxyphenyl)-2-butanol), which is an effective inhibitor of tyrosinase and used as an ingredient in cosmetics, was reported to cause patches of leucoderma on the skin of the face, neck, and hands because of its toxicity to melanocytes [Citation13–15]. Thus, it is undeniable that effective and safe tyrosinase inhibitors are required.
The Fungus/Mushroom Resource and Research Center (FMRC), Tottori University possesses a collection of mushroom strains (8387 strains belonging to 1400 species, as on March 2017), which is one of the largest mushroom collections in the world. Recently, the analyses of bioactive compounds present in these strains have been initiated, which have resulted in the identification of several antimicrobial compounds [Citation16,17]. We performed a screening for the extracts derived from the fruiting bodies and liquid cultures of the FMRC-preserved edible mushroom strains, in an attempt to identify novel tyrosinase inhibitors. Edible mushrooms are considered valuable sources for identifying novel bioactive compounds, which may find applications in functional foods and/or cosmetics, since their constituents are expected to exhibit no-to-low toxicity.
Neolentinus lepideus (Fr.) Redhead & Ginns (formerly Lentinus lepideus (Fr.) Fr.) is a wood-decaying mushroom belonging to the family Gloeophyllaceae and produces brown rot. It is one of the popular edible mushrooms in East Asian countries, namely China, Korea, and Japan. The cultivation of N. lepideus was first reported by Ogiyama [Citation18]. This mushroom is commercially available in Korea. The screening indicated that the extract of culture filtrate of N. lepideus effectively inhibited tyrosinase activity. Thus, we identified the active compounds in the extract of N. lepideus culture filtrate and characterized them using enzyme- and cell-based inhibition assays.
Materials and methods
General procedures
1H- and 13C-NMR spectra and 2D spectra (COSY, HMQC, and HMBC) were recorded using an Avance II instrument (Bruker, Billerica, MA, USA). High-resolution mass spectra were measured using an Exactive mass spectrometer (Thermo Fisher Scientific, Waltham, MA, USA), and ESI MS were recorded by a Quattro Micro API mass spectrometer (Waters, Milford, MA, USA) connected to an Acquity UPLC (Waters). Preparative HPLC was performed with a 10A HPLC system (Shimadzu, Kyoto, Japan). Enzyme activity was determined with an Infinite 200 PRO microplate reader (TECAN, Männedorf, Switzerland).
Reagents
Dulbecco’s modified Eagle’s medium (DMEM), synthetic melanin, tyrosinase from mushroom, and recombinant tyrosinase were obtained from Sigma-Aldrich (Tokyo, Japan). α-MSH and l-DOPA were purchased from Wako Pure Chemical Industries (Osaka, Japan). Phosphate-buffered saline (PBS) and trypsin used for cell culturing were obtained from Life Technologies (Carlsbad, CA, USA). All other reagents used were of the highest commercially available purity.
Tyrosinase inhibitory activity assay
Tyrosinase activity was determined using the method described by Shimizu et al. [Citation19] and Arung et al. [Citation20] with slight modifications. In brief, a mixture consisting of 133 μL of phosphate buffer (0.1 M, pH 6.5), 47 μL of 2.5 mM l-DOPA, and 8 μL of sample solution in DMSO was preincubated at 25 °C for 15 min in a well of a microplate. Next, for initiating the reaction, 12 μL of tyrosinase (extracted from mushroom, 6.4 units) in phosphate buffer (0.1 M, pH 6.5) was added to the mixture. For human tyrosinase (recombinant) reaction, the reaction mixture was downsized by half, and 6 μL of tyrosinase (5 units) was added to the mixture. The reaction mixture was incubated at 25 °C for 5 min, and absorbance was recorded at 475 nm. The inhibition rate was calculated according to the following equation:
where A is the absorbance at 475 nm for the complete reaction; B and C are the values of the reaction mixtures without tyrosinase and the sample solution, respectively; and D is the value of the reaction mixture without both tyrosinase and the sample solution. In the reactions without tyrosinase and the samples, phosphate buffer and DMSO, respectively, were added.
Liquid culture of N. lepideus
Neoentinu lepideus (TUFC31432) is the preserved strain of FMRC, Tottori University. The strain was cultured without shaking at 25 °C for 47 days in the dark in 500-mL conical flasks containing a liquid medium (200 mL/flask, 25 flasks) consisting of glucose (30 g/L), peptone (3 g/L), the extract from 50 g/L malt, and tap water.
Purification of active compounds
Culture filtrate (5 L) of N. lepideus was extracted with ethyl acetate (1.7 L) three times. The extract was dried over Na2SO4 overnight and evaporated to dryness. The extract (1.14 g) was subjected to column chromatography using silica gel (100 g, Daisogel IR-60-63/210, Daiso, Osaka, Japan). The column was eluted with mixtures of acetone and hexane. The concentration of acetone was increased from 0 to 100% in increments of 10%, with the volume of each fraction being 1 L. Activity was detected in the 30 and 40% acetone fractions.
The 40% acetone fraction (60 mg) was subjected to silica gel column chromatography (6 g, Daisogel IR-60-63/210). The column was eluted with mixtures of ethyl acetate and hexane. The concentration of acetone was increased from 40 to 100% in increments of 10%, with the volume of each mixture being 60 mL. The eluate for each ethyl acetate concentration was collected as three fractions, with the volume of one fraction being 20 mL. Activity was detected in the 50%-1 fraction (first fraction in 50% ethyl acetate). This fraction was concentrated to afford 1.
Compound 1 (9.1 mg). Negative ESI MS: m/z 167 [M−H]−; Positive ESI MS: m/z 169 [M+H]+; HR ESI MS: m/z 169.0495 [M+H]+ (calc. for C8H9O4: 169.0495), 191.0316 [M+Na]+ (calc. for C8H8O4Na: 191.0315), 167.0345 [M–H]− (calc. for C8H7O4: 167.0344). NMR data are shown in Table .
Table 1. 1H (600 MHz) and 13C (150 MHz) NMR data for 1 and 2 in methanol-d4
The 30% acetone fraction (140 mg), obtained after the first column chromatography, was also subjected to the second round of silica gel column chromatography (14 g, Daisogel IR-60-63/210). The column was eluted with mixtures of ethyl acetate and hexane, and the concentration of ethyl acetate was increased from 40 to 100% in increments of 10%. The volume of each mixture was 140 mL. The eluate for each ethyl acetate concentration was collected as three fractions, with the volume of one fraction being 47 mL. The 40%-3 fraction (25 mg) was subjected to the third round of silica gel column chromatography (2.5 g, Daisogel IR-60-63/210). The column was eluted with mixtures of methanol and chloroform. The column was eluted with 0, 2, 5, 10, and 100% methanol. The volume of each mixture was 25 mL. The eluate for each methanol concentration was collected as three fractions, with the volume of one fraction being 8.3 mL. The 2%-2 (3.5 mg) fraction was subjected to preparative HPLC. The HPLC conditions were as follows: column, Cosmosil 5C18-MS-II (10 × 250 mm, Nacalai Tesque, Kyoto, Japan); temperature, 40 °C; solvent, 18% acetonitrile in water; flow rate, 3 mL/min; detection, 254 nm. The peak with retention time of 8.2 min was denoted as compound 2. The fractions containing 2 were concentrated to afford 2.
Compound 2 (3.2 mg). Negative ESI MS: m/z 181 [M−H]−; positive ESI MS: m/z 183 [M+H]+; HR ESI MS: m/z 183.0629 [M+H]+ (calc. for C9H11O4: 183.0652), 205.0473 [M+Na]+ (calc. for C9O10O4Na: 205.0471), 181.0500 [M−H]− (calc. for C9H9O4: 181.0501). NMR data are shown in Table .
Cell culture
The B16 melanoma (mouse melanin-producing melanoma, RCB1283) cells were purchased from Riken Bio Resource Center (Tsukuba, Japan). The B16 cells were cultured in DMEM supplemented with 10% (v/v) fetal bovine serum (Biowest, Paris, France) and 1% (v/v) penicillin/streptomycin (Wako Pure Chemical Industry) in air containing 5% CO2 at 37 °C. During incubation, the medium was changed every 3 days.
Assay for evaluating inhibition of melanin synthesis using a cell culture system
Compounds 1 and 2 were dissolved in minute volumes of ethanol and diluted with distilled water. Melanin content was determined as follows: Briefly, the B16 cells were cultured in the presence of 10 nM α-MSH with 5 × 104 cells/plate in a 35 × 10-mm cell culture plate. After 24 h, the solutions of 1 and 2 were added to the medium. The final concentrations of 1 and 2 were 0, 1, 3, and 15 μg/mL. The cells were cultured for an additional 48 h. Then, the cells were washed with PBS and harvested by trypsinization. The cell pellets were treated with 1 mL of 1 M NaOH at 80 °C for 1 h. The absorbance of cell extracts was determined at 475 nm using a Shimadzu spectrophotometer. Synthetic melanin was used as the standard. Cell viability was analyzed by a TC20 cell counter (Bio-Rad Laboratories, Hercules, CA, USA).
qRT-PCR
Total RNA was prepared from the cultured cells using Sephasol-RNA1 (Nacalai Tesque), and used to synthesize cDNA by the PrimeScript RT Reagent Kit with gDNA Eraser (Takara Bio, Otsu, Japan). The primer pairs for qPCR were designed using the GENETYX software (GENETYX, Tokyo, Japan) to yield 18-24-nucleotide sequences with amplification products of approximately 100 bp (Supplementary Table 1). A CFX Connect Real-Time System (Bio-Rad) with SYBR Premix Ex Taq (Takara Bio) was used to perform qPCR. GAPDH was used as the internal standard. qPCR experiments were repeated at least three times for each cDNA prepared from three preparations of cells.
Western blot analysis
After reaching confluence, the cells were harvested and lysed using the passive lysis buffer (Promega, Madison, WI, USA) at 4 °C for 30 min. The cell lysate was centrifuged at 10,000× g for 10 min at 4 °C, and the supernatant was used as the crude protein for western blot analysis. The protein extract was subjected to SDS-polyacrylamide gel electrophoresis. We used a precast slab gel (e-PAGEL, model E-R520L; ATTO, Tokyo, Japan) for performing electrophoresis through a 5–20% (w/w) linear gradient of polyacrylamide in the presence of SDS. After electrophoresis, the proteins were transferred onto a polyvinylidene difluoride (PVDF) membrane (Bio-Rad) in a Trans-Blot SD semi-dry electrophoretic transfer cell (Bio-Rad). Non-specific binding was blocked with 5% (w/v) non-fat dry milk in PBS/Tween 20 buffer. The PVDF membranes were probed with anti-tyrosinase (ab180753, Abcam, Cambridge, MA, USA), anti-MiTF (ab20663, Abcam), anti-TRP1 (ab83774, Abcam), anti-TRP2 (ab74073, Abcam), and anti-GAPDH (ab9485, Abcam) antibodies individually. Immunodetection was performed using the anti-rabbit IgG secondary antibody (ab6721, Abcam) coupled to the tetramethylbenzidine-stabilized substrate for horseradish peroxidase (Promega, Tokyo, Japan), in accordance with the manufacturer’s instructions. A protein ladder (Amersham ECL Rainbow Marker-Full Range; GE Healthcare, Minneapolis, MN, USA) was used to determine the molecular mass.
Statistical analyses
B16 cell viability and melanin concentration were analyzed using one-way ANOVA with Tukey’s multiple comparison test. The analyses were performed using GraphPad Prism for Windows, version 5.03 (GraphPad Software, La Jolla, CA). All data were expressed as mean ± standard deviation, and the differences were considered to be statistically significant at p < 0.05.
Results
Isolation of tyrosinase inhibitors from N. lepideus
We examined inhibitory activity for the mushroom tyrosinase in extracts of mycelia and culture filtrates of 32 edible mushrooms, and extracts of fruiting bodies of 9 edible mushrooms. The extract of culture filtrate of N. lepideus exhibited the strongest activity with the inhibition rate of 72% at 1000 μg/mL. The inhibition rate of arbutin at 1000 μg/mL was 24%. To isolate tyrosinase inhibitors from the culture filtrate, we cultured N. lepideus in a liquid malt medium for 47 days. The culture filtrate was extracted and subjected to activity-guided fractionation by column chromatography. Finally, we obtained two active compounds 1 and 2.
HR ESI MS data of 1 showed the peak of protonated molecule at m/z 169.0495, establishing the molecular formula as C8H8O4, and thus, the hydrogen deficiency index of 1 was 5. Two methylene groups, represented by the signals at δH 4.96 and 4.99 in the 1H NMR spectrum, were considered to be connected via an oxygen atom on the basis of their chemical shifts and correlation in the H–H COSY spectrum (Figure ). A signal at δH 6.25 in the 1H NMR spectrum and six signals at δC 146.9, 145.0, 133.2, 128.3, 117.1, and 103.9 in the 13C NMR spectrum indicated the presence of a five-substituted benzene ring. The molecular formula suggested the presence of three hydroxyl groups that were considered linked to the benzene ring, based on the low magnetic field shifts of three carbon signals in the benzene ring (δC 146.9, 145.0, and 133.2). The positions of substituents on the benzene ring were determined by the HMBC correlations as shown in Figure , and 1 was identified to be 1,3-dihydroisobenzofuran-4,5,7-triol (Figure ). To the best of our knowledge, this compound has not been described in the literature to date.
Figure 1. HMBC and COSY correlations of 1 and 2. Arrows indicates the correlation from protons to carbons observed by HMBC, and bold lines indicate the correlations observed by COSY.
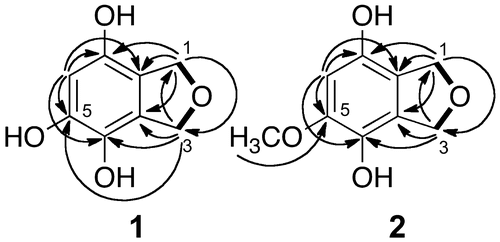
The molecular formula of 2 was determined to be C9H10O4, on the basis of the peak of the protonated molecule at m/z 183.0629 in HR ESI MS. The hydrogen deficiency index was 5. The 1H and 13C NMR spectra of 2 were similar to those of 1, except for the presence of a methoxy group (δH 3.80 and δC 56.8) in 2 (Table ). The 1H and 13C NMR spectra indicated the presence of two methylene groups connected by an oxygen atom and a benzene ring. Considering the molecular formula, the presence of two hydroxy groups was indicated. In the HMBC spectrum (Figure ), the methylene protons at positions 1 and 3 showed correlations with carbons at 3a, 4, 7a, and 7 positions, but not to the carbon bearing the methoxy group. Thus, the methoxy group was located at position 5, as shown in Figure . On the basis of these data, compound 2 was identified to be 5-methoxy-1,3-dihydroisobenzofuran-4,7-diol (Figure ). This compound also has not been described in the literature to date. The chemical structures of 1 and 2 are shown in Figure .
We developed an MRM method to detect 1 and 2, and analyzed their concentrations in the fruiting bodies collected from fields in Japan. The concentrations of 1 and 2 were below the detection limits (Supplementary materials).
Inhibition of tyrosinases by 1 and 2
We performed reactions using various concentrations of 1 and 2, as well as the known tyrosinase inhibitors hydroquinone and arbutin, with mushroom tyrosinase (Figure ). The respective IC50 values of 1, 2, hydroquinone, and arbutin were 173 μg/mL (1 mM), 263 μg/mL (1.4 mM), 1970 μg/mL (7.80 mM), and 19,000 μg/mL (69.9 mM). Accordingly, 1 and 2 showed inhibitory activity against mushroom tyrosinase at concentrations lower than those of hydroquinone and arbutin. The concentrations of hydroquinone and arbutin in cosmetic formulations are in the range from 10 to 50 mg/mL [Citation21,22].
Figure 3. Inhibition of tyrosinase by compound 1 (closed circles), 2 (closed triangles), hydroquinone (open squares), and arbutin (open diamonds).
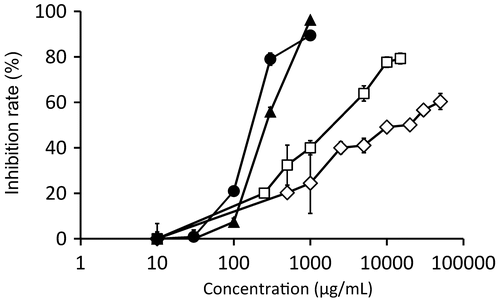
Next, we performed enzyme reactions in the presence of 1 at 100 and 300 μg/mL to determine the mode of inhibition. In the Lineweaver-Burk plot (Figure ), the regression lines crossed on the y-axis, indicating competitive inhibition.
Figure 4. Lineweaver-Burk plot for the enzyme reactions in the presence of 100 μg/mL (circles) and 300 μg/mL (squares) of 1.
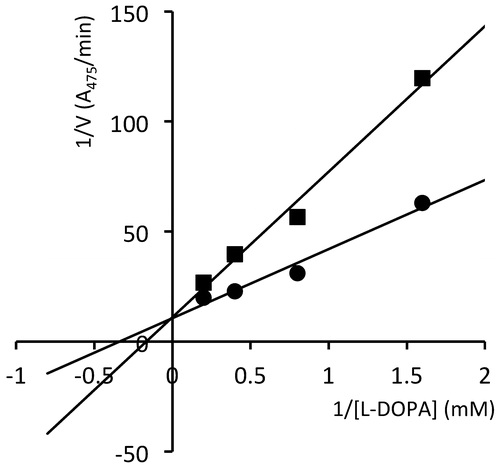
We also investigated the effects of these compounds on human recombinant tyrosinase. As shown in Figure , 1 and 2 clearly inhibited human tyrosinase at 500 μg/mL (Figure ).
Effects of 1 and 2 on melanogenesis in B16 melanoma cells
We examined the effects of treatments with 1 and 2 on the proliferation of B16 cells (Figure ). Since both compounds suppressed cell proliferation at concentrations higher than 30 μg/mL in preliminary experiment, we treated the cells with the compounds at 1, 3, and 15 μg/ml. Treatment with 1 did not affect the proliferation of cells at 15 μg/mL, whereas treatment with 2 caused a 72% decrease in cell numbers, indicating that 2 exhibited higher toxicity than 1.
Figure 6. Cytotoxicity of compounds 1 (A) and 2 (B) towards the B16 melanoma cells. The B16 cells were treated for 72 h with varying concentrations of 1 and 2 in the presence of α-MSH, and the number of viable cells was counted with a TC20 cell counter. Data are expressed as mean ± SD (n = 3). Different letters indicate statistical differences (p < 0.05, Tukey’s multiple comparison test). The photographs of harvested cells are shown as insets.
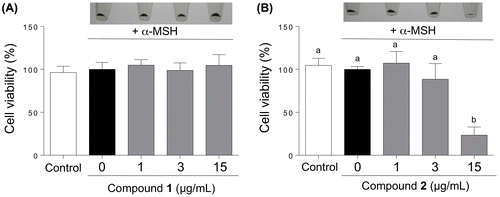
Then, we examined the inhibition of melanin accumulation in the cells by 1 and 2. The stimulation of cells by α-MSH induced an increased accumulation of melanin. Treatment with 1 at 15 μg/mL resulted in approximately 30% decrease in the melanin concentration (Figure (A)). Treatment with 2 at 15 μg/mL also resulted in a considerable decrease in melanin concentration (Figure (B)); however, the decrease was attributable to the decreased number of viable cells.
Figure 7. Effects of compounds 1 and 2 on the melanin content of B16 cells. The B16 cells were treated for 72 h with varying concentrations of 1 and 2 in the presence of α-MSH, and melanin contents were determined on the basis of the absorbance at 475 nm for the cell extracts. Data are expressed as mean ± SD (n = 3). Different letters indicate statistical differences (p < 0.05, Tukey’s multiple comparison test).
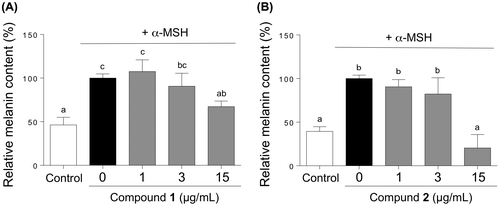
Further, we analyzed the effects of 1 on the expression of biosynthetic genes for melanin (TYR, TRP-1, and TRP-2) and the gene (MiTF) encoding the transcription factor that controls the expression of biosynthetic genes using quantitative reverse-transcriptase PCR (Figures (A)–(D)). The amount of TYR transcript decreased markedly after treatment with 1 at 15 μg/mL (Figure (A)), while the amounts of TRP-1, TRP-2, and MiTF transcripts were not affected (Figure (B)–(D)). Western blot analysis indicated that the decreased amounts of TYR transcript was reflected by a significantly decreased accumulation of TYR protein (Figure (E)). The treatment with 1 at 15 μg/mL did not significantly affect the amounts of TRP-1, TRP-2 and MiTF (Figure (E)).
Figure 8. Effects of treatment with 1 on the accumulation of transcripts of TYR (A), TRP-1 (B), TRP-2 (C), and MiTF (D), and on the accumulation of TYR, TRP1, TRP2, and MiTF proteins. The B16 cells were treated for 72 h with varying concentrations of 1 in the presence of α-MSH. The transcript levels were determined by quantitative RT-PCR with specific primer sets, and the proteins were detected by western blot analyses.
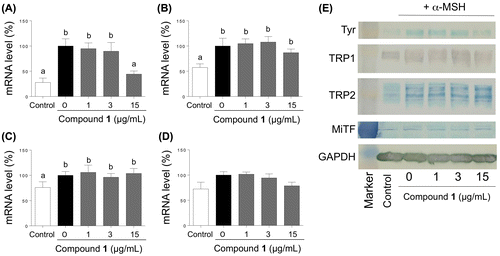
Discussion
We successfully identified the tyrosinase inhibitors, which had not been reported previously from mushroom extracts, proving that mushrooms are a promising target for searching novel bioactive compounds. The IC50 values of 1 and 2 for mushroom tyrosinase were 173 μg/mL (1 mM) and 263 μg/mL (1.4 mM), respectively. The values of hydroquinone and arbutin determined under the same conditions were 1970 μg/mL (17.8 mM) and 19,000 μg/mL (69.9 mM), respectively. Thus, 1 and 2 were considered more effective than these known tyrosinase inhibitors. The mode of inhibition of 1 was competitive inhibition, similar to that of arbutin [Citation23].
While analyzing the constituents of the N. lepideus fruiting body, various sesquiterpene hydrocarbons and alcohols were identified [Citation24,25]. In addition, the constituents of N. lepideus have been shown to exhibit multiple biological activities beneficial for human health. The water-soluble exopolysaccharides obtained from submerged culture of N. lepideus exhibited immunomodulatory activities [Citation26]. The extracts of N. lepideus fruiting body have showed antihyperlipidemic activities in hypercholesterolemic rats [Citation27] and strong antioxidant activity in inhibiting the oxidation of β-carotene and in scavenging 1,1-diphenyl-2-picrylhydrazyl radicals [Citation28]. Moreover, the extracts inhibited xanthine oxidase and tyrosinase activities. However, the specific compounds responsible for inhibition of these enzymes were not identified. Notably, 5-methoxyisobenzofuran-4,7(1H, 3H)-dione and 1,3-dihydroisobenzofuran-4,6-diol, together with multiple phenylpropanoids from the culture of N. lepideus were found to inhibit nitric oxide production in the lipopolysaccharide-induced macrophages [Citation29]. These compounds were similar to 1 and 2 because they possessed the same isobenzofuran skeleton. Compounds possessing the isobenzofuran skeleton are rarely found in nature, and the biosynthetic pathways for this class of compounds have not been investigated to date.
We observed that 1 and 2 at 15 μg/mL reduced the melanin content in the murine B16 cells that were stimulated by α-MSH, although 2 significantly inhibited cell proliferation at this concentration. The effective concentration was lower than the IC50 values for tyrosinase inhibition. This could be because of the effective incorporation of compounds into the cells. Alternatively, the long duration of treatment with compounds might have resulted in the inactivation of the enzyme. We also found that 1 negatively affected the transcript level of TYR and accumulation of TYR protein. Thus, the reduced melanin accumulation in murine B16 cells treated with 1 is likely attributable to the suppressed expression of TYR.
MITF plays the central role in the regulation of pigmentation proteins including TYR and TYRP1 and TYRP2. In our experiments, however, the changes in expression of MiTF and accumulation MITF protein were not obviously observed 72 h after α-MSH treatment. This could be attributable to the kinetics of expression of MiTF and/or turnover of MITF protein in the α-MSH treated cells. The treatment with 1 at 15 μg/mL suppressed of gene expression of TYR in spite of the unchanged expression levels of MiTF. In addition to transcription level of MiTF, multiple factors are involved in the regulation of TYR gene expression. For instance, the transactivation potential of MITF is affected by the phosphorylation. The Steel factor:c-Kit signaling pathway is linked to phosphoryalation of MITF at Ser73 and Ser409 through activation of MAP kinase and RSK-1, respectively [Citation30,31]. At the transcriptional level, besides MITF, other bHLH-LZ transcription factors, such as USF-1 and TFE3 can bind to the E-boxes in the TYR promoter and stimulate TYR expression [Citation32–34]. The TYR core-enhancer is another possible factor affecting the TYR expression level. The Sox10 transcription factor is able to specifically transactivate the TYR core-enhancer located in the 3.7 kb distal regulatory element [Citation35].
The secondary metabolites produced in liquid cultures of mushrooms are often different from the ones in the fruiting body. In N. lepideus, this was the case for 1 and 2 because we were unable to detect these compounds in the fruiting body. It is natural for the composition of secondary metabolites to be affected by differences in substrates. Thus, the fruiting body of N. lepideus might accumulate 1 and 2 depending on the substrate. In fact, the closely related compounds 5-methoxyisobenzofuran-4,7(1H,3H)-dione and 1,3-dihydroisobenzofuran-4,6-diol were isolated from the fruiting body of N. lepideus [Citation29]. Alternatively, 1 and 2 might be the compounds that are produced and function only in mycelia.
Only a limited number of tyrosinase inhibitors have been purified from fungi and chemically identified. These compounds include azelaic acid (1,7-heptanedicarboxylic acid) from Pityrosporum ovale [Citation36], kojic acid from Aspergillus and Penicillium [Citation37], and agaritine (β-N-(γ-L-glutamyl)-4-hydroxymethylphenylhydrazine) from Agaricus bisporus [Citation38]. However, the inhibitory activity on tyrosinase has been detected in the extracts of many mushrooms species. Extracts from fruiting bodies of 13 species, and from mycelia of 17 species and culture filtrates of 17 species were listed as the potential sources for tyrosinase inhibitors in a Japanese patent [Citation39] although the active principles of these extracts have not been identified. Indeed, we have also detected tyrosinase inhibitory activity in the extracts from multiple edible mushrooms. The analysis of edible mushrooms could be a fruitful approach in discovering novel tyrosinase inhibitors.
Author contribution
Atsushi Ishihara designed experiments. Yuri Ide and Tomohiro Bito performed the experiments, while Tomohiro Bito, Naoki Ube, Kotomi Ueno discussed on the experimental details. Naoki Endo, Kozue Sotome, Nitaro Maekawa, Akira Nakagiri prepared experimental materials including mushrooms. Tomohiro Bito, Kotomi Ueno and Atsushi Ishihara analyzed data, and edited the manuscript.
Supplemental data
Supplemental data for this article can be accessed https://doi.org/10.1080/09168451.2017.1415125.
Disclosure statement
No potential conflict of interest was reported by the authors.
Supplementary_materials.pdf
Download PDF (90.6 KB)Acknowledgements
We thank Mr Akito Koyama, Mr Toshikazu Imoto, Ms Sayo Imoto, and Ms Michiyo Nabe for kindly providing fruiting bodies of N. lepideus.
References
- Sanchez-Ferrer A, Rodriguez-Lopez JN, Garcia-Ganovas F, et al. Tyrosinase: a comprehensive review of its mechanism. Biochim Biophys Acta. 1995;1247:1–11.
- Yokoyama K, Yasumoto K, Suzuki H, et al. Cloning of the human DOPA chrome tautomerase/tyrosinase-related protein 2 gene and identification of two regulatory regions required for its pigment cell-specific expression. J Biol Chem. 1994;269:27080–27087.
- Kobayashi T, Urabe K, Winder A, et al. Tyrosinase related protein 1 (TRP-1) functions as a DHICA oxidase in melanin biosynthesis. EMBO J. 1994;13:5818–5825.
- Tachibana M. MITF: a stream flowing for pigment cells. Pigment Cell Res. 2000;13:230–240.10.1034/j.1600-0749.2000.130404.x
- Slominski A, Tobin DJ, Shibahara S, et al. Melanin pigmentation in mammalian skin and its hormonal regulation. Physiol Rev. 2004;84:1155–1228.10.1152/physrev.00044.2003
- Hodgkinson CA, Moore KJ, Nakayama A, et al. Mutations at the mouse microphthalmia locus are associated with defects in a gene encoding a novel basic-helix-loop-helix-zipper protein. Cell. 1993;74:395–404.10.1016/0092-8674(93)90429-T
- Steingrímsson E, Moore KJ, Lamoreux ML, et al. Molecular basis of mouse microphthalmia (mi) mutations helps explain their developmental and phenotypic consequences. Nat Genet. 1994;8:256–263.10.1038/ng1194-256
- Arandt KA, Fitzpatrick TB. Topical use of hydroquinine as a depigmenting agent. J Am Med Assoc. 1965;194:117–119.
- Fitzpatrick TB, Arandt KA, El-Mofty AMMA. Hydroquinone and psoralens in the therapy of hypermelanosis and vitiligo. Arch Dermatol. 1966;93:589–600.10.1001/archderm.1966.01600230093025
- Maeda K, Fukuda M. In vitro effectiveness of several whitening cosmetic components in human melanocyte. J Soc Cosmet Chem. 1991;42:361–368.
- Kligman AM, Willis I. A new formula for depigmenting human skin. Arch Dermatol. 1975;111:40–48.10.1001/archderm.1975.01630130042004
- Matsuki M, Watanabe T, Ogasawara A, et al. Inhibitory mechanism of melanin synthesis by glutathione. J Pharm Soc Jpn (Yakugaku Zasshi). 2008;128:1203–1207.10.1248/yakushi.128.1203
- Aoyama Y, Ito A, Suzuki K, et al. The first epidemiological report of rhododenol-induced leukoderma in Japan based on a nationwide survey. Jpn J Dermatol. 2014;124:2095–2109.
- Suzuki K, Aoyama Y, Ito A, et al. The second epidemiology report of rhododenol-induced leukoderma in Japan based on a nationwide survey. Jpn J Dermatol. 2014;124:3125–3142.
- Sasaki M, Kondo M, Sato K, et al. Rhododendrol, a depigmentation-inducing phenolic compound, exerts melanocyte cytotoxicity via a tyrosinase-dependent mechanism. Pigm Cell Melanom Res. 2014;27:754–763.10.1111/pcmr.2014.27.issue-5
- Nishino S, Parada RY, Ichiyanagi T, et al. 1-Phenyl-3-pentanone, a volatile compound from the edible mushroom Mycoleptodonoides aitchisonii active against some phytopathogenic fungi. J Phytopathol. 2013;161:515–521.10.1111/jph.2013.161.issue-7pt8
- Oka K, Ishihara A, Sakaguchi N, et al. Antifungal activity of volatile compounds produced by an edible mushroom Hypsizygus marmoreus against phytopathogenic fungi. J Phytopathol. 2015;163:987–996.10.1111/jph.2015.163.issue-11-12
- Ogiyama K. Cultivation of brown rot fungus, Matsuozi (Lentinus lepideus Fr.) in Sugi (Cryptomeria japonica D. Don) wood medium. Bull Yamagata Univ Agr Sci. 1986;10:19–31.
- Shimizu K, Kondo R, Sakai K, et al. The inhibitory components from Artocarpus incisus on melanin biosynthesis. Planta Med. 1998;64:408–412.10.1055/s-2006-957470
- Arung ET, Shimizu K, Kondo R. Inhibitory effect of artocarpanone from Artocarpus heterophyllus on melanin biosynthesis. Biol Pharm Bull. 2006;29:1966–1969.10.1248/bpb.29.1966
- Sugai T. Clinical effects of arbutin in patients with chloasma. Skin Res. 1992;34:522–539 (in Japanease).
- Draelos ZD. Skin lightening preparations and the hydroquinone controversy. Dermatol Ther. 2007;20:308–313.10.1111/dth.2007.20.issue-5
- Maeda K, Naganuma M. Depigmenting action of and its new aspects in cosmetic science. J Jpn Cosmet Sci Soc. 1996;20:12–16.
- Hanssen HP. Sesquiterpene hydrocarbons from Lentinus lepideus. Phytochemistry. 1982;21:1159–1160.10.1016/S0031-9422(00)82440-1
- Hanssen HP. Sesquiterpene alcohols from Lentinus lepideus. Phytochemistry. 1985;24:1293–1294.10.1016/S0031-9422(00)81118-8
- Jung YS, Yang BK, Jeong YT, et al. Immunomodulating activities of water-soluble exopolysaccharides obtained from submerged culture of Lentinus lepideus. J Microbiol Biotechnol. 2008;18:1431–1438.
- Yoon KN, Lee JS, Kim HY, et al. Appraisal of antihyperlipidemic activities of Lentinus lepideus in hypercholesterolemic rats. Mycobiology. 2011;39:283–289.10.5941/MYCO.2011.39.4.283
- Yoon KN, Alam N, Lee KR, et al. Antioxidant and antityrosinase activities of various extracts from the fruiting bodies of Lentinus lepidus. Molecules. 2011;16:2334–2347.10.3390/molecules16032334
- Li Y, Bao L, Song B, et al. A new benzoquinone and a new benzofuran from the edible mushroom Neolentinus lepideus and their inhibitory activity in NO production inhibition assay. Food Chem. 2013;141:1614–1618.10.1016/j.foodchem.2013.04.133
- Price ER, Ding H-F, Badalian T, et al. Lineage-specific signaling in melanocytes. C-kit stimulation recruits p300/CBP to microphthalmia. J Biol Chem. 1998;273:17983–17986.10.1074/jbc.273.29.17983
- Wu M, Hemesath TJ, Takemoto CM, et al. c-Kit triggers dual phosphorylations, which couple activation and degradation of the essential melanocyte factor Mi. Genes Dev. 2000;14:301–312.
- Corre S, Galibert M. Upstream stimulating factors: highly versatile stress-responsive transcription factors. Pigment Cell Res. 2005;18:337–348.10.1111/pcr.2005.18.issue-5
- Corre S, Primot A, Sviderskaya E, et al. UV-induced expression of key component of the tanning process, the POMC and MC1R genes, is dependent on the p-38-activated upstream stimulating factor-1 (USF-1). J Biol Chem. 2004;279:51226–51233.10.1074/jbc.M409768200
- Verastegui C, Bertolotto C, Bille K, et al. TFE3, a transcription factor homologous to microphthalmia, is a potential transcriptional activator of tyrosinase and TyrpI genes. Mol Endocrinol. 2000;14:449–456.10.1210/mend.14.3.0428
- Murisier F, Guichard S, Beermann F. The tyrosinase enhancer is activated by Sox10 and MITF in mouse melanocytes. Pigm Cell Res. 2007;20:173–184.10.1111/pcr.2007.20.issue-3
- Schallreuter KU, Wood JW. A possible mechanism of action for azelaic acid in the human epidermis. Arch Dermatol Res. 1990;282:168–171.10.1007/BF00372617
- Parrish FW, Wiley BJ, Simmons EG, et al. Production of aflatoxins and kojic acid by species of Aspergillus and Penicillium. App Microbiol. 1966;14:139.
- Espín JC, Jolivet S, Wichers HJ. Inhibition of mushroom polyphenol oxidase by agaritine. J Agric Food Chem. 1998;46:2976–2980.10.1021/jf9802732
- Morimura K, Michihata T, Kokame S, et al. Mushroom extracts. Japan Patent JP5154596; 2012.