Abstract
Quercetin (QT) is a plant polyphenol with various pharmacological properties. However, the low water solubility limits its therapeutic efficacy. In the present study, QT-loaded sodium taurocholate-Pluronic P123 (QT-loaded ST/P123) mixed micelles were developed and characterized, and the effect of the formulation on improving the water solubility of QT was investigated. QT-loaded ST/P123 mixed micelles were prepared by thin film hydration-direct dissolution and optimized by uniform design. The optimal formulation possessed high drug loading (12.6%) and entrapment efficiency (95.9%) in small (16.20 nm) spherically-shaped micelles. A low critical micelle concentration indicated that the micelles were stable, and they showed a sustained release pattern, as determined in vitro in simulated gastric fluid and intestinal fluid. Pharmacokinetic evaluation showed the Cmax and AUC0–24 were 1.8-fold and 1.6-fold higher than the QT suspension. The present results indicate that QT-loaded ST/P123 micelles are potential candidates to improve the solubility and oral bioavailability of QT.
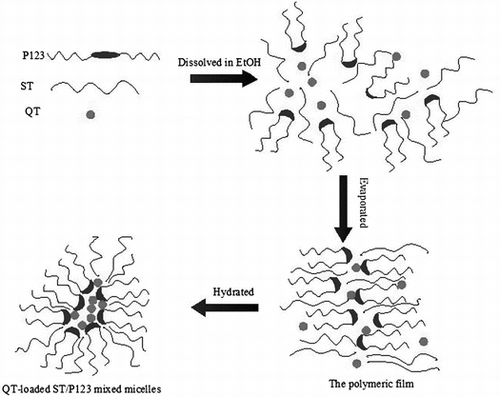
Schematic illustration of QT-loaded mixed micelles. ST, P123 and QT were dissolved in EtOH, then evaporated to obtain thin film, followed by hydration to form a uniform system.
Oral administration remains the preferred drug delivery route because it is convenient and improves patient compliance. In the gastrointestinal (GI) tract, however, gastric acid, bile, and various digestive enzymes cause drug degradation, leading to decreased absorption efficacy. Therefore, new strategies are needed to facilitate drug transport from the GI tract to the blood circulation and improve oral bioavailability [Citation1–3].
Quercetin (QT, 3,3′,4′,5,7-pentahydroxy flavone) is a potent polyphenolic flavonoid antioxidant with various pharmacological properties, including anti-inflammatory [Citation4,5], antioxidative [Citation6,7], antiviral [Citation8,9], and anticancer effects [Citation10]. However, the clinical use of QT is limited by its poor water-solubility, GI instability, minimal oral bioavailability (<17% in rats and <2% in humans) [Citation11], and extensive first pass metabolism before reaching the systemic circulation [Citation12]. To overcome these shortcomings, recent studies have relied on nanotechnology [Citation13] to develop various QT-loaded nanocarriers such as nanoparticles [Citation14–18], polymeric micelles [Citation19–21], conjugates [Citation22], inclusion complexes [Citation23], and nanosuspensions [Citation24]. Mixed micelles with nanosized distribution have several favorable properties, such as increased micelle stability, solubility, and bioactivity. A simple preparation process and low toxicity in the absence of organic solvents make mixed micelles potential candidates for QT delivery, especially for oral administration [Citation25,26]. For instance, lecithin-based mixed polymeric micelles improved the solubility of QT loaded in nanosystems to 5.81 mg/mL compared with 0.17–7.7 μg/mL for free QT in water [Citation12].
Pluronic P123 (P123), composed of PEO20-PPO68-PEO20, is an amphiphilic synthetic polymer containing hydrophilic poly(ethylene oxide) (PEO) block as an outer shell and hydrophobic poly(propylene oxide) (PPO) block as an inner core [Citation27,28]. The PPO core can serve as a “pool” for the encapsulation of hydrophobic drugs in the lipophilic microenvironment, whereas the hydrophilic PEO corona maintains the dispersion stability of micelles [Citation29]. Bile salt is considered as an important biosurfactant with strong solubilization ability and good biocompatibility [Citation30], and mixed micelles containing bile salt increase the solubility and improve the oral bioavailability of hydrophobic drugs [Citation31–33].
In the present work, QT loaded sodium taurocholate/Pluronic P123 (QT-loaded ST/P123) mixed micelles were initially prepared to improve the solubility of QT in water, increase oral absorption, and protect the drug during passage through the GI tract. The mixed micelles were prepared by the thin-film hydration-direct dissolution method and the preparation was optimized by uniform design. The physicochemical properties were characterized and the drug release pattern was evaluated in simulated gastric fluid and intestinal fluid. The pharmacokinetics of QT-loaded ST/P123 micelles were investigated in rats.
Materials and methods
Materials and animals
QT, Pluronic P123 (P123), and sodium taurocholate (ST) were purchased from Sigma-Aldrich (USA). Tween 80 was provided by Tianjin Kermel Chemical Ltd (China). All other chemicals used were of analytical grade.
Male Wistar rats (weight: 200 ± 20 g) were supplied by the Laboratory Animal Center of Shandong University (China). The rats were kept in cages for 2 weeks before experiments and fed with food and water ad libitum. Before the experiments, the rats were fasted for 12 h with free access to water. The procedures complied with the requirements of the Institute’s Animal Care and Use Committee of Shandong University.
Preparation of QT-loaded ST/ P123 mixed micelles
QT-loaded ST/P123 mixed micelles were prepared by the thin-film hydration-direct dissolution method [Citation34,35]. Briefly, QT, ST, and P123 (the total amount of ST and P123 was 200 mg) were dissolved in ethanol and the solvent was removed under reduced pressure to acquire a thin polymeric film in a 50-ml round-bottom flask. The thin film was dried under vacuum overnight, and the resulting dry polymeric film was hydrated with 4 mL of deionized water, and then vortexed for 5 min. Finally, the mixture was centrifuged at 12,000 rpm for 5 min to remove unloaded drugs, resulting in a clear supernatant as the micelle solution.
Optimization of the QT-loaded ST/P123 mixed micelle formulation
The uniform design method was used to determine the optimal formulation of QT-loaded ST/P123 micelles. The complete design consisted of 9 (32) experimental points. Before using the design, a great number of single-factor experiments were preliminarily conducted using drug loading coefficient (DL) and encapsulation efficiency (EE) as evaluation indexes.
Characterization of QT-loaded ST/P123 mixed micelles
Particle size distribution and zeta potential
The size distribution and zeta potential of QT-loaded ST/P123 micelles were measured using a dynamic light scattering instrument (DLS, DELSATM NANO particle size, and zeta potential analyzer, Beckman Coulter Inc., USA) at 25 °C. Measurements were processed in triplicate for each sample.
Surface morphology
The surface morphology of micelles was observed by transmission electron microscopy (TEM, JEM-100SX, JEOL, Japan) after negative staining with a phosphotungstic acid solution (1.5%, w/v).
The thermal characteristics of the QT, blank micelles, physical mixture, and QT-loaded ST/P123 mixed micelles were determined using a TGA/DSC1 instrument (Mettler Toledo, Switzerland) under a 10 mL/min stream of nitrogen purge. All samples were heated from 30 °C to 300 °C at the rate of 10 °C/min in an open aluminum pan, and TA4 Analysis software was used for analysis.
Differential scanning calorimetry (DSC)
Thermograms of QT, blank micelles, a physical mixture of QT and blank micelles, and QT-loaded micelles were recorded on a differential scanning calorimeter (Mettler Toledo). Weighed samples of 10 mg were placed in aluminum pans and heated at a rate of 10 °C from 25 °C to 400 °C in a nitrogen atmosphere. Aluminum oxide was used as the internal standard.
Drug loading and entrapment efficiency
To determine the DL content and EE of micelles, QT-loaded ST/P123 mixed micelles were dissolved in methanol to break up the nanostructures. The concentration of QT was then detected by an ultraviolet-visible spectrophotometer (UV-2102, Shanghai Instrument Ltd., China) at 376 nm. The DL and EE were calculated using the following equations:[Citation36]:
Critical micelle concentration determination
Critical micelle concentration (CMC) of the mixed micelles was determined by fluorescence spectroscopy using pyrene as the fluorescence probe [Citation37]. When the concentration of polymers increased up to the CMC in an aqueous environment, lipophilic pyrene tended to accumulate in the inside core of micelles, resulting in an alteration in the intensity ratio of I374/I384. The fluorescence spectrum was recorded on a fluorescence spectrophotometer at room temperature. In brief, pyrene was dissolved in acetone, before being diluted to a final concentration of 2.0 × 10−6 M. A total of 100 μL of pyrene solution was extracted into a colorimetric tube and the solvent was evaporated under nitrogen flow. After that, a series of polymer solutions with different concentrations (ranging from 1 × 10−3 M to 3 M) was added into the colorimetric tubes and vortexed for 5 min. After storage in the dark for 24 h, the fluorescence spectra of samples were recorded at 350–500 nm. The excitation wavelength was set at 334 nm, and the excitation and emission slit widths were set at 5 and 2.5 nm, respectively.
In vitro release studies
Dialysis was used to investigate the in vitro release pattern of QT from QT- loaded ST/P123 mixed micelles using a dialysis bag (MWCO 3500 Da, Solarbio, China). QT-loaded ST/P123 mixed micelles (200 μL) were introduced into the dialysis membrane bag, and the end-sealed dialysis bag was then immersed in 50 mL release medium at 37 ± 0.5 °C and shaken at a speed of 100 rpm. After 2 h of incubation in simulated gastric fluid (SGF, pH = 1.2), the bag was transferred into simulated intestinal fluid (SIF, pH = 6.8) for the next 46 h. At predetermined time intervals, 2 mL of the release media was withdrawn and replaced with an equal volume of the fresh release medium. The concentration of QT was determined by the UV method with 375 nm as the determination wavelength, and the cumulative release of QT was calculated according to the formula described below. The release pattern of QT from QT propylene glycol solution was assessed under the same conditions as the control group. All samples were performed in triplicate.
where Er is DTX accumulative release, %; Ve stands for displacement volume of release medium, 2 mL; V0 represents the volume of release medium, 80 mL; Ci is the release solution concentration at the displaced time, mg/mL; mdrug stands for QT amount of QT-loaded mixed micelles (mg); and n is the number of times of release medium displacement.
Pharmacokinetic studies
Wistar rats were randomly divided into two groups, and intragastrically administered QT-loaded ST/P123 mixed micelles and QT suspension with the same concentration. QT suspension was prepared by dispersing 40 mg QT in 10 mL of 0.4% carboxymethylcellulose sodium solution. The dose of QT in all samples was maintained at 80 mg/kg. At each time point (0.25, 0.5, 1, 2, 4, 6, 8, 10, 12, and 24 h), 300 μL of blood was collected from the sinus jugularis of rats and placed into heparinized tubes. Samples were then centrifuged immediately (4000 rpm for 10 min) and the separated plasma was stored at –20 °C until analysis. Rat plasma (200 μL) was added to acetonitrile-acetic acid (400 μL, 9:1, v/v), then vortexed for 90 s, hydrolyzed in a 50 °C water bath for 15 min, and then centrifuged (10,000 rpm for 10 min). The supernatant (20 μL) was analyzed by HPLC. The pharmacokinetic parameters were calculated using DAS (version 2.0).
HPLC analysis of QT
The samples were analyzed using an HPLC system (1200 series; Agilent Technologies, USA) and an HPLC column (C18, 4.6 × 250 mm, 5 μm). The mobile phase was a mixture of acetonitrile-water at a ratio of 33: 67 (v:v) containing 0.75% acetic acid. The flow rate was 1 mL/min, and the detection wavelength was 376 nm. Aliquots of 20 μL of each sample were injected into the column, and all operations were performed at ambient temperature.
Statistical analysis
Data expressed as the mean ± standard deviation (SD) are representative of at least three different experiments. Statistical data were analyzed by the Student’s t-test at the level of p < 0.05.
Results
Optimization of QT-loaded P123 /ST mixed micelles
Single-factor experiment
Single-factor experiments were performed to obtain optimal formulations. The EE and DL were selected as indexes to optimize the formulation of QT-loaded P123/ST mixed micelles. The results of preliminary experiments showed that carriers ratio (P123:ST), hydration temperature, and the added amount of QT affected the DL and EE. Therefore, single-factor experiments were performed to determine the appropriate ranges of these three factors. Table shows the EE and DL values according to P123:ST ratio, hydration temperature, and QT amount. The highest DL and EE values were obtained at a P123:ST ratio of 1:1, a temperature of 40 °C, and with 30 mg of added QT.
Table 1. Single-factor experiments with investigated factors and observed results at different levels.
Optimization of QT-loaded P123/ST micelles based on uniform design
The independent variables affecting the DL and EE in the uniform design are shown in Table . The multiple regression method was used to fit the data to regression equations. The quadratic polynomial equation generated by the design was as follows:
Table 2. Results of uniform design experiments.
where S stands for , X1 represents the ratio of P123 to ST, and X2 represents the amount of added QT.
From the formula, all the dependent variables can be described by the quadratic polynomial with a high correlation coefficient (r > 0.95), and response surface plots (Figure ) were generated according to the regression equations. These results indicated that the added amount of QT played a crucial role in DL and EE. Increasing the QT in formulations increased the amount of QT likely to be encapsulated in micelles, resulting in increased DL and EE. However, the EE decreased when the QT amount exceeded the entrapment capability of the micelles. Considering all the factors, the optimal formulation was set as follows: P123:ST ratio of 120:80 mg, QT of 30 mg, hydration temperature of 40 °C, and hydration volume of 4 mL.
Characterization of mixed micelles
TEM is a practical technique to visualize the morphology of micelles. As shown in Figure (A), the mixed micelles generated homogeneous nanometric spheres with an obvious core-shell structure. In addition, the micelles showed good formability and uniform dispersion without aggregation. The outer shell of the micelles was formed by the ST and PEO in P123, and the inner core consisted of hydrophobic PPO and QT.
Figure 2. Characterization of mixed micelles. Transmission electron microscope images of QT-loaded mixed micelles (A); size distribution of micelles without QT (B) and QT-loaded mixed micelles (C); the zeta potential distributions of unloaded mixed micelles (D) and QT-loaded mixed micelles (E); external photos of QT-loaded mixed micelles in water (F-i) and QT in water at the same concentration (F-ii).
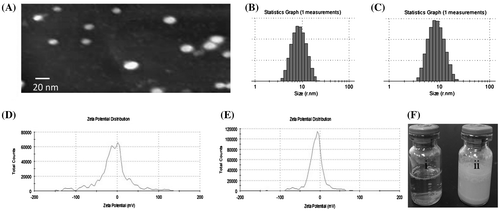
The mean size of the QT-loaded mixed micelles was 16.20 ± 2.12 nm, with a polydispersity index (PDI) of 0.123 ± 0.071, and that of the micelles without QT was 17.67 ± 1.97 nm (PDI: 0.213 ± 0.054) (Figure (B) and (C)). Analysis of the zeta potential of blank ST/P123 and QT-loaded ST/P123 micelles showed that the surface was negatively charged, with zeta-potential values of −8.65 ± 0.972 mV and −10.0 ± 1.192 mV, respectively, indicating the good stability of micelles (Figure (D) and (E)). The solubility of QT in the optical QT-loaded micelle solution determined using the UV method was 7.08 mg/mL, which was higher than that of free QT in water (0.17–7.7 μg/mL). With respect to the surface morphology, QT-loaded mixed micelles aqueous solution was clear and transparent, while the QT dispersing in water at equal mass appeared turbid, non-transparent and insoluble state (Figure (F)).
Figure shows the thermograms of QT, blank micelles, the physical mixture of QT and blank micelles, and QT-loaded micelles. QT (a) exhibited a sharp endothermic peak at 315 °C, which could be attributed to its crystalline nature, as reported previously [Citation38]. The blank micelles (c) showed a broad endothermic peak at 90 °C, whereas in the physical mixture with QT (b), a smaller peak at 320 °C was observed for QT, indicating the presence of remaining crystalline drugs. The QT-loaded micelles showed no endothermic peak around the melting point of crystal QT (d), suggesting that the QT in micelles was in a noncrystalline state.
CMC determination
When the concentration of polymers increases above the CMC, P123, and ST can self-assemble into aggregates in an aqueous environment. Therefore, the CMC value is an important parameter and micelles with a low CMC show high stability and anti-dilution properties [Citation39]. As a hydrophobic molecule, pyrene was used as a probe because it preferentially enters the hydrophobic microenvironment of aggregates, and its solubility significantly increases when the concentration of polymers increases above the CMC. The CMC values of ST/P123 mixed aggregates were obtained by plotting the I374:I384 ratio of the emission spectrum profile against the concentration of aggregates, as shown in Figure . The inflection point of the ST/P123 mixed micelles was 3.25 × 10−3 g/L.
Drug release profile in simulated GI tract
The in vitro profile of the release of QT from ST/P123 micelles was determined in a simulated GI tract including SGF (pH 1.2) and SIF (pH 6.8). As shown in Figure , 29.20% of the QT was released from QT-loaded ST/P123 micelles in SGF within 2 h and then 78.56% in SIF within 24 h. However, 37.96% of the QT was released from propylene glycol in SGF within 2 h and then 99.64% in SIF within 11 h. Therefore, the QT-loaded mixed micelles showed a controlled and sustained release pattern.
In vivo pharmacokinetics
A part of QT was metabolized into glycuronate or sulfated compounds after entering blood through the oral absorption. The hydrolysis process could revert these glycuronate or sulfated compounds back to the QT form, and then all the QT would be analyzed with the method of HPLC [Citation40]. The total concentration of QT that was absorbed into blood from the QT-loaded ST/P123 mixed micelles and QT suspension during a 24 h period was determined. Pharmacokinetic analysis showed that the total QT plasma concentration was significantly higher for the QT-loaded ST/P123 mixed micelles than for the QT suspension (p < 0.05), as determined by plasma concentration-time curves (Figure ). In accordance with the result of in vitro drug release, QT was detectable in plasma within 24 h, indicating that QT was released from QT-loaded ST/P123 mixed micelles in a sustained manner over a long period of time. The pharmacokinetic parameters are listed in Table . For QT-loaded ST/P123 micelles, the peak concentration (Cmax) of the drug in mixed micelles (5.13 mg/L) was 1.8-fold higher than that of the QT suspension (2.82 mg/L). The area under the concentration-time curve (AUC0–24), a key drug therapeutic index, was 80.42 mg/L × h for QT-loaded ST/P123 mixed micelles, which was 1.6-fold higher than that of the QT suspension at 50.38 mg/L × h (Table ).
Figure 6. The plasma concentration-time curves of QT suspension and QT-loaded mixed micelles. Values are presented as the mean ± SD (n = 5).*p < 0.05 vs. QT solution.
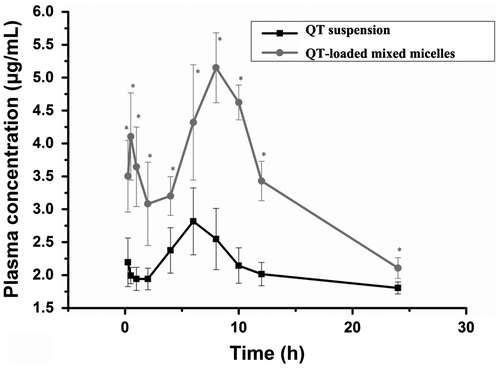
Table 3. Pharmacokinetic parameters after oral administration (values are presented as the mean ± SD, n = 5).
Discussion
Drug delivery systems based on polymeric micelles have gained attention because of their potential to increase drug absorption by protecting drugs from the GI tract environment, providing controlled drug release at target sites, increasing the time of residence of a drug in the gut, and improving the bioavailability and delivery of poorly water-soluble drugs [Citation41]. Amphiphilic polymers that assemble into nanocarriers with a hydrophobic core and a hydrophilic shell have been developed to improve the delivery of poorly soluble drugs [Citation42,43]. The encapsulation of hydrophobic drugs in the core protects them from degradation, increasing bioavailability, and the small size of micelles increases circulation time and favors biodistribution [Citation44,45]. Here, QT-loaded ST/P123 mixed micelles were developed, characterized, and optimized, and shown to improve the solubility of QT, increase oral absorption, and protect the drug during passage through the GI tract.
For oral administration, the particle size and surface properties of nanocarriers could affect the absorption rate in the GI tract [Citation19]. QT-loaded mixed micelles showed a slight decrease in the average size comparing with the micelles without QT, which could be attributed to the formation of a compact hydrophobic core due to the addition of QT and increased hydrophobic interaction between drugs and carriers. A small size prolongs the retention time of micelles in intestinal villi and increases the internalization ability via the intestinal epithelium [Citation46,47]. The nanoparticles with sizes of 10–100 nm can avoid being filtered out by the kidneys or absorbed and metabolized by the liver [Citation48]. Therefore, QT-loaded mixed micelles with a particle size of 16.2 nm can avoid rapid metabolism and elimination, ensure stability and long circulation time, and improve targeting abilities by increasing permeability and retention.
The zeta potential has an important effect on the storage stability of colloid dispersion systems, and particle aggregation is likely to occur if the zeta potential of particles is too low to provide sufficient electric repulsion or steric barriers [Citation49]. The zeta potential of QT-loaded ST/P123 micelles was slightly higher than that of blank micelles. This change in zeta potential could be attributed to the dissociation of acidic phenolic OH groups on the molecule of QT [Citation50]. Chitkara et al. prepared QT-loaded poly(lactic-co-glycolic acid) nanoparticles by the emulsion diffusion evaporation method and obtained a zeta potential of −6.06 mV [Citation11]. Xu et al. used a self-assembly method to prepare QT-loaded MPEG-PCL nanomicelles. The zeta potential of the nanomicelles was only −1.56 mV [Citation51]. The higher the zeta potential value, the higher the electrostatic repulsion, which results in a greater stability of particles. Compared with the above results, the QT-loaded ST/P123 micelles with a zeta potential of −10.0 mV could have better stability.
In the present study, ST and P123 mixed micelles were used to increase the water solubility of QT. The capacity of the polymer micelles to increase the aqueous solubility of hydrophobic molecules is related to their hydrophobic core, which provides a suitable microenvironment for a hydrophobic solute [Citation52]. The effects of mixed polymeric micelles prepared using P123 on improving the solubility of QT were reported previously [Citation53]. The presence of ST in micelle preparations increased the solubilities of poorly soluble drugs, which was consistent with previous studies [Citation48].
The physicochemical status of QT in the present formulation was assessed by DSC. The results of DSC demonstrated that the phase state of QT in mixed micelles had changed and the drug was highly dispersed in the micelles system. The change in the structure of QT in mixed micelles suggested that QT in the micelles was in a noncrystalline state, such as an amorphous or disorder crystalline phase of molecular dispersion [Citation28], which was favorable to improve the solubility of poorly water-soluble drugs.
The delivery of drugs by the oral route requires resistance against degradation in the acidic environment of the GI tract and in the presence of digestive enzymes and bile salts. CMC is the threshold at which polymer molecules start to assemble into polymeric micelles, and a low CMC value denotes a greater stability [Citation54]. In the present study, low CMC values indicated that the mixed micelles composed of ST/P123 maintained their integrity even upon extreme dilution in the GI tract.
QT-loaded mixed micelles showed a controlled and sustained release pattern. The slower release of QT from QT-loaded ST/P123 micelles compared with that of QT propylene glycol in SGF/SIF could be attributed to the encapsulation of QT in the core of micelles. With the uptake of water, micelles are likely to swell, leading to drug diffusion and the erosion of polymers [Citation55]. However, the diffusion of water into micelles could be delayed because of the hydrophobic core structure, which contributed to their sustained release. This property contributed to preventing rapid leakage and precipitation within the GI lumen during drug delivery via oral administration.
The in vivo pharmacokinetic study was performed to investigate the oral bioavailability of QT. As shown in Figure , the total QT plasma concentration of the QT-loaded ST/P123 mixed micelles was significantly higher than that of the QT suspension, which could have a positive effect on the therapeutic index. The Cmax of the free QT in mixed micelles was higher than that of the QT suspension, indicating that a greater amount of QT was absorbed in the GI tract from QT-loaded micelles. The results might be attributed to the in vivo behaviors difference between QT suspension and QT-loaded mixed micelles. For QT suspension, it was difficult for hydrophobic QT to be absorbed by mucus, leading to the reduced accumulation in the blood circulation and a low Cmax. However, for QT-loaded mixed micelles, the micelles increased the drug solubility and stability in the GI tract and the small particle size enabled the whole micelles to penetrate across the pre-epithelial medium mucus and then be uptaken into enterocyte [Citation54]. In addition, free QT could enter the enterocyte with the help of various surfactants after the diffusion of the micelles, and many drugs were absorbed into the enterocyte by passive diffusion. Meanwhile, the component P123 in mixed micelles could inhibit P-gp transporter in the enterocyte to prevent the efflux of QT [Citation56,57]. Therefore, the ST/P123 mixed micelles dramatically increased the total concentration of QT in plasma and significantly improved the oral bioavailability of QT.
In this study, QT-loaded mixed micelles composed of Pluronic P123 and ST copolymers for oral administration were prepared and the formulation was optimized by uniform design. The prepared QT-loaded micelles showed high stability and a controlled and sustained release pattern. The results indicate that QT-loaded ST/P123 mixed micelles could be a potential nanoformulation to enhance the solubility and oral bioavailability of QT.
Author contributions
Conceived and designed the experiments: Guangxi Zhai, Zhen Lu, Cuiping Bu. Performed the experiments: Hui Zhang, Mengrui Liu, Meiqi Lu. Contributed reagents/materials/ analysis tools: Zhen Lu, Cuiping Bu, Weicheng Hu. Wrote the paper: Guangxi Zhai, Zhen Lu, Cuiping Bu, Weicheng Hu. All the authors reviewed the results and approved the final version of the manuscript.
Disclosure statement
No potential conflict of interest was reported by the authors.
Funding
This work was supported by the National Natural Science Foundation of China [grant number 31300463]; the Natural Science Foundation of Jiangsu Province [grant number BK20130414]; Qing Lan Project of Jiangsu Province; and the Science Research Program of Taian [grant number 201440774-30B].
Acknowledgments
The authors gratefully acknowledge the help of Xingzhen Zhang, who provided technical assistance with HPLC.
References
- Duan Y, Zhang B, Chu L, et al. Evaluation in vitro and in vivo of curcumin-loaded mPEG-PLA/TPGS mixed micelles for oral administration. Colloids Surf B. 2016;141:345–354.10.1016/j.colsurfb.2016.01.017
- Lee DR, Ho MJ, Jung HJ, et al. Enhanced dissolution and oral absorption of tacrolimus by supersaturable self-emulsifying drug delivery system. Int J Nanomed. 2016;11:1109–1117.
- Khani S, Keyhanfar F, Amani A. Design and evaluation of oral nanoemulsion drug delivery system of mebudipine. Drug Delivery. 2016;23(6):2035–2043.10.3109/10717544.2015.1088597
- Chanput W, Krueyos N, Ritthiruangdej P. Anti-oxidative assays as markers for anti-inflammatory activity of flavonoids. Int Immunopharmacol. 2016;40:170–175.10.1016/j.intimp.2016.08.038
- Caddeo C, Díez-Sales O, Pons R, et al. Topical anti-inflammatory potential of quercetin in lipid-based nanosystems: in vivo and in vitro evaluation. Pharm Res. 2014;31(4):959–968.10.1007/s11095-013-1215-0
- Bagad M, Khan ZA. Poly (n-butylcyanoacrylate) nanoparticles for oral delivery of quercetin: preparation, characterization, and pharmacokinetics and biodistribution studies in Wistar rats. Int J Nanomed. 2015;10:3921–3935.
- Gomes IB, Porto ML, Santos MC, et al. Renoprotective, anti-oxidative and anti-apoptotic effects of oral low-dose quercetin in the C57BL/6J model of diabetic nephropathy. Lipids Health Dis. 2014;13(1):184.10.1186/1476-511X-13-184
- Chiow KH, Phoon MC, Putti T, et al. Evaluation of antiviral activities of Houttuynia cordata Thunb. extract, quercetin, quercetrin and cinanserin on murine coronavirus and dengue virus infection. Asian Pac J Trop Med. 2016;9(1):1–7.
- Johari J, Kianmehr A, Mustafa MR, et al. Antiviral activity of baicalein and quercetin against the Japanese encephalitis virus. Int J Mol Sci. 2012;13(12):16785–16795.10.3390/ijms131216785
- Lockhart JN, Stevens DM, Beezer DB, et al. Dual drug delivery of tamoxifen and quercetin: regulated metabolism for anticancer treatment with nanosponges. J Control Release. 2015;220:751–757.10.1016/j.jconrel.2015.08.052
- Chitkara D, Nikalaje SK, Mittal A, et al. Development of quercetin nanoformulation and in vivo evaluation using streptozotocin induced diabetic rat model. Drug Delivery Transl Res. 2012;2(2):112–123.10.1007/s13346-012-0063-5
- Chen LC, Chen YC, Su CY, et al. Development and characterization of self-assembling lecithin-based mixed polymeric micelles containing quercetin in cancer treatment and an in vivo pharmacokinetic study. Int J Nanomed. 2016;11:1557–1566.
- Beik J, Abed Z, Ghoreishi FS, et al. Nanotechnology in hyperthermia cancer therapy: from fundamental principles to advanced applications. J Control Release. 2016;235:205–221.10.1016/j.jconrel.2016.05.062
- Du H, Liu M, Yang X, et al. The role of glycyrrhetinic acid modification on preparation and evaluation of quercetin-loaded chitosan-based self-aggregates. J Colloid Interface Sci. 2015;460:87–96.10.1016/j.jcis.2015.08.049
- Liu C, Shan W, Liu M, et al. A novel ligand conjugated nanoparticles for oral insulin delivery. Drug Delivery. 2016;23(6):2015–2025.10.3109/10717544.2015.1058433
- Malathi S, Nandhakumar P, Pandiyan V, et al. Novel PLGA-based nanoparticles for the oral delivery of insulin. Int J Nanomed. 2015;10:2207–2218.
- Liao ZX, Chuang EY, Hsiao CW, et al. 21. pH-sensitive chitosan-based nanoparticles for protein drug delivery: oral approaches: Original research article: a novel pH-sensitive hydrogel composed of carboxymethyl chitosan and alginate cross-linked by genipin for protein drug delivery, 2004. J Control Release. 2014;190:68–70.
- Ralay-Ranaivo B, Desmaële D, Bianchini EP, et al. Novel self assembling nanoparticles for the oral administration of fondaparinux: synthesis, characterization and in vivo evaluation. J Control Release. 2014;194:323–331.10.1016/j.jconrel.2014.07.060
- Span K, Verhoef JJ, Hunt H, et al. A novel oral iron-complex formulation: encapsulation of hemin in polymeric micelles and its in vitro absorption. Eur J Pharm Biopharm. 2016;108:226–234.10.1016/j.ejpb.2016.09.002
- Dian L, Yu E, Chen X, et al. Enhancing oral bioavailability of quercetin using novel soluplus polymeric micelles. Nanoscale Res Lett. 2014;9(1):684.10.1186/1556-276X-9-684
- Mathot F, van Beijsterveldt L, Préat V, et al. Intestinal uptake and biodistribution of novel polymeric micelles after oral administration. J Control Release. 2006;111:47–55.10.1016/j.jconrel.2005.11.012
- Wang X, Chen Y, Dahmani FZ, et al. Amphiphilic carboxymethyl chitosan-quercetin conjugate with P-gp inhibitory properties for oral delivery of paclitaxel. Biomaterials. 2014;35(26):7654–7665.10.1016/j.biomaterials.2014.05.053
- Chen ZP, Sun J, Chen HX, et al. Comparative pharmacokinetics and bioavailability studies of quercetin, kaempferol and isorhamnetin after oral administration of Ginkgo biloba extracts, Ginkgo biloba extract phospholipid complexes and Ginkgo biloba extract solid dispersions in rats. Fitoterapia. 2010;81(8):1045–1052.10.1016/j.fitote.2010.06.028
- Sun M, Gao Y, Pei Y, et al. Development of nanosuspension formulation for oral delivery of quercetin. J Biomed Nanotechnol. 2010;6(4):325–332.10.1166/jbn.2010.1133
- Kim S, Shi Y, Kim JY, et al. Overcoming the barriers in micellar drug delivery: loading efficiency, in vivo stability, and micelle-cell interaction. Expert Opin Drug Delivery. 2010;7(1):49–62.10.1517/17425240903380446
- Salzano G, Costa DF, Sarisozen C, et al. Mixed nanosized polymeric micelles as promoter of doxorubicin and miRNA-34a co-delivery triggered by dual stimuli in tumor tissue. Small. 2016;12(35):4837–4848.10.1002/smll.v12.35
- Nakanishi T, Fukushima S, Okamoto K, et al. Development of the polymer micelle carrier system for doxorubicin. J Control Release. 2001;74(1–3):295–302.10.1016/S0168-3659(01)00341-8
- Cai X, Liu M, Zhang C, et al. pH-responsive copolymers based on pluronic P123-poly(β-amino ester): synthesis, characterization and application of copolymer micelles. Colloids Surf B. 2016;142:114–122.10.1016/j.colsurfb.2016.02.033
- Yang B, Guo C, Chen S, et al. Effect of acid on the aggregation of poly(ethylene oxide)−poly(propylene oxide)−poly(ethylene oxide) block copolymers. J Phys Chem B. 2006;110(46):23068–23074.10.1021/jp0634149
- Trauner M, Boyer JL. Bile salt transporters: molecular characterization, function, and regulation. Physiol Rev. 2003;83(2):633–671.10.1152/physrev.00027.2002
- Jin S, Fu S, Han J, et al. Improvement of oral bioavailability of glycyrrhizin by sodium deoxycholate/phospholipid-mixed nanomicelles. J Drug Target. 2012;20(7):615–622.10.3109/1061186X.2012.702770
- Van Hasselt PM, Janssens GE, Slot TK, et al. The influence of bile acids on the oral bioavailability of vitamin K encapsulated in polymeric micelles. J Control Release. 2009;133(2):161–168.10.1016/j.jconrel.2008.09.089
- Yu JN, Zhu Y, Wang L, et al. Enhancement of oral bioavailability of the poorly water-soluble drug silybin by sodium cholate/phospholipid-mixed micelles. Acta Pharmacol Sin. 2010;31(6):759–764.
- Wei Z, Hao J, Yuan S, et al. Paclitaxel-loaded pluronic P123/F127 mixed polymeric micelles: formulation, optimization and in vitro characterization. Int J Pharm. 2009;376(1–2):176–185.10.1016/j.ijpharm.2009.04.030
- Dabholkar RD, Sawant RM, Mongayt DA, et al. Polyethylene glycol–phosphatidylethanolamine conjugate (PEG–PE)-based mixed micelles: some properties, loading with paclitaxel, and modulation of P-glycoprotein-mediated efflux. Int J Pharm. 2006;315(1–2):148–157.10.1016/j.ijpharm.2006.02.018
- Liu M, Du H, Zhai G. Self-assembled nanoparticles based on chondroitin sulfate-deoxycholic acid conjugates for docetaxel delivery: effect of degree of substitution of deoxycholic acid. Colloids Surf B. 2016;146:235–244.10.1016/j.colsurfb.2016.06.019
- Luo S, Zhang Y, Cao J, et al. Arginine modified polymeric micelles as a novel drug delivery system with enhanced endocytosis efficiency. Colloids Surf B. 2016;148:181–192.10.1016/j.colsurfb.2016.07.023
- Zhang K, Zhang M, Liu Z, et al. Development of quercetin-phospholipid complex to improve the bioavailability and protection effects against carbon tetrachloride-induced hepatotoxicity in SD rats. Fitoterapia. 2016;113:102–109.10.1016/j.fitote.2016.07.008
- Liu Y, Yang J, Wang X, et al. In vitro and in vivo evaluation of redox-responsive sorafenib carrier nanomicelles synthesized from poly (acryic acid)-cystamine hydrochloride-D-α-tocopherol succinate. J Biomater Sci Polym Ed. 2016;27(17):1729–1747.10.1080/09205063.2016.1236883
- Li H, Zhao X, Ma Y, et al. Enhancement of gastrointestinal absorption of quercetin by solid lipid nanoparticles. J Control Release. 2009;133(3):238–244.10.1016/j.jconrel.2008.10.002
- Xu W, Ling P, Zhang T. Polymeric micelles, a promising drug delivery system to enhance bioavailability of poorly water-soluble drugs. J Drug Delivery. 2013;2013:15.
- Gaucher G, Dufresne MH, Sant VP, et al. Block copolymer micelles: preparation, characterization and application in drug delivery. J Control Release. 2005;109(1–3):169–188.10.1016/j.jconrel.2005.09.034
- Torchilin VP. Structure and design of polymeric surfactant-based drug delivery systems. J Control Release. 2001;73(2–3):137–172.10.1016/S0168-3659(01)00299-1
- Gong J, Chen M, Zheng Y, et al. Polymeric micelles drug delivery system in oncology. J Control Release. 2012;159(3):312–323.10.1016/j.jconrel.2011.12.012
- Lu Y, Park K. Polymeric micelles and alternative nanonized delivery vehicles for poorly soluble drugs. Int J Pharm. 2013;453(1):198–214.10.1016/j.ijpharm.2012.08.042
- Gaucher G, Satturwar P, Jones MC, et al. Polymeric micelles for oral drug delivery. Eur J Pharm Biopharm. 2010;76(2):147–158.10.1016/j.ejpb.2010.06.007
- Nam YS, Kang HS, Park JY, et al. New micelle-like polymer aggregates made from PEI-PLGA diblock copolymers: micellar characteristics and cellular uptake. Biomaterials. 2003;24(12):2053–2059.10.1016/S0142-9612(02)00641-5
- Zhang H, Zhao L, Chu L, et al. Preparation, optimization, characterization and cytotoxicity in vitro of Baicalin-loaded mixed micelles. J Colloid Interface Sci. 2014;434:40–47.10.1016/j.jcis.2014.07.045
- Zhao L, Du J, Duan Y, et al. Curcumin loaded mixed micelles composed of Pluronic P123 and F68: preparation, optimization and in vitro characterization. Colloids Surf B. 2012;97:101–108.10.1016/j.colsurfb.2012.04.017
- Liu W, Guo R. Interaction between flavonoid, quercetin and surfactant aggregates with different charges. J Colloid Interface Sci. 2006;302(2):625–632.10.1016/j.jcis.2006.06.045
- Xu G, Shi H, Ren L, et al. Enhancing the anti-colon cancer activity of quercetin by self-assembled micelles. Int J Nanomed. 2015;10:2051–2063.
- Zhang H, Yang X, Zhao L, et al. In vitro and in vivo study of Baicalin-loaded mixed micelles for oral delivery. Drug Delivery. 2016;23(6):1933–1939.
- Zhao L, Shi Y, Zou S, et al. Formulation and in vitro evaluation of quercetin loaded polymeric micelles composed of pluronic P123 and Da-tocopheryl polyethylene glycol succinate. J Biomed Nanotechnol. 2011;7(3):358–365.10.1166/jbn.2011.1298
- Pepić I, Lovrić J, Filipović-Grčić J. How do polymeric micelles cross epithelial barriers? Eur J Pharm Sci. 2013;50(1):42–55.
- Mu L, Teo MM, Ning HZ, et al. Novel powder formulations for controlled delivery of poorly soluble anticancer drug: application and investigation of TPGS and PEG in spray-dried particulate system. J Control Release. 2005;103(3):565–575.10.1016/j.jconrel.2004.12.023
- Kabanov AV, Batrakova EV, Miller DW. Pluronic® block copolymers as modulators of drug efflux transporter activity in the blood-brain barrier. Adv Drug Del Rev. 2003;55(1):151–164.10.1016/S0169-409X(02)00176-X
- Wang T, Wang N, Song H, et al. Preparation of an anhydrous reverse micelle delivery system to enhance oral bioavailability and anti-diabetic efficacy of berberine. Eur J Pharm Sci. 2011;44(1–2):127–135.10.1016/j.ejps.2011.06.015