Abstract
To identify yeasts involved in white-colony formation on Japanese commercial kimchi products, three types of kimchi were prepared and fermented at four different temperatures. At 4 °C, yeast colonies did not appear until 35 days, while more rapid white-colony formation occurred at higher temperatures (10, 15, and 25 °C). Combination of PCR-DGGE and direct isolation of yeasts from white colonies revealed that Kazachstania exigua and K. pseudohumilis were responsible for the white-colony formation. Inoculation of the isolated Kazachstania strains into fresh kimchi successfully reproduced white-colony formation at 15 °C but not at 4 °C. Growth experiments in liquid medium revealed that Kazachstania spp. grew fast at 15 °C even in the presence of acidulants, which are commonly added to Japanese kimchi products for prevention of yeast growth. These results suggest that white-colony formation on Japanese kimchi is caused by the genus Kazachstania, and that one of important factors determining white-colony formation is its fermentation temperature.
Kimchi inoculated with 102 CFU g−1 of Kazachstania pseudohumilis after fermentation at 15 °C for 21 days (left) or at 4˚C for 51 days (right).
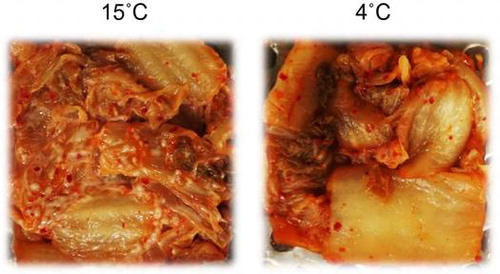
Kimchi is a traditional fermented food in Korea and is produced via fermentation of various salted vegetables such as Chinese cabbage, green onion, and radish, with a seasoning made from salt, sugar, garlic, ginger, red pepper powder, and fermented seafood [Citation1,2]. The raw materials of kimchi are not usually sterilized, and therefore kimchi fermentation is a natural process initiated by various microorganisms including lactic acid bacteria (LAB), yeasts, and aerobic bacteria originally present in the raw materials. In recent years, kimchi’s health-promoting characteristics such as antiobesity effects, cholesterol reduction, and skin health promotion have been reported [Citation2,3]. According to the Japanese Food Marketing Research and Information Center, annual production of kimchi in Japan was 192,177 tons in 2015, which is the largest among various pickled vegetables. In Japan, commercial kimchi products are mass-produced, and raw materials are partly sterilized to suppress fast growth of LAB. In addition, various weak organic acids are also added as acidulants to suppress undesirable microorganisms.
To date, a wide variety of LAB have been found in kimchi, e.g., Lactobacillus brevis, Lb. curvatus, Lb. plantarum, Lb. sakei, Leuconostoc carnosum, Lc. citreum, Lc. inhae, Lc. kimchi, Lc. gasicomitatum, Lc. gelidum, Lc. mesenteroides, Weissella kimchi, and W. koreensis [Citation4–8]. Lactic acid and acetic acid produced by LAB decrease pH of kimchi and are thought to suppress the growth of potentially putrefactive microorganisms such as aerobic bacteria and filamentous fungi. Several physicochemical and biological factors affect LAB community structure during kimchi fermentation, and these include fermentation temperature, fermentation period, salt concentration, and types of the seasoning. Among these factors, fermentation temperature strongly influences quality and shelf life of kimchi products by affecting the growth of LAB and the relative growth rate of each LAB group [Citation5,9]. Generally, kimchi is fermented at low temperatures (2–6 °C) to ensure proper ripening and preservation. At higher temperatures, for example, 10–20 °C, the growth of LAB is rapid and causes a significant decrease in pH, which is sometimes unacceptable for consumers. Thus, temperature control during kimchi fermentation, distribution, and storage is necessary to ensure organoleptic quality of kimchi products.
Not only LAB but also yeasts commonly inhabit kimchi. Yeasts are eukaryotic single-celled microorganisms classified as members of the phylum Ascomycota, and most of them reproduce asexually by budding. To date, yeasts belonging to genera Candida, Kazachstania, Pichia, Rhodotorula, and Saccharomyces have been isolated from kimchi [Citation10–12]. Chang et al. [Citation13] performed PCR-denaturing gradient gel electrophoresis (PCR-DGGE) analysis of yeast community structure in seven different commercial kimchi products and found that yeast community structure depends on the manufacturer. Although the proportion of yeast cells in kimchi (at most 106 colony-forming units [CFU] per gram) is significantly smaller than that of LAB cells (at most 108 CFU g−1), they may play significant roles in kimchi fermentation and its quality. It is also known that certain yeasts cause kimchi spoilage, which typically appears as an off-odor, texture-softening, expansion of packages, and white-colony or -film formation on the surface of products [Citation12]. In Japanese market, white-colony formation severely reduces the product value of kimchi and easily leads to complaint from consumers.
In contrast to LAB, there have been only limited attempts to isolate yeast strains from kimchi. In addition, it remains unclear how fermentation temperature affects the yeast community structure in kimchi. Information about undesirable yeasts causing white-colony formation on kimchi is still scarce. In this study, we fermented three types of Japanese kimchi at two temperatures (4 and 15 °C) and determined yeast population dynamics by both culture-dependent and -independent (PCR-DGGE) methods. In addition, to reproduce white-colony formation in a laboratory, kimchi was fermented at four different temperatures (4, 10, 15, and 25 °C) until white-colony formation. Finally, yeast strains were isolated directly from the surface of the kimchi and were inoculated into fresh kimchi to confirm their capacity for white-colony formation. The mechanism by which white-colony-forming yeasts predominate in kimchi is also discussed.
Materials and methods
Preparation and fermentation of kimchi
Chinese cabbage was cut, washed with sodium hypochlorite, and salted. The cabbage was then mixed with various seasonings including salt, sugar, garlic, ginger, red pepper powder, vinegar, onion, and fermented shrimp. A mixture of acidulants including sodium acetate, glacial acetic acid, fumaric acid, dl-malic acid, and thiamine lauryl sulfate was also added. To compare microbial population dynamics, we prepared three types of Japanese kimchi (kimchi A–C) with different seasoning combinations. Typical ingredients in kimchi A were apple juice, soy sauce, and wheat flour, whereas the main ingredient in kimchi C was sesame oil. Kimchi B contained much more garlic than kimchi A and C did. They were chosen because of their big sales. The expiration date of these kimchi products was 20 days. The prepared kimchi (1.5 kg) was packed into sterile 15-mL plastic tubes in 15-g portions. Kimchi was then fermented at 4 or 15 °C for 28 days. In another experiment, kimchi was fermented at various temperatures (4, 10, 15, or 25 °C) until formation of white colonies on the surface. In this case, the prepared kimchi was packed into cylindrical plastic containers with a bottom radius of 60 mm and a height of 30 mm. After 300 g of kimchi was packed, the containers were sealed with a heat-sensitive film, and fermented for 35 days at most.
Viable cell counts of yeast and LAB
During the fermentation, Japanese kimchi samples (15 g) were collected every 7 days, and were squeezed using sterile gauze. The pH level of kimchi soup (approximately 5 to 7 mL) was measured with a compact pH meter (HORIBA, Japan). The soup was serially diluted with sterile 0.8% NaCl, and then spread onto the YPD agar medium consisting of 10 g L−1 yeast extract, 20 g L−1 peptone, 20 g L−1 glucose, and 15 g L−1 agar. To prevent bacterial growth, 100 mg L−1 chloramphenicol and 50 mg L−1 ampicillin were also added. After 4 days of incubation at 15 °C, viable numbers of yeast cells were estimated as CFUs per gram of kimchi. Viable cell numbers of LAB were also determined by means of the GYP agar medium containing 10 mg L−1 each of sodium azide and cycloheximide as described previously [Citation14].
PCR-DGGE analysis of microbial community structure
Genomic DNA was extracted from kimchi soup using the FastDNA Spin Kit for Soil (MP Biomedicals, Solon, OH, USA). For analysis of yeast community structure, the D1/D2 domain of the 26S ribosomal RNA (rRNA) gene was amplified. The PCR was conducted with the GC-clamped forward primer NL-1 (5′- CGCCCGCCGCGCGGCGGGCGGGGCGGGGGCGCATATCAATAAGCGGAGGAAAAG-3′) and reverse primer LS-2 (5′-ATTAAACAACTCGACTC-3′) [Citation13]. The amplification was carried out in a standard reaction mixture containing 1 × PCR Gold buffer (MgCl2 free), 0.2 mM dNTP mixture, 1.5 mM MgCl2, 25 mU μL−1 of AmpliTaq Gold (Thermo Fisher Scientific, Waltham, MA, USA), each primer at 0.4 pM, and the DNA template of 50 ng. The PCR program was as follows: 1 cycle at 95 °C for 5 min; 30 cycles at 95 °C for 1 min, 52 °C for 1 min, and 72 °C for 2 min; and final extension at 72 °C for 7 min. The PCR product (approximately 150 bp) was confirmed on a 1% agarose gel.
In the analysis of kimchi fermented at 4 °C, no obvious PCR bands were observed probably due to low abundance of yeasts in the kimchi. In this case, two-step nested PCR was run [Citation13]. The first PCR was conducted with the forward primer NL-1 (5′-GCATATCAATAAGCGGAGGAAAAG-3′) and reverse primer NL-4 (5′-GGTCCGTGTTTCAAGACGG-3′). The PCR program was as follows: 1 cycle at 95 °C for 10 min; 30 cycles at 95 °C for 1 min, 50 °C for 45 s, 72 °C for 1 min; and final extension at 72 °C for 10 min. The amplicon (approximately 600 bp) from the first PCR was further amplified in the second PCR using the GC-clamped forward primer NL-1 and reverse primer LS-2 as described above.
DGGE was performed using a DCode Universal Mutation Detection System (Bio-Rad Laboratories, Hercules, CA, USA) at 140 V for 6 h at a constant temperature of 60 °C. After the electrophoresis, the gels were stained with ethidium bromide for 15 min. The major bands were excised and used for reamplification with primers NL1 and LS2. The sequences of the reamplified DNA fragments were determined using a DNA sequencing kit (Big Dye Terminator V3.1, Applied Biosystems, Carlsbad, CA, USA) and a DNA sequencer (ABI PRISM 3100 Genetic Analyzer, Applied Biosystems). The sequences were aligned with known sequences in the DNA Data Bank of Japan (DDBJ)/European Molecular Biology Laboratory (EMBL)/GenBank databases by means of the Basic Local Alignment Search Tool (BLAST) software (http://www.ncbi.nlm.nih.gov/BLAST/).
For analysis of bacterial community structure, the variable V3 region of the 16S rRNA gene was amplified with primers 338fGC and 518r as described elsewhere [Citation15].
Isolation and identification of yeast strains
Yeast colonies were picked randomly on the YPD agar medium and were purified by repetitive streaking on the same medium at 15 °C. The genomic DNA of the isolates was extracted as described previously [Citation16]. The D1/D2 domain of the isolates was analyzed by means of PCR primers NL-1 and NL-4 as described above. The yeast strains causing white-colony formation were isolated directly from the white colonies that appeared on the surface of the kimchi.
Fermentation of kimchi inoculated with yeast strains
Strains of Candida sake (WG04-1 and WG04-4), Kazachstania exigua (WH25-4 and WG15-1), K. pseudohumilis (WH10-1 and WY25-4), and Pichia fermentans (WH25-3 and WH25-6) isolated from the kimchi were grown statically in 5 mL of the liquid YPD medium at 15 °C for 2 days. Before inoculation of the yeast strains, freshly prepared kimchi A was heated at 65 °C for 1 h to eliminate indigenous yeasts and LAB. The absence of these microorganisms after the heat treatment was confirmed by spreading samples on YPD and GYP media. Thirty-gram samples of the heat-treated kimchi A were then dispensed into sterile plastic dishes, and inoculated with one of yeast cultures to attain the final yeast viable cell count of 102 CFU g−1. The inoculated kimchi A was fermented at 4 °C for 51 days or at 15 °C for 21 days.
Growth experiment
Yeast strains were pregrown statically in 10 mL of the YPD liquid medium at 15 °C for 2 days. Next, 100 μL of each preculture was inoculated into 10 mL of the same medium and incubated statistically at 4 or 15 °C for 14 days. The growth of the yeast strains at the two temperatures was measured by optical density at 600 nm, and growth rates (μ) were calculated. In some cases, a mixture of acidulants was added at final concentrations of 0.2 to 0.4% (w/v). The mixture (powder form) consisted of sodium acetate (52%), glacial acetic acid (20%), fumaric acid (12%), and dl-malic acid (12%), thiamine lauryl sulfate (2%), and cyclodextrin (3%).
Nucleotide sequence accession numbers
The gene sequences identified in this study were deposited in the DDBJ/EMBL/GenBank databases under the accession numbers LC219450 to LC219514.
Results
The numbers of yeast and LAB cells during fermentation
The pH change during fermentation of Japanese kimchi at 4 and 15 °C is shown in Figure (A). The initial pH of all types of kimchi was 4.8 and did not change significantly at 4 °C even after the fermentation for 28 days. In contrast, at the fermentation temperature of 15 °C, pH decreased rapidly to 3.8 within 14 days.
Figure 1. Changes in pH (A) and viable cell counts of LAB (B) and yeast (C) in kimchi A (circles), kimchi B (triangles), and kimchi C (squares). Kimchi was fermented at 4 °C (open symbols) or 15 °C (filled symbols) for 28 days. Viable cell counts of LAB and yeast were determined with GYP and YPD agar media, respectively.
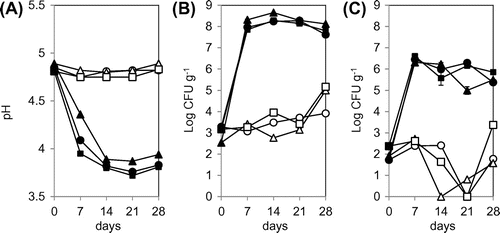
The initial viable cell count of LAB in the three types of kimchi was 1.3 ± 0.67 × 103 CFU g−1 (Figure (B)). The number of LAB cells increased slowly at fermentation temperature 4 °C, and final viable cell counts were 0.8 × 104 to 1.4 × 105 CFU g−1 at 28 days. In contrast, at 15 °C, the number of LAB cells increased more rapidly to 0.7 to 2.0 × 108 CFU g−1 within 7 days.
The initial viable cell count of yeast cells was 1.2 ± 0.8 × 102 CFU g−1 in all kimchi types (Figure (C)). At 4 °C, the number of yeast cells decreased gradually and was below the detection limit of 0.3 CFU g−1 after the fermentation for 14 to 21 days. Nonetheless, the number of yeast cells increased again and reached 3 × 101 to 2.3 × 103 CFU g−1 at 28 days. At 15 °C, the number of yeast cells increased rapidly to 2 to 4 × 106 CFU g−1 within 7 days, and afterwards, it remained stable.
PCR-DGGE analysis of microbial community in kimchi
To examine the changes in the LAB communities during the fermentation of kimchi, PCR-DGGE analysis of the 16S rRNA gene was performed (Supplementary Figure S1 and Table S1). At 4 °C, no distinct band was observed until fermentation day 21, but the bands closely related to Lb. heilongjiangensis (kimchi A and C), Lb. sakei (kimchi B), Lb. curvatus (kimchi B and C), and Lb. plantarum (kimchi C) appeared at 28 days. At 15 °C, multiple bands of relatively strong intensity emerged after the fermentation for 7 days in the analysis of all kimchi types, and they were found to be closely related to Lb. plantarum and Lb. brevis.
Similar PCR-DGGE analysis was performed on the yeast 26S rRNA gene (Figure and Table ). Because noticeable rRNA gene fragments failed to be amplified from the samples of kimchi fermented at 4 °C, two-step nested PCR was carried out prior to DGGE analysis. As shown in Figure , multiple DGGE bands including those of plant origin were observed at 4 °C. Sequencing of these bands showed that they belong to members of genera Candida, Debaryomyces, Geotrichum, Pichia, and Trichosporon (Table ). At the fermentation temperature of 15 °C, only one major band (corresponding to bands f, o, and w in Figure ) was observed. Sequencing of this band revealed that it was closely related to K. exigua or K. pseudohumilis with sequence similarities of 100%. Because these yeast species are phylogenetically very close, they could not be discriminated by means of the relatively short (approximately 150 bp) nucleotide sequences of the DGGE band.
Figure 2. PCR-DGGE analysis of yeast communities in three types of kimchi fermented at 4 or 15 °C. Bands labeled with letters were excised and their DNA sequences were determined for identification of yeasts (see Table ). The numbers above each lane represent the fermentation period (days).
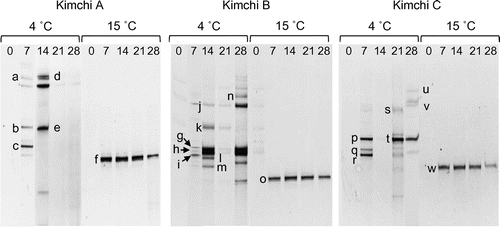
Table 1. Sequence analysis of 26S rRNA genes recovered from DGGE bands.
Isolation and identification of yeast strains from kimchi
In the above experiment, yeast strains were routinely isolated from kimchi by means of the YPD agar medium and were identified on the basis of 26S rRNA gene (D1/D2 region) sequences. Twenty-four strains were successfully isolated from kimchi fermented at 4 °C, among which 19 strains were identified as D. hansenii. The other five strains were affiliated with Candida picinguabensis (two strains), C. sake (one strain), P. fermentans (one strain), and T. moniliforme (one strain). Twenty strains were isolated from kimchi fermented at 15 °C. All these strains were affiliated with K. exigua, suggesting that the major bands (f, o, and w) observed in the PCR-DGGE analysis belonged to K. exigua.
Reproduction of white-colony formation on kimchi
To reproduce white-colony formation in the laboratory, three types of kimchi were fermented at four temperatures (4, 10, 15, and 25 °C). At 10 °C, white colonies appeared after fermentation for 20 days, whereas they appeared within 5 days at fermentation temperatures of 15 and 25 °C (Supplementary Figure S2). At 4 °C, yeast colonies were not observed until the expiration date of 20 days. After a much longer fermentation period of 35 days, white colonies appeared only on kimchi C. In kimchi with white colonies, more than 106 CFU g−1 of yeast cells were detected. Nonetheless, white-colony formation did not take place when viable cell counts of yeast were less than 105 CFU g−1 (data not shown).
DGGE banding patterns for the yeast communities in kimchi forming white colonies are shown in Figure . In the kimchi fermented at 10, 15, and 25 °C, only one major band affiliated with K. exigua or K. pseudohumilis was observed (band a). In contrast, a band closely related to C. sake was observed during analysis of kimchi C fermented at 4 °C (band b). For kimchi C fermented at 25 °C, a band of P. fermentans (band c) was also observed.
Figure 3. PCR-DGGE analysis of yeast communities in three types of kimchi after white-colony formation. Kimchi was fermented at 4, 10, 15, or 25 °C until white colonies appeared on the surface. Note that white-colony formation at 4 °C was detected only in kimchi C. Bands labeled with letters were excised, and their DNA sequences were determined for identification of yeasts (see text). Numbers above each lane represent fermentation temperature (°C).
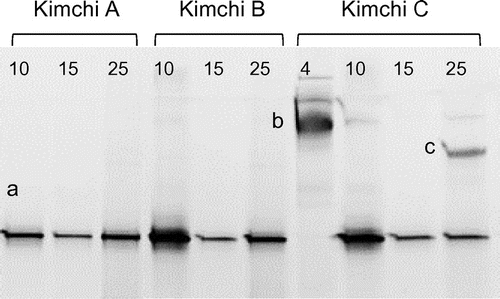
A total of 43 yeast strains were isolated directly from white colonies that appeared on the surface of kimchi. As shown in Table , K. exigua was most frequently isolated from the kimchi fermented at 15 and 25 °C. At 25 °C, six strains of P. fermentans were also isolated. At 10 °C, K. pseudohumilis was isolated preferentially, although K. exigua was still present as a minor population. At 4 °C, only strains of C. sake were isolated.
Table 2. Yeast strains isolated directly from white colonies formed on kimchi surface.
White-colony formation on kimchi inoculated with yeast strains
Yeast strains (C. sake, K. exigua, K. pseudohumilis, and P. fermentans) isolated from the kimchi were inoculated into fresh kimchi A with an initial population size of 102 CFU g−1, and the inoculated kimchi A was fermented at 4 or 15 °C. After the fermentation at 15 °C for 21 days, a large number of white colonies appeared on the surface of kimchi inoculated with K. exigua or K. pseudohumilis (Figure (B) and (C)). In contrast, no noticeable colonies were observed on the kimchi inoculated with C. sake or P. fermentans (data not shown) as well as in the control kimchi without inoculation (Figure (A)). In kimchi forming white colonies, approximately 108 CFU g−1 of yeast cells were detected after the white-film formation, but no detectable yeast was observed in unspoiled kimchi. At a fermentation temperature of 4 °C, no white-colony formation occurred on any kimchi even after fermentation for 51 days (Figure (D)–(F)).
Figure 4. White-colony formation on the surface of kimchi A inoculated with yeast strains. Freshly prepared kimchi A was heat-treated and inoculated with 102 CFU g−1 of Kazachstania exigua (B and E) or K. pseudohumilis (C and F). Control kimchi without yeast inoculation is also shown (A and D). The kimchi was incubated at 15 °C for 21 days (panels A–C) or at 4 °C for 51 days (D–F). Two strains were inoculated individually for each yeast species, but essentially the same results were obtained. White arrows indicate the yeast colonies.
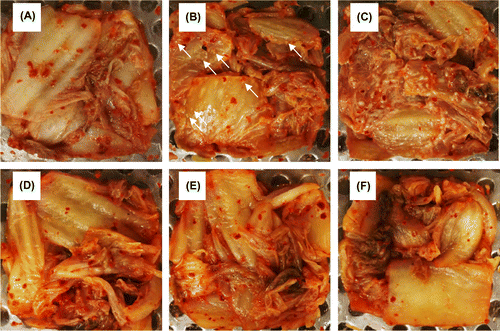
Growth of yeast strains at 4 and 15 °C
Among the yeast strains isolated in this study, one or two representative strains from each yeast species were chosen. They were grown in the YPD liquid medium for 14 days at 4 or 15 °C. As shown in Table , the growth rates (μ) of K. exigua and K. pseudohumilis at 15 °C were the highest among the seven species. In contrast, these two species and C. picinguabensis did not show significant growth at 4 °C, whereas the remaining four species still grew slowly at 4 °C. Growth of D. hansenii, T. moniliforme, and C. picinguabensis was strongly suppressed or inhibited completely by the addition of a mixture of acidulants (Table ), which is commonly used to suppress growth of yeasts in commercial Japanese kimchi. However, K. exigua, K. pseudohumilis, C. sake, and P. fermentans still showed significant growth even in the presence of 0.4% of the acidulants mixture.
Table 3. The growth rates (μ) of yeast strains at 4 and 15 °C in YPD medium.Table Footnotea
Discussion
In this study, we determined the effect of fermentation temperature on yeast and LAB community structures in Japanese kimchi by both culture-dependent and -independent methods. At the fermentation temperature of 4 °C, pH of all kimchi types did not decrease from the initial pH value of 4.8 probably owing to the growth suppression of LAB at this temperature (Figure ). This phenomenon is desirable particularly for the Japanese pickled vegetable market because kimchi with strong acidity (below pH 4.3) is not acceptable to most of Japanese consumers. By contrast, when fermentation temperature was raised to 15 °C, the decrease in pH was rapid as a result of LAB growth and the consequent acid production. Our results clearly indicate that an appropriate control of fermentation temperature is necessary to prevent excess acidity of kimchi products.
At the fermentation temperature of 4 °C, the number of yeast cells in all kimchi types stayed low, in most cases less than 103 CFU g−1, throughout the fermentation period of 28 days (Figure (C)). DGGE bands closely related to C. picinguabensis, C. sake, D. hansenii, Geotrichum fragrans, P. fermentans, Trichosporon middelhovenii, and T. moniliforme were detected (Figure and Table ), and most of these yeast species have been found in various fermented foods such as cider (C. sake) [Citation17], cheese (D. hansenii) [Citation18], fermented cereal beverages (G. fragrans) [Citation19], fermented milk (P. fermentans) [Citation20], and kimchi (Trichosporon spp.) [Citation13]. Although yeast community structure was found to be different among the three types of kimchi, DGGE bands corresponding to D. hansenii were always detected in all kimchi types. Actually, 19 out of 24 yeast strains isolated from kimchi fermented at 4 °C were identified as D. hansenii. This yeast is halotolerant and can grow in the presence of more than 10% NaCl [Citation21]. In addition, growth of D. hansenii between 5 and 10 °C and even below 0 °C has been reported [Citation22]. These data suggest that D. hansenii is one of the predominant yeast species in kimchi fermented at low temperatures, probably because of its halotolerance and psychrotolerance.
When kimchi was fermented at 15 °C, the diversity of yeast community structure decreased dramatically, and only one DGGE band corresponding to K. exigua or K. pseudohumilis was observed (Figure ). In addition, only strains of K. exigua could be isolated from the kimchi, indicating that this yeast became predominant at this temperature. K. exigua is a synonym of Saccharomyces exiguus and Candida holmii and has been isolated from diverse food sources including buttermilk, fermented cucumber brine, sauerkraut, grape must, and sourdough [Citation23]. When Chang et al. [Citation13] determined yeast community structure in eight types of commercial kimchi, three out of eight products were dominated by Kazachstania unispora (Saccharomyces unisporus). In addition, Moon et al. [Citation12] isolated K. bulderi, K. exigua, and K. servazzii from over-ripened kimchi. Therefore, it is possible that Kazachstania spp. are distributed widely in kimchi that is being fermented and play an important role in kimchi spoilage. Although we did not determine any spoilage factors such as hardness and volatile compounds in this study, kimchi covered with Kazachstania spp. displayed strong sour- and rotten-odors.
The combination of PCR-DGGE and direct isolation of yeast strains revealed that white-colony formation on kimchi was caused by specific groups of yeast including C. sake, K. exigua, K. pseudohumilis, and P. fermentans (Figure and Table ). Among them, strains of C. sake were isolated frequently from the kimchi fermented at 4 °C, but not from the kimchi fermented at 15 or 25 °C. Because C. sake is well-known as a representative psychrophilic yeast [Citation24], it may be involved in white-colony formation on kimchi fermented at 4 °C. In contrast, DGGE bands corresponding to K. exigua or K. pseudohumilis were routinely observed in almost all spoiled kimchi. K. exigua can grow at 30 °C, whereas the maximal growth temperature of K. pseudohumilis is 28 °C [Citation25], suggesting that the latter species is more psychrophilic. Consistent with these observations, strains of K. pseudohumilis were isolated frequently from the kimchi fermented at 10 °C, whereas K. exigua was isolated predominantly from the kimchi fermented at 15 or 25 °C (Table ). Thus, there seems to be niche segregation between these two Kazachstania species in kimchi, probably because of differences in adaptation to cold temperature.
Recently, Moon et al. [Citation12] isolated yeast strains from over-ripened kimchi, on which white-colony formation was observed. Some of these strains were inoculated into fresh kimchi, and Pichia kudriavzevii was found to cause white-colony formation, strong off-odors, moldy odors, and texture softening. Although they inoculated 107 CFU g−1 of yeast strains into kimchi, our inoculum size was much smaller (102 CFU g−1) in order to adjust yeast population size to that at the beginning of fermentation (Figure (C)). As a consequence, white-colony formation by K. exigua and K. pseudohumilis could be reproduced successfully at 15 °C but not at 4 °C. This finding is consistent with the fact that both K. exigua and K. pseudohumilis grew in a liquid medium at 15 °C but not at 4 °C (Table ). Thus, it is likely that one of physicochemical factors determining white-colony formation on kimchi is its fermentation temperature. In contrast, neither C. sake nor P. fermentans could form white colonies regardless of fermentation temperature, even though they showed significant growth rates at 4 and 15 °C (Table ). Therefore, there seems to be another physicochemical or biological factor controlling white-colony formation by these yeasts. Given that white-colony formation by C. sake took place only on kimchi C, certain ingredients (such as sesame oil) present in kimchi C may be required for predominant growth of C. sake in kimchi.
In commercial Japanese kimchi production, it is common to add a mixture of acidulants to suppress undesirable microorganisms and to improve shelf life of the products. Such mixtures usually consist of various weak organic acids such as sodium acetate, glacial acetic acid, fumaric acid, and dl-malic acid together with vitamin B1 derivatives such as thiamine lauryl sulfate. In this study, growth of certain yeasts was found to be highly resistant to the mixture of acidulants (Table ). In particular, both K. exigua and K. pseudohumilis grew well even in the presence of 0.4% of the mixture. Thus, it may be possible that resistance to acidulants is another biological factor determining white-colony formation on kimchi. Further study is needed to understand the mechanism by which specific yeasts acquire resistance to organic acids and thiamine lauryl sulfate.
In conclusion, we found that yeasts belonging to genus Kazachstania are mainly responsible for white-colony formation on Japanese kimchi, and that fermentation temperature is one of the important factors controlling the spoilage of kimchi. After white-colony formation, the yeast colonies spread gradually on the kimchi surface (white-film formation) and eventually led to off-odor formation and texture softening (data not shown). In contrast, most of yeast species cannot form white colonies on kimchi fermented at 4 °C, and diverse psychrotolerant yeasts such as D. hansenii coexist at this temperature. Although only limited information is still available on whether such psychrotolerant yeast participate in kimchi fermentation and affect its quality, it would be interesting to select a beneficial yeast strain and apply it as a starter to improve the quality of kimchi. Alternatively, it is possible to use such beneficial yeast as an antagonist to prevent growth of undesirable white-colony-forming yeasts in kimchi. Screening of such yeast strains is underway in our laboratory.
Author contribution
S.A. and A.S. conceived and designed the study. A.S. and N.M. performed the experiments. S.A. and A.S. drafted the manuscript. M.N. and Y.Y. offered counsels on the study and reviewed the manuscript.
Disclosure statement
No potential conflict of interest was reported by the authors.
Supplemental data
Supplemental data for this article can be accessed https://doi.org/10.1080/09168451.2017.1419853.
Supplemental_data.pdf
Download PDF (4.5 MB)References
- Jung JY, Lee SH, Jeon CO. Kimchi microflora: history, current status, and perspectives for industrial kimchi production. Appl Microbiol Biotechnol. 2014;98:2385–2393.10.1007/s00253-014-5513-1
- Patra JK, Das G, Paramithiotis S, et al. Kimchi and other widely consumed traditional fermented foods of Korea: a review. Front Microbiol. 2016;7:1493.
- Park KY, Jeong JK, Lee YE, et al. Health benefits of kimchi (Korean fermented vegetables) as a probiotic food. J Med Food. 2014;17:6–20.10.1089/jmf.2013.3083
- Cho J, Lee D, Yang C, et al. Microbial population dynamics of kimchi, a fermented cabbage product. FEMS Microbiol Lett. 2006;257:262–267.10.1111/fml.2006.257.issue-2
- Hong Y, Yang H-S, Chang H-C, et al. Comparison of bacterial community changes in fermenting kimchi at two different temperatures using a denaturing gradient gel electrophoresis analysis. J Microbiol Biotechnol. 2013;23:76–84.10.4014/jmb
- Kim M, Chun J. Bacterial community structure in kimchi, a Korean fermented vegetable food, as revealed by 16S rRNA gene analysis. Int J Food Microbiol. 2005;103:91–96.10.1016/j.ijfoodmicro.2004.11.030
- Lee JS, Heo GY, Lee JW, et al. Analysis of kimchi microflora using denaturing gradient gel electrophoresis. Int J Food Microbiol. 2005;102:143–150.10.1016/j.ijfoodmicro.2004.12.010
- Park E-J, Chun J, Cha C-J, et al. Bacterial community analysis during fermentation of ten representative kinds of kimchi with barcoded pyrosequencing. Food Microbiol. 2012;30:197–204.10.1016/j.fm.2011.10.011
- Mheen T-I, Kwon T-W. Effect of temperature and salt concentration on Kimchi fermentation. Korean J Food Sci Technol. 1984;16:443–450.
- Jeong SH, Jung JY, Lee SH, et al. Microbial succession and metabolite changes during fermentation of dongchimi, traditional Korean watery kimchi. Int J Food Microbiol. 2013;164:46–53.10.1016/j.ijfoodmicro.2013.03.016
- Jeong SH, Lee SH, Choi EJ, et al. Microbial succession and metabolite changes during long-term storage of kimchi. J Food Sci. 2013;78:M763–M769.10.1111/1750-3841.12095
- Moon SH, Chang M, Kim HY, et al. Pichia kudriavzevii is the major yeast involved in film-formation, off-odor production, and texture-softening in over-ripened Kimchi. Food Sci Biotechnol. 2014;23:489–497.10.1007/s10068-014-0067-7
- Chang HW, Kim KH, Nam YD, et al. Analysis of yeast and archaeal population dynamics in kimchi using denaturing gradient gel electrophoresis. Int J Food Microbiol. 2008;126:159–166.10.1016/j.ijfoodmicro.2008.05.013
- Kozaki M, Uchimura T, Okada S. Experimental manual of lactic acid bacteria. Tokyo: Asakurasyoten; 1992. p. 29–72.
- Muyzer G, de Waal EC, Uitterlinden AG. Profiling of complex microbial populations by denaturing gradient gel electrophoresis analysis of polymerase chain reaction-amplified genes coding for 16S rRNA. Appl Environ Microbiol. 1993;59:695–700.
- Hiraishi A. Direct automated sequencing of 16S rDNA amplified by polymerase chain reaction from bacterial cultures without DNA purification. Lett Appl Microbiol. 1992;15:210–213.10.1111/j.1472-765X.1992.tb00765.x
- Coton E, Coton M, Levert D, et al. Yeast ecology in French cider and black olive natural fermentations. Int J Food Microbiol. 2006;108:130–135.10.1016/j.ijfoodmicro.2005.10.016
- Capece A, Romano P. ‘Pecorino di Filiano’ cheese as a selective habitat for the yeast species, Debaryomyces hansenii. Int J Food Microbiol. 2009;132:180–184.10.1016/j.ijfoodmicro.2009.04.007
- Osimani A, Garofalo C, Aquilanti L, et al. Unpasteurised commercial boza as a source of microbial diversity. Int J Food Microbiol. 2015;194:62–70.10.1016/j.ijfoodmicro.2014.11.011
- Qvirist LA, de Filippo C, Strati F, et al. Isolation, identification and characterization of yeasts from fermented goat milk of the Yaghnob Valley in Tajikistan. Front Microbiol. 2016;7:1690.
- Prista C, Michán C, Miranda IM, et al. The halotolerant Debaryomyces hansenii, the Cinderella of non-conventional yeasts. Yeast. 2016;33:523–533.10.1002/yea.3177
- Breuer U, Harms H. Debaryomyces hansenii –an extremophilic yeast with biotechnological potential. Yeast. 2006;23:415–437.10.1002/(ISSN)1097-0061
- Vaughan-Martini A, Lachance M-A, Kurtzman CP. The yeasts, a taxonomic study. 5th ed. 2011. Chapter 34, Kazachstania Zubkova (1971); p. 439–470.
- Lachance M-A, Boekhout T, Fell JW, et al. The yeasts, a taxonomic study. 5th ed. 2011. Chapter 90, Candida Berkhout (1923); p. 987–1278.
- Jacques N, Sarilar V, Urien C, et al. Three novel ascomycetous yeast species of the Kazachstania clade, Kazachstania saulgeensis sp. nov., Kazachstania serrabonitensis sp. nov. and Kazachstania australis sp. nov. Reassignement of Candida humilis to Kazachastania humilis f. a. comb. nov. and Candida pseudohumilis to Kazachstania pseudohumilis f. a. comb. nov. Int J System Evol Microbiol. 2016;66:5192–5200.