Abstract
Dry eye syndrome (DES) is considered as an ocular surface inflammatory disease. Previous studies have shown inflammation plays an important role in the progression and onset of DES. Co-culture of human bone marrow mesenchymal stem cells (HBMSCs) and macrophages showed immunomodulatory effects via regulation of cytokine regulation. Thus, the aim of this study was to investigate the effect of the interaction of these cells on in vitro DES model. The conditioned media (CM) from macrophages, HBMSCs, and HBMSCs + macrophages were treated to human corneal epithelial cells, which showed significant reduction in IL-1α and IL-1β expression levels in HBMSCs + macrophages group. Moreover, the IL-1 Receptor Antagonist (IL-1RA) was highly expressed in the CM from the HBMSCs + macrophages group. Wounded eyes of mice were treated with IL-1RA at 0–100 ng/mL for 16 h, the wound size was reduced. The results of this study might lead to the identification of new therapeutic targets for DES.
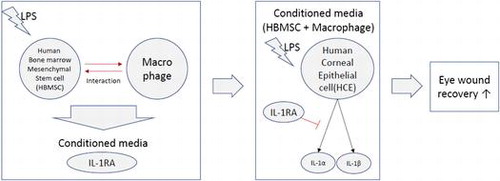
Conditioned media from co-cultured HBMSCs and macrophages had a synergistic immunomodulatory effect on corneal epithelial cells.
Abbreviations:
- DES: dry eye syndrome
- MSCs: mesenchymal stem cells
- HBMSCs: human bone marrow mesenchymal stem cells
- HCE cells: human corneal epithelial cells
- CM:conditioned media
- RA: Receptor Antagonist
- FBS:fetal bovine serum
- PMA: Phorbol-12-myristate-13-acetate
- LPS: Lipopolysaccharide
- SEC: size exclusion chromatography
- QTOF-LC-MS/MS: quadrupole time of flight tandem mass spectroscopy
- ELISA: enzyme linked immunosorbent assay
- RPMI: Roswell Park Memorial Institute
- DMEM: Dulbecco’s modified Eagle’s medium
- DMSO: dimethyl sulfoxide
- PBS: phosphate-buffered saline
- FACS: fluorescence-activated cell sorting
Dry eye syndrome (DES) significantly affects quality of life by not only deteriorating functional vision, but also impairing routine activities, including driving, reading, and watching television [Citation1]. Symptoms of DES are eye irritation, blurred vision, tear film instability, increased tear osmolarity, and ocular surface epithelial disease [Citation2,3]. Recently, there have been increasing reports that DES is an inflammatory disease. DES affects tear composition, for instance, it induces hyperosmolarity, which prompts the expression of inflammatory factors on the ocular surface [Citation4]. Inflammation could induce dysfunction of tear secretion or retention [Citation5]. These sequential events lead to a vicious cycle of inflammatory reaction, resulting in ocular surface disease [Citation6].
Clinical evidence demonstrated that anti-inflammatory therapies that inhibit these inflammatory mediators could attenuate the symptoms. Anti-inflammatory agents are also very important for managing DES to suppress inflammation and to prevent scarring on the ocular surface. Corticosteroids are effective as anti-inflammatory agents for DES [Citation7], however, their use is associated with problems such as risk of infection from their immunosuppressive effects, stromal melting, and potential toxicity from long-term use. Therefore, a new anti-inflammation therapy for DES is required.
Macrophages are broadly distributed in the human body and plays critical role in innate immunity. When inflammatory triggers are fired by various causes such as injury and infection, circulating peripheral blood monocytes migrate into tissues, and differentiate into macrophages [Citation8]. In case of cornea, the monocytes in the corneal peripheral blood vessel could migrate into corneal tissue. It is well-known that there are two types of macrophages, classically activated macrophages (M1) and alternatively activated macrophages (M2) [Citation9]. Macrophages can shift between pro-inflammatory M1 and tissue-remodeling M2, and this shift plays important role in tissue regeneration [Citation10–12].
Mesenchymal stem cells (MSCs) has potential capability to regenerate damaged tissue, and it is speculated that their capability is from immunological regulation or vascular neogenesis [Citation13] . These cells manipulate and change macrophage to alternatively activated form and is expected to play a role in tissue repair [Citation14]. Moreover, it was shown that allogeneic MSCs inhibited corneal inflammation and neovascularization through paracrine mechanism [Citation15], and MSC-induced therapeutic effects of tissue repair are by secreting soluble factors that modulate inflammation and angiogenesis [Citation16].
Recent studies have been investigated the interactions of MSCs with immune cells including T lymphocytes, natural killer cells, and dendritic cells [Citation17], however, many aspects of the interactions of MSCs with macrophages are not revealed yet.
Based on these studies, we designed in vitro DES model, using LPS-treated HCE cells cultured under conditioned media (CM) from co-cultured HBMSCs and macrophages in order to examine their interaction. In addition, we analyzed soluble factors in the co-cultured CM by size exclusion chromatography (SEC) and quadrupole time of flight tandem mass spectroscopy (QTOF LC-MS/MS).
Materials and methods
Cell culture
HBMSCs were purchased from Cambrex (Walkersville, MD, USA). HBMSCs were cultured in minimal essential medium (α-MEM; GIBCO, Waltham, MA, USA) with 10% fetal bovine serum (FBS), penicillin G, and streptomycin at 37 °C in a humidified atmosphere containing 95% air and 5% CO2. All experiments were carried out with HBMSCs in the fourth passage. The THP-1 monocyte cell line was purchased from the American Type Culture Collection (Manassas, VA, USA). The cells were cultured in Roswell Park Memorial Institute (RPMI) 1640 medium containing 10% heat-inactivated FBS, 10 mM HEPES, 2 mM glutamine, penicillin, and streptomycin, and maintained at 37 °C under 5% CO2. Human corneal epithelial (HCE) cells were purchased from the American Type Culture Collection and cultured in Dulbecco’s modified Eagle’s medium (DMEM)/F12 medium supplemented with 10% FBS and 5 ng/mL human recombinant epidermal growth factor at 37 °C with 5% CO2. Phorbol-12-myristate-13-acetate (PMA) was solubilized in 0.1% dimethyl sulfoxide (DMSO).
THP-1 differentiation
THP-1 cells were seeded in 12-well plates. The cells were incubated with PMA at 37 °C. The living cells were counted in a hemocytometer by trypan blue staining after 24, 48, and 72 h. Each experiment was performed in triplicate. In addition, the THP-1 cells were plated into a 60 mm dish (1 × 106 cells) and treated with PMA (100 nM) for 48 h. The shape of the cells was observed to confirm that the cells were differentiated into macrophages. To identify CD14, which is a surface marker of macrophage differentiation, the differentiated cells were washed three times with phosphate-buffered saline (PBS) and trypsinized. The trypsinized cells were incubated with an Fc blocking reagent for 30 min, followed by incubation with fluorescein isothiocyanate-conjugated anti-human CD14 (eBioscience, San Diego, CA, USA) for 30 min on ice. After three washes with PBS, the cells were resuspended in 200 μL of PBS and then analyzed by flow cytometry using a FACScan flow cytometer (Becton Dickinson, CA, USA). Isotype-matched, monoclonal antibody-stained cells were used as the background control in all experiments.
Co-culture and treatment with co-culture CM
Differentiated macrophages were plated onto the HBMSCs (after they reached 80% confluency), at an HBMSC-to-macrophage ratio of 1:1, and co-cultured for 24 h. Lipopolysaccharide (LPS) was added to the co-cultured cells to stimulate the in vitro inflammatory response [Citation18]. During the 24 h culture, there was no change in the morphology of HBMSCs and macrophage cells. The groups were as follows: macrophages alone, HBMSCs alone, and HBMSCs + macrophages. The cell culture supernatants were carefully harvested from each culture, filtered with 0.2 μm filters, and separated into greater than 3 kDa (≥3 kDa) and less than 3 kDa (≤3 kDa) fractions, using a Microcon Centrifugal Filter Device (Millipore, USA) according to the manufacturer’s protocol. The cell-free supernatant (whole, ≥3 kDa, ≤ 3 kDa) was stored at −80 °C for further analysis. HCE cells were incubated with 100 μg/mL of LPS for 24 h. The cells were washed three times with PBS and then cultured for 24 h. The cells were treated with whole, ≥3 kDa, or ≤3 kDa of CM from macrophages, HBMSCs, and HBMSCs + macrophages co-cultured for 24 h. The cell culture supernatant was carefully harvested and filtered through 0.2 μm filters. After filtration, the HCE cell culture supernatant was removed and stored at −80 °C for further analysis.
Cytokine IL-1α, IL-1β, and IL-1RA quantification
Media from the HBMSC and macrophage co-culture CM (whole, ≥3 kDa, or ≤3 kDa)-treated HCE cells were collected and the levels of IL-1α and IL-1β were detected using a quantitative enzyme linked immunosorbent assay (ELISA) kit (KOMA Biotech, Korea), following the manufacturer’s instructions. The IL-1RA levels in the CM (≥3 kDa) were also assessed by ELISA (R&D Systems, Minneapolis, MN, USA). The test groups were as follows: (1) macrophages, (2) HBMSCs, and (3) HBMSCs + macrophages co-culture. The supernatant was carefully harvested from each culture and filtered through 0.2 μm filter. After filtration, CM were separated into the ≥3 kDa fraction, using a Microcon Centrifugal Filter Device. Each experimental condition was performed in triplicate.
Animal experiment
BALB/c (6–8 weeks old, n = 5) mice were anesthetized with xylazine (7 mg/kg) and ketamine (70 mg/kg). A 2 mm diameter filter was soaked in 20% ethanol and placed in the right eye of the mouse for 1 minute. The right eye of the mouse was washed with PBS and then a wound with a diameter of 2 mm was made using surgical punches and blades. The wound was visualized using 0.25% fluorescein and photographed under a microscope. IL-1RA (Sino Biological Inc, Beijing, China) was mixed with PBS to form eye drops and applied to the damaged eyes. After 16 h, the reduction of wound area at each concentration of IL-1RA was measured using the Image J software.
Size exclusion chromatography (SEC)
The prepared CM were lyophilized and dissolved in 50 mM sodium phosphate buffer (pH 6.8). After 0.45 μm filtration, the CM were subjected to SEC on a Phenomenex Biosep-SEC-S 2000 column (7.8 × 300 mm) (Phenomenex, Torrance, CA, USA). The column was run with 50 mM sodium phosphate buffer (pH 6.8) at a flow rate of 0.5 mL/min; fractions (1 mL) were combined.
Q-TOF-LC-MS/MS
Q-TOF-LC-MS/MS was performed as described previously [Citation19]. To identify secreted proteins, the MS/MS spectra were compared against protein sequences from the National Center for Biotechnology Information database, using the Mascot search program (Matrix Science, Boston, MA, USA).
To identify the proteins, all the MS/MS spectra of the tryptic peptides that were derived from spots were compared against protein sequences from the National Center for Biotechnology information (NCBI) database by using the Mascot search program (Matrix Science, Boston, MA).
Results
PMA-induced differentiation of THP-1 cells into macrophages
To confirm the differentiation of THP-1 monocyte cells into macrophages, the increase in the cytoplasmic volume of the differentiated cells was confirmed by microscopy. The cytoplasmic volume of THP-1-derived cells in the PMA-treated group increased compared with that in the control group (Figure S1A). When the THP-1 cells were treated with PMA, they were transformed from suspension cells to adherent cells. The cells also lost their ability to proliferate during the process; however, the cells in the control group showed increased proliferation activity. Therefore, the PMA-treated cells could be recognized as arrested cells (Figure S1B). In addition, expression of CD14, a macrophage differentiation marker, was measured by fluorescence-activated cell sorting (FACS), which confirmed that the number of CD14-expressing cells in the PMA-treated group increased compared with that in the control group (Figure S1C).
Anti-inflammatory effects of HBMSCs and macrophages CM
The level of the IL-1α protein decreased in culture supernatants of HCE cells grown in CM from HBMSCs + macrophages compared with that in cells grown in CM from macrophages (374.59 pg/mL) or HBMSCs (373.21 pg/mL) (Figure (A)). While the decrease in IL-1α protein level was not significant between the other CM (macrophages and HBMSCs), there was a significant decrease in cultures grown in CM from HBMSCs + macrophages (120.73 pg/mL) following LPS treatment (p < 0.01). Likewise, a significant decrease in the IL-1β protein level was observed in cultures grown in CM from HBMSCs + macrophages (60.34 pg/mL) compared with that in cells grown in CM from macrophages (242.67 pg/mL) and HBMSCs (245.55 pg/mL) alone (Figure (B)). In the experiment using the fractionated CMs (≥3 kDa or ≤ 3 kDa), the ≥3 kDa CM showed a similar result to the unfractionated CM. However, the ≤ 3 kDa CM had no significant effect on the levels of IL-1α and IL-1β proteins in the cell cultures (Figure (C) and (D)).
Figure 1. Level of IL-1α (A, C) and IL-1β (B, D) in the conditioned media (CM)-treated human corneal epithelial (HCE) cell culture supernatant. IL-1α and IL-1β in HCE cell culture supernatant grown for 24 h with lipopolysaccharide (LPS) in CM from cultures of macrophages, human bone marrow mesenchymal stem cells (HBMSCs), or HBMSCs + macrophages were measured by an enzyme linked immunosorbent assay (ELISA). The CM used were whole (A, B) or separated into greater than or less than 3 kDa (C, D). Error bars represent means ± S.E.M.; *p < 0.05; **p < 0.01.
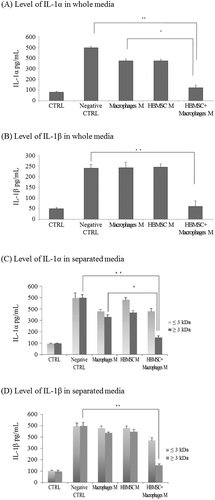
HBMSCs and macrophages co-culture produced IL-1RA
The IL-1RA protein was detected in the CM from macrophages and HBMSCs + macrophages. The level of IL-1RA in the CM from HBMSCs + macrophages (1424.53 pg/mL) was significantly higher than that in the CM from macrophages CM (785.72 pg/mL) or from HBMSCs (13.82 pg/mL) (Figure (A), p < 0.001, p < 0.01, respectively). To determine whether the increase in IL-1RA production was caused by a direct interaction between HBMSCs and macrophages, the two cell populations were cultured in indirect (using the transwell assay) or direct contact (using co-culture). When in indirect contact, the cells were placed in 6-well transwell plates. The two different cells were placed into two parts, upper and lower, separated by a permeable membrane (Figure (B)). In the direct culture conditions, the cell populations were simply mixed and cultured in a single plate. As a result, when the macrophages were in direct contact with HBMSCs, IL-1RA production reached 1614.04 pg/mL, which was significantly higher than that by the indirectly contacted cells (824.76 pg/mL) (Figure (C), p < 0.05).
Figure 2. IL-1RA level in HBMSC and macrophage co-culture CM. The level of IL-1RA secretion was evaluated by an enzyme linked immunosorbent assay (ELISA). (A) The expression of IL-1RA in culture supernatant from human bone marrow mesenchymal stem cells (HBMSCs) + macrophages co-culture in direct contact was evaluated. (B) Direct or indirect co-culture with macrophages and HBMSCs. To verify if the increased IL-1RA production was caused by an interaction between HBMSCs and macrophages, macrophages were co-cultured in vitro with HBMSCs with no direct contact using the transwell assay (indirect co-culture) or co-cultured with HBMSCs in direct contact (direct co-culture). (C) Evaluated level of IL-1RA from indirect or direct co-culture. IL-1RA production during the indirect or direct co-culture of HBMSCs and macrophages is shown at 24 h after lipopolysaccharide (LPS) stimulation. Data represent at least three experiments. Error bars represent means ± S.E.M.; *p < 0.05, **p < 0.01, ***p < 0.001.
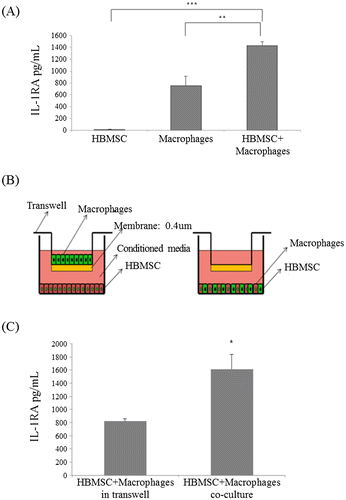
IL-1RA administration caused recovery of eye wounds in corneal wound-healing model
To confirm that IL-1RA accelerates the recovery of eye wounds in mice, eye drops containing IL-1RA at 0, 0.1, 1, 10, or 100 ng/mL were used to treat wounded mouse eyes every hour. After 16 h, the size of the wound was measured under a microscope after fluorescein staining. Compared with the wounds in the control group, those of the IL-1RA-treated groups showed a decreasing trend at 16 h after the initial treatment (Figure ). The 0.1 ng/mL-treated group (103.34 mm2, p < 0.05), 1 ng/mL-treated group (29.65 mm2, p < 0.001), and the 10 ng/mL-treated group (13.76 mm2, p < 0.001) all showed significantly reduced wound sizes compared with those of the control group (173.01 mm2). However, in the 100 ng/mL-treated group (161.89 mm2, N.S.), the wound size did not decrease compared with that in the control group.
Figure 3. Recovery of eye wounds by IL-1RA using in vivo corneal wound-healing model. The mice were treated with IL-1RA-containing eye drops at concentrations of 0, 0.1, 1, 10, or 100 ng/mL on the right eye after scarring in the eye of each mouse. The control group was treated with vehicle. (A) Eye wound areas stained with fluorescein at 16 h after the administration of IL-1RA. (B) Graph showing the area of the eye wounds (n = 5). Error bars represent means ± S.E.M.; *p < 0.05; **p < 0.01 ***p < 0.001.
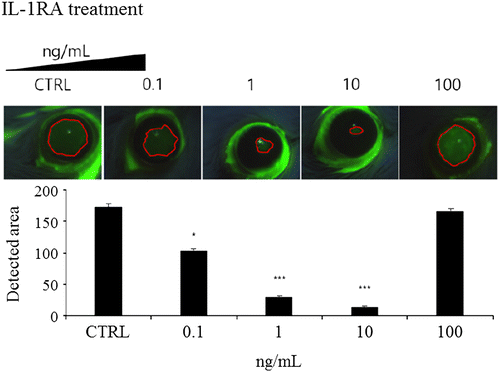
Quantitative analysis of CM by SEC
The co-cultured CM (≥3 kDa) and macrophages CM (≥3 kDa), which were shown to have anti-inflammatory effects on inflammatory HCE cells, were analyzed by SEC for marker of three different sizes markers (440 kDa, 67 kDa, and 13.7 kDa) at a wavelength of 280 nm (Figure ). The HBMSCs CM-only (≥3 kDa) group was excluded from the analysis, since it did not show significant anti-inflammatory effects. At 67 kDa, the co-cultured CM had a significantly higher peak than macrophages-only CM. In contrast, the 13.7 kDa peak had a significantly lower peak than co-cultured CM (Figure (A)). Subsequently, the two peaks that showed differences were extracted and fractionated (Figure (B)).
Figure 4. Analysis of conditioned media (CM) by size exclusion chromatography (SEC). The CMs from macrophage culture (green arrow) or human bone marrow mesenchymal stem cells (HBMSCs) + macrophages co-coculture (red arrow) were analyzed at 280 nm wavelengths, using three markers (black arrow). Overlay of a single injection chromatograms of the CM (≥3 kDa), is injection was 100 μL.
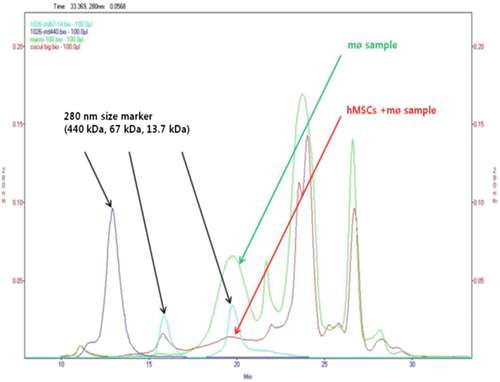
Figure 5. Quantitative differences in the macrophage and co-culture conditioned media (CM) by size exclusion chromatography (SEC). (A) A comparison of the two groups (green arrow: macrophages CM, red arrow: co-culture CM) showed a difference in peak levels at 67 kDa and 13.7 kDa. (B) The two peaks showing a difference were then partitioned (red box, left: 67 kDa, right: 13.7 kDa).
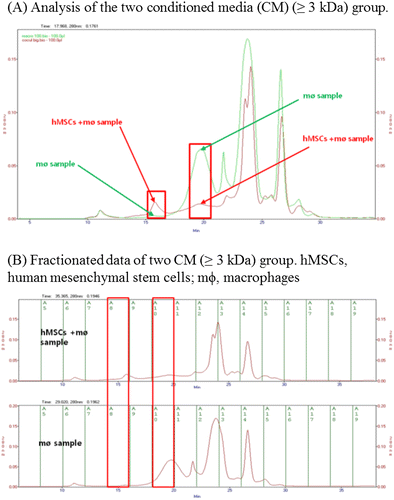
Identification of CM by QTOF-LC-MS/MS
On analyzing the separated co-cultured CM by QTOF-LC-MS/MS, we found that co-cultured CM (≥3 kDa) contained proteins such as immunoglobulin variable region VH gamma domain, alpha-catulin, and zinc finger protein ubi-d4 at the 67-kDa peak. These proteins were not found in CM from macrophages (≥3 kDa) (Table ). Analysis of the 13.7 kDa peak showed that both CM (macrophage and co-culture) contained immunologically responsive proteins. B cell antibody heavy chain variable region and PAM (protein associated with Myc) were detected in the co-cultured CM, whereas the CM from macrophages did not contain any effective proteins other than B cell antibody heavy chain variable region (Table ).
Table 1. Protein analysis of the co-culture and macrophages culture CM (67 kDa).
Table 2. Protein analysis of the co-culture and macrophages culture conditioned (CM) (13.7 kDa).
Discussion
In this study, we examined the influence of MSC-macrophage interaction on wounded corneal tissue in vitro, using LPS-damaged HCE cells grown in HBMSC + macrophages co-culture conditioned-media.
In the HCE cell culture media, the IL-1α and IL-1β levels of the group treated with the CM from HBMSCs and macrophages co-culture were significantly lower than those in the negative control, CM from macrophages, or CM from HBMSCs (Figure (A) and (B)). Thus, the co-culture of HBMSC and macrophage had a significant anti-inflammatory effect on damaged HCE cells. Moreover, when the HCE cells were treated with separated CM (≥3 kDa or ≤ 3 kDa), IL-1α and IL-1β levels in the group treated with the ≥3 kDa CM from HBMSCs and macrophages co-culture significantly decreased (Figure (C) and (D)). Thus, we speculated that the factor responsible for the anti-inflammatory effect of CM is a macromolecule (i.e. protein).
In order to investigate factor(s) responsible for the anti-inflammatory effect, we analyzed the CM using SEC, subsequently fractionated the significant peak at 67 and 13.7 kDa (Figure and ), and analyzed these two peaks by QTOF–LC-MS/MS (Table and ). QTOF-LC-MS/MS identified various proteins, such as albumin, IgG variable region VH gamma domain, alpha-catulin, and zinc finger protein ubi-d4 in the 67 kDa peak of co-cultured CM (≥3 kDa). These proteins were not found in macrophage CM (≥3 kDa) (Table ). Further analysis of the 13.7 kDa peak showed that both macrophage and co-culture CM contained immune response proteins (B cell antibody heavy chain variable region). PAM (protein associated with Myc) was also detected in co-cultured CM (Table ). Although albumin which showed the highest Mascot score has been reported to have a positive effect on inflammation in DES [Citation20–22], we speculated that the concentration of albumin in the co-cultured medium might not be sufficient to restore wound healing. Because very high concentration of albumin (5 mg/mL) in eye drops is needed to decrease eye wound [Citation23] and such amount is too high concentration to synthesize and secrete from cells, also compared to physiological concentration in tear film [Citation24].
Q-TOF-LC-MS/MS method has a limitation in identifying low-concentration cytokines and various growth factors. To identify the various molecules secreted by cells in co-culture CM, other methods, such as antibody arrays and bioinformatics, need to be performed for accurate qualitative analysis.
In order to clarify the factor that affects IL-1α and IL-1β expression, we hypothesized IL-1RA as a candidate factor. IL-1RA is structurally involved with the IL-1 family, such as IL-1α and IL-1β. However, IL-1RA acts as a competitive inhibitor of IL-1α and IL-1β [Citation25]. Moreover, it was reported that when IL-1RA is treated on PBMCs and monocytes in vitro, IL-1-induced IL-1 or TNF-α expression is inhibited [Citation26]. Anti-inflammatory effect of IL-1RA application has also been tested in various experimental models, such as corneal alkali injury [Citation27] and corneal transplant [Citation28].
On the other hand, recent studies have focused on the balance of IL-1 and IL-1RA in DES. Normal tear fluid contains IL-1α, IL-1β, and IL-1RA in a consistent ratio, in addition to precursor IL-1β. However, the tear fluid from DES patients contains increased IL-1α and mature IL-1β levels, so that the IL-1RA vs. IL-1 ratio is decreased. The IL-1RA concentration in tears increases in DES patients; however, the IL-1RA vs. IL-1α ratio is lower than normal. When the ratio increased in rheumatoid patients with DES, the clinical DES symptoms significantly improved [Citation29].
Therefore, we investigated the effects of the HBMSC-macrophage interaction with a focus on IL-1RA. IL-1RA level in the co-cultured CM was measured by ELISA, and it was significantly elevated in the co-culture group, although the macrophage CM also showed IL-1RA, the expression level of co-culture group was significantly higher than macrophage group (Figure (A)). Thus, we speculated that HBMSCs support IL-1RA expression of macrophages, because the macrophage-only CM contained IL-1RA as previously reported [Citation30].
Because there have been various studies showing that intercellular contact regulates proliferation and modulation cytokine secretion [Citation31,32], we investigated the difference in expression of IL-1RA during direct or indirect contact between HBMSC and macrophage. The cells were cultured in direct contact (simply mixed culture) or indirect contact using a Transwell assay (Figure (B) and (C)). The results showed that the expression of IL-1RA in the direct-contact group was two-fold higher than that in the indirect-contact group, which implied that direct contact plays important role in the IL-1RA release. The IL-1RA expression in the indirect-contact group (Figure (C)) was similar to that of the macrophage-alone group (Figure (A)), even though it is difficult to compare the results because the experiments were not conducted simultaneously.
In order to determine the effect of increased IL-1RA levels by the interaction between HBMSCs and macrophages on corneal tissue damage repair, we investigated the effect of IL-1RA administration in a corneal wound-healing mouse model. IL-1RA doses of 0.1–10 ng/mL showed a dose-dependent and significant wound healing. However, 100 ng/mL group was similar to the control group. Therefore the overall dose-response relationship showed skewed U-shape. This could be explained with the balance effect between IL-1 and IL-1RA. A level of IL-1β is required in normal wound repair because IL-1β plays a critical role in the migration of immune cells to corneal wound via production of proteolytic enzymes such as MMPs [Citation33,34]. In the inflammatory environment, proper amount of IL-1RA can inhibit the excessive activity of IL-1β, therefore facilitate corneal wound healing. However, if too large amount of IL-1RA is administered, IL-1β could be blocked, and then the immune cell migration essentially needed to wound healing could also be inhibited. Actually, it was reported when 20 mg/mL of IL-1RA was topically administered to mechanically injured cornea model, the inflammatory cell influx was markedly blocked [Citation35].
In summary, CM from co-cultured HBMSCs and macrophages had a synergistic immunomodulatory effect on corneal epithelial cells in the inflammatory environment. The CM contained significantly increased IL-1RA, and the CM treatment reduced IL-1α and IL-1β levels in damaged HCE cell cultures. The direct interaction between HBMSCs and macrophages led to increased expression of IL-1RA. Moreover, IL-1RA treatment of wounded mouse eyes significantly reduced the size of the wounds after 16 h of treatment.
Unfortunately, the factor responsible for the increased IL-1RA release from HBMSCs and macrophages co-culture remains unknown. The HBMSCs and macrophages interaction could be a candidate mechanism underlying the immunomodulatory treatment of DES. Further research might reveal that this interaction could be applied to other inflammatory diseases.
Author contributions
WYJ and JHK designed the study and wrote the manuscript. CWK contributed to the development of the manuscript. WYJ and JHK carried out the experiments and analyzed the data. All authors have read and approved the final manuscript.
Disclosure statement
No potential conflict of interest was reported by the authors.
Funding
This work was supported by Korea University, with additional support from Korea University BK21 Plus Program (School of Life Sciences and Biotechnology, Korea University).
Supplemental data
Supplemental data for this article can be accessed https://doi.org/10.1080/09168451.2018.1438167
Sppl_Figures.pdf
Download PDF (171.1 KB)Acknowledgments
I appreciate all my research collaborators and the members of the Laboratory of Bio Pharmaceutical Processes department of Life Sciences and Biotechnology at Korea University for their help and technical support.
References
- Goto E, Yagi Y, Matsumoto Y, et al. Impaired functional visual acuity of dry eye patients. Am J Ophthalmol. 2002;133(2):181–186.
- Tavares Fde P, Fernandes RS, Bernardes TF, et al. Dry eye disease. Semin Ophthalmol. 2010;25(3):84–93.
- Avni I, Garzozi HJ, Barequet IS, et al. Treatment of dry eye syndrome with orally administered CF101: data from a phase 2 clinical trial. Ophthalmology. 2010;117(7):1287–1293.
- Luo L, Li DQ, Doshi A, et al. Experimental dry eye stimulates production of inflammatory cytokines and MMP-9 and activates MAPK signaling pathways on the ocular surface. Invest Ophthalmol Vis Sci. 2004;45(12):4293–4301.
- Niederkorn JY, Stern ME, Pflugfelder SC, et al. Desiccating stress induces T cell-mediated Sjogren’s Syndrome-like lacrimal keratoconjunctivitis. J Immunol. 2006;176(7):3950–3957.
- Management and therapy of dry eye disease: report of the management and therapy subcommittee of the international dry eye workshop. Ocul Surf. 2007;5(2):163–178.
- Paiva CS, Pflugfelder SC. Rationale for anti-inflammatory therapy in dry eye syndrome. Arq Bras Oftalmol. 2008;71(6 Suppl):89–95.
- Gordon S. The macrophage: past, present and future. Eur J Immunol. 2007;37(Suppl 1):S9–17.
- Martinez FO, Helming L, Gordon S. Alternative activation of macrophages: an immunologic functional perspective. Annu Rev Immunol. 2009;27:451–483.
- Lucas T, Waisman A, Ranjan R, et al. Differential roles of macrophages in diverse phases of skin repair. J Immunol. 2010;184(7):3964–3977.
- Nahrendorf M, Swirski FK, Aikawa E, et al. The healing myocardium sequentially mobilizes two monocyte subsets with divergent and complementary functions. J Exp Med. 2007;204(12):3037–3047.
- Pull SL, Doherty JM, Mills JC, et al. Activated macrophages are an adaptive element of the colonic epithelial progenitor niche necessary for regenerative responses to injury. Proc Nat Acad Sci USA. 2005;102(1):99–104.
- Oh JY, Kim MK, Shin MS, et al. Cytokine secretion by human mesenchymal stem cells cocultured with damaged corneal epithelial cells. Cytokine. 2009;46(1):100–103.
- Kim J, Hematti P. Mesenchymal stem cell-educated macrophages: a novel type of alternatively activated macrophages. Exp Hematol. 2009;37(12):1445–1453.
- Oh JY, Kim MK, Shin MS, et al. The anti-inflammatory and anti-angiogenic role of mesenchymal stem cells in corneal wound healing following chemical injury. Stem cells. 2008;26(4):1047–1055.
- Phinney DG, Prockop DJ. Concise review: mesenchymal stem/multipotent stromal cells: the state of transdifferentiation and modes of tissue repair–current views. Stem cells. 2007;25(11):2896–2902.
- Nauta AJ, Fibbe WE. Immunomodulatory properties of mesenchymal stromal cells. Blood. 2007;110(10):3499–3506.
- Nemeth K, Leelahavanichkul A, Yuen PS, et al. Bone marrow stromal cells attenuate sepsis via prostaglandin E(2)-dependent reprogramming of host macrophages to increase their interleukin-10 production. Nat Med. 2009;15(1):42–49.
- Jung IS, Kim HJ, Noh R, et al. Effects of extremely low frequency magnetic fields on NGF induced neuronal differentiation of PC12 cells. Bioelectromagnetics. 2014;35(7):459–469.
- Mushtaq S, Naqvi ZA, Siddiqui AA, et al. Albumin precursor and Hsp70 modulate corneal wound healing in an organ culture model. Acta Histochem. 2011;113(1):36–42.
- Gong H, Johnson M, Ye W, et al. The non-uniform distribution of albumin in human and bovine cornea. Exp Eye Res. 1997;65(6):747–756.
- Tsubota K, Higuchi A. Serum application for the treatment of ocular surface disorders. Int Ophthalmol Clin. 2000;40(4):113–122.
- Higuchi A, Ueno R, Shimmura S, et al. Albumin rescues ocular epithelial cells from cell death in dry eye. Curr Eye Res. 2007;32(2):83–88.
- Runstrom G. Tighe B tear albumin concentration: effects of sampling technique, tear flow stimulation and subject individuality. Contact Lens and Anterior Eye. 2013;36:e44.
- Arend WP, Smith MF Jr, Janson RW, et al. IL-1 receptor antagonist and IL-1 beta production in human monocytes are regulated differently. J Immunol. 1991;147(5):1530–1536.
- Granowitz EV, Clark BD, Vannier E, et al. Effect of interleukin-1 (IL-1) blockade on cytokine synthesis: I. IL-1 receptor antagonist inhibits IL-1-induced cytokine synthesis and blocks the binding of IL-1 to its type II receptor on human monocytes. Blood. 1992;79(9):2356–2363.
- Yamada J, Dana MR, Sotozono C, et al. Local suppression of IL-1 by receptor antagonist in the rat model of corneal alkali injury. Exp Eye Res. 2003;76(2):161–167.
- Dana MR, Yamada J, Streilein JW. Topical interleukin 1 receptor antagonist promotes corneal transplant survival. Transplantation. 1997;63(10):1501–1507.
- Solomon A, Dursun D, Liu Z, et al. Pro- and anti-inflammatory forms of interleukin-1 in the tear fluid and conjunctiva of patients with dry-eye disease. Invest Ophthalmol Vis Sci. 2001;42(10):2283–2292.
- Janson RW, Hance KR, Arend WP. Production of IL-1 receptor antagonist by human in vitro-derived macrophages. Effects of lipopolysaccharide and granulocyte-macrophage colony-stimulating factor. J Immunol. 1991;147(12):4218–4223.
- St Croix B, Sheehan C, Rak JW, et al. E-Cadherin-dependent growth suppression is mediated by the cyclin-dependent kinase inhibitor p27(KIP1). The Journal of cell biology. 1998;142(2):557–571.
- Barille S, Collette M, Bataille R, et al. Myeloma cells upregulate interleukin-6 secretion in osteoblastic cells through cell-to-cell contact but downregulate osteocalcin. Blood. 1995;86(8):3151–3159.
- Bondeson J, Wainwright SD, Lauder S, et al. The role of synovial macrophages and macrophage-produced cytokines in driving aggrecanases, matrix metalloproteinases, and other destructive and inflammatory responses in osteoarthritis. Arthritis research & therapy. 2006;8(6):R187.
- Lee JG, Heur M. Interleukin-1beta enhances cell migration through AP-1 and NF-kappaB pathway-dependent FGF2 expression in human corneal endothelial cells. Biol Cell. 2013;105(4):175–189.
- Stapleton WM, Chaurasia SS, Medeiros FW, et al. Topical interleukin-1 receptor antagonist inhibits inflammatory cell infiltration into the cornea. Exp Eye Res. 2008;86(5):753–757.