Abstract
To investigate the regulation of metallothionein genes (HsMTs) of Hyriopsis schlegelii, 1,121-bp and 1,270-bp regions of the HsMT1 and HsMT2 promoters were cloned and analyzed, respectively. The two promoters shared partially conserved features and possessed distinct characteristics such as the number or position of metal response elements (MREs). Further analysis of the HsMT1 and HsMT2 promoters was performed by the reporter assay using the luciferase gene. Both promoters were activated by various metals, and presented different levels of metal ions inducibility in human hepatoblastoma cells. Deletion mutant assays demonstrated that both the longest promoter regions achieved the maximum inducibility, and the metal inducibility was dependent on the presence of the MRE in HsMT1 and the distal MRE in HsMT2. In addition, we cloned a putative metal responsive transcription factor (hereby designated as HsMTF-like) and studied its effect on HsMTs expression in human hepatoblastoma cells. An in vivo assay demonstrated that HsMTF-like activates basal HsMTs transcription level, and the MRE in the HsMTs promoter mediates this activation process. Moreover, this basal transcription level can be further boosted by zinc treatment. In conclusion, the regulation mechanism for MT activation in H. schlegelii should be evolutionarily conserved.
Metal response elements (MREs) play important roles in metallothionein (MT) transcription of Hyriopsis schlegelii.
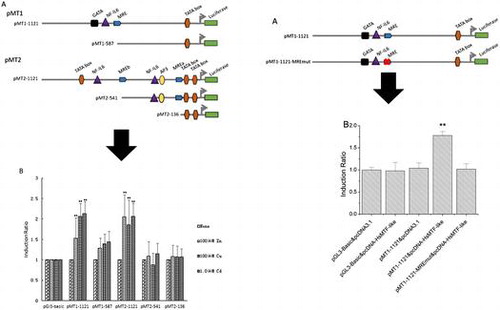
Mammalian metallothioneins (MTs) are a family of cysteine-rich, low-molecular weight (6–7 kDa), inducible proteins in which numerous (about 30%) cysteine residues confer substantial metal-binding capabilities through the formation of metal-thiolate bonds [Citation1,2]. MTs regulate the concentration of essential metal ions (e.g. copper ion, Cu2+ and zinc ion, Zn2+) and detoxify non-essential metal ions (e.g. cadmium ion, Cd2+) or excessive amounts of essential metal ions in cells [Citation1,3]. Based on these features, MTs have been utilized as biomarkers for the evaluation of the effects of pollutants in different aquatic organisms [Citation4].
The transcription of MTs could be induced by various agents and conditions, including metal ions, cytokines, stress, free radicals, and hormones, and is mediated by cis-acting elements that occur within its promoter region [Citation5]. Metal ions are the most common and potent inducers of MTs [Citation6]. Further analysis of metallothionein gene promoters has identified metal response elements (MREs). Multiple copies of MREs exist in the upstream regions of MT genes and share the highly conserved core sequence 5′-TGCRCNC-3′ (R represents a purine, and N is any nucleotide). It is essential for MRE-mediated transcriptional induction by metal ions to activate a metal responsive transcription factor [Citation7]. Metal responsive transcription factor 1 (MTF-1) binds to these MREs and confers metal ions inducibility [Citation8]. Furthermore, it regulates the expression of genes, including MT, glutamylcysteine synthetase (GCS), copper transporters, and Zn2+ transporters, and all these genes have multiple MREs in their promoters [Citation9,10]. MTF-1 is a highly conserved zinc fingers protein that possesses six C2H2-type zinc finger domains at its N-terminal that function as DNA-binding domains that recognize the core sequence 5′-TGCRCNC-3′ in MREs. MTF-1 is a key factor in the cellular response to metal and oxidative stress [Citation11]. MTF-1 has been identified in various species, including mouse [Citation12], human (hMTF-1) [Citation13], Japanese pufferfish [Citation14], zebrafish [Citation15,16], tilapia [Citation17], common carp [Citation18], Drosophila [Citation8], and Pacific oyster [Citation19]. Based on these studies, it could be inferred that the activation of MT genes is caused by the translocation of MTF-1 from the cytoplasm and crosstalk with co-activators after metal ion treatment [Citation20]. However, limited information about the mechanism for MTs activation in freshwater mussels was known. Recently, some researchers pointed out that the conserved mechanism for MTs activation in vertebrates was not the same for most invertebrate species [Citation21]. In earthworms, alternative MT activation mechanism is mediated by a cAMP responsive element binding protein (CREB) that is a transcriptional activator of its MT [Citation22]. Thus, it is necessary to characterize the underlying molecular mechanisms and regulatory pathways of MTs.
Hyriopsis schlegelii, which sourced from the Biwa Lake of Japan, is a commercially important aquaculture species of freshwater mussel used for pearl cultivation in China. Molluscan MT expressions exposed in the aqueous field have been assessed to be ideal indicators for evaluating contamination of water [Citation23]. In a previous study, we reported the presence of two distinct MT cDNA isoforms, namely, HsMT1 and HsMT2 in Hyriopsis schlegelii [Citation24]. HsMT1 transcripts are differentially expressed in various tissues, and the highest expression was detected in the hepatopancreas, whereas HsMT2 transcripts mainly accumulated in the gonads in normal conditions. In addition, we also determined that HsMT1 and HsMT2 are involved in responses to Cd2+ exposure [Citation24]. Therefore, it is very significant to clarify the induction mechanism of MT in mussel under heavy metals load. We thus inferred that metal response elements (MREs) within their promoter regions should be different and it was worthy to be recognized. Therefore, the aims of the present study were to clone and characterize promoters of HsMTs, and elucidate its roles in response to heavy metal ions.
Materials and methods
Cloning of HsMT1 and HsMT2 promoter regions from genomic DNA
Using the cDNA sequence of HsMT1 and HsMT2 (GenBank Accession Nos. KJ019820 and KJ019821) that was reported in our previous study [Citation24], we designed primers to PCR amplify the promoter regions of HsMTs from genomic DNA.
The promoter region of HsMT1 was obtained using a genomic walking kit (Code: D316, Takara, Dalian, China). The adaptor primer AP2 was included in the kit, and the HsMT1 gene-specific primers (HsMT1-GSP1, HsMT1-GSP2, and HsMT1-GSP3) are listed in the Table . PCRs were performed thrice, and the PCR products of the former two reactions were used as templates of the subsequent cycles. AP2 was always used as the forward primer; HsMT1-GSP1, HsMT1-GSP2, and HsMT1-GSP3 were the reverse primers, respectively. After three rounds of PCR amplification, the 1,100-bp bands were purified by agarose gel electrophoresis and subcloned into pMD19-T vectors (Takara) for nucleotide sequencing analysis.
Table 1. Primers used in this study.
The promoter region of the HsMT2 gene was obtained using the Universal Genome Walker 2.0 kit (Cat. No. 636405, Takara), following the manufacturer’s instructions. Briefly, after digestion using four restriction enzymes (Dra I, EcoR V, Pvu II, and Stu I) overnight at 37 °C, the partially digested and purified genomic DNAs were ligated to GenomeWalker adaptors. The forward primers were AP1 and AP2, which were provided by the kit. The reverse primers were HsMT2-GSP1 and HsMT2-GSP2 (listed in the Table ), which were complementary to the cloned 5′-untranslated region (5′-UTR) sequence of HsMT2. The first round of PCR was performed using the AP1 and HsMT2-GSP1 primers, and the second round of PCR with the AP2 and HsMT2-GSP2 primers. After two rounds of nested PCR amplification, a 1,270-bp band was resolved and purified by agarose gel electrophoresis and then subcloned into pMD19-T vectors (Takara). Nucleotide sequences were confirmed by sequencing, which was performed by Sangon Biotech (Shanghai, China).
Cloning of HsMTF-like whole ORF cDNA
Based on the gonad transcriptome data that was constructed by our laboratory [Citation25], a MTF1 homologue (hereby designated as HsMTF-like) that was homologous to human MTF-1 was found. The HsMTF-like gene nucleotide sequence was composed of three parts including a 77-bp 5′-untranslated regions (5′-UTR), a 1,551-bp open reading frame (ORF) and 405-bp 3′-UTR. Subsequently, the complete ORF was amplified by PCR through designing a pair of primers (HsMTF-like-F/R) that contained Nhe I and Hind III restriction sites (in Table ), respectively. Thirty-five cycles of PCR amplification (temperature profiles, 98 °C for 10 s, 55 °C for 5 s, and 72 °C for 2 min) were performed in a thermalcycler.
Bioinformatics analysis
Sequence alignment of the HsMT1 and HsMT2 promoters was performed using ClustalW (http://www.ebi.ac.uk/clustalw/). Transcription factor binding sites (TFBs) in the promoter regions were predicted using the MATCH program in the TRANSFAC database 7.0 (http://www-bimas.cit.nih.gov/molbio/signal/). The ORF of HsMTF-like cDNA was predicted and deduced to the amino acid sequence with ORF Finder program (https://www.ncbi.nlm.nih.gov/orffinder). HsMTF-like cDNA sequence was subjected to BLASTX search against NCBI GenBank (https://blast.ncbi.nlm.nih.gov/Blast.cgi) for examining the sequence homology with its orthologs. Multiple sequence alignment of HsMTF-like was also performed with ClustalW program (https://www.genome.jp/tools/clustalw/).
Constructs of deletion mutants
PCR and digestion-ligation methods were used to construct the desired five deletion mutants (two for HsMT1 and three for HsMT2). The longest fusion constructs, pMT1-1121 and pMT2-1121, were generated by amplifying genomic DNA by primers with the corresponding restrictions sites (Table ). The pGL3-Basic vectors use for mutant construction were prepared from overnight bacterial cultures using an E.Z.N.A. plasmid mini kit (Omega) according to the manufacturer’s protocol. The purified PCR products and pGL3-Basic vector (Promega) were ligated with T4 DNA ligase (Takara) after double-digestion with Kpn I / Hind Ш (for HsMT1) or Kpn I / Nhe I (for HsMT2). Finally, both HsMT1 and HsMT2 promoters were introduced into vector pGL3-Basic, directly upstream of the firefly luciferase reporter gene.
The deletion mutants pMT1-587, pMT2-541, and pMT2-136 were obtained by inserting the PCR products amplified from pMT1-1121 and pMT2-1121 using the corresponding primers (Table ) into the pGL3-Basic vectors as previously described. Kpn I, Nhe I, or Hind Ш sites were introduced into the five sense or two antisense PCR primers, respectively (Table ). The constructs were verified by sequencing with the GLprimer2 (Table ) at Sangon Biotech (Shanghai, China).
Preparation of recombinant plasmids and mutagenesis
To further assess the possible binding activation of the MRE motifs in HsMT1 and HsMT2 promoters with HsMTF-like, series of recombinant plasmids were constructed and employed as follows. Six kinds of recombinant plasmids, which included wild-type HsMT1 and HsMT2 promoter-luciferase reporter vectors (referred to pMT1-1121 and pMT2-1121), HsMTs promoter-luciferase reporter vectors with mutated MREs (named as pMT1-1121-MREmut, pMT2-1121-MREamut, pMT2-1121-MREbmut and pMT2-1121-MREabmut), and an eukaryotic expression vector pcDNA-HsMTF-like were prepared.
The whole HsMTF-like ORF cDNA sequence was digested with the restriction enzymes Nhe I and Hind Ш, and inserted into the eukaryotic expression vector pcDNA3.1 at the same sites. Positive clones containing the pcDNA3.1-HsMTF-like were subjected to sequence confirmation.
In order to construct the plasmid of pMT1-1121-MREmut, pMT2-1121-MREamut, pMT2-1121-MREbmut and pMT2-1121-MREabmut, MRE motifs were mutated by fusion PCR with a pair of complementary primers containing the desired nucleotides. The mutations were generated using a QuickChange Site-Directed Mutagenesis kit (Qiagen) and the primers used for mutagenesis were listed in Table . Finally, the obtained PCR fragments were inserted into pGL3-Basic vector and verified by DNA sequencing at Sangon Biotech (Shanghai, China).
Cell culture and transfections
Because no immortalized molluscan cell line has been established to date, HepG2 (ATCC HB 8065, human hepatoblastoma) cells were used to study the functional characterization of the promoter regions of HsMTs. HepG2 cell line is a well-studied hepatoblastoma cell line used for MT gene regulation studies for many years [Citation26], and many MT gene promoters such as trout [Citation6], loach and pike [Citation27], icefish [Citation28], Zebrafish [Citation26] and crucian carp [Citation29] were tested in HepG2 cell line. Considering the conservation of structure and regulation of MT genes [Citation24], HepG2 is chosen as a model to test various heavy metals for their inductions of the HsMTs genes. HepG2 cells were cultured and maintained in Dulbecco’s modified Eagle’s medium (DMEM, GIBCO, NY, USA) containing 100 μg/mL of penicillin-streptomycin and 10% fetal bovine serum (FBS) and maintained at 37 °C under a humidified atmosphere with 5% CO2. The medium was changed two or three times each week. HepG2 cells were seeded into 24-well plates containing approximately 1.2 × 104 cells/well 20 h prior to transfection. Then, 100 ng of the recombinant pGL3-promoter plasmids and 5 ng of the internal reference pRL-TK vectors were co-transfected into the cells using Fugene reagent (Promega, USA). Three hours after transfection, the cells were incubated with serum-free medium containing various concentrations of Zn2+ (50, 100, and 150 μM), Cd2+ (0.5, 1.0, and 1.5 μM), and Cu2+ (50, 100, and 150 μM) for 12 h for the following activity assays. After the application of the heavy metals, the cells were harvested and prepared for analysis of HsMT promoters activity.
In addition, for the HsMTF-like induction experiment: 1 μg of pGL3-Basic, pMT1-1121, pMT2-1121, pMT1-1121-MREmut, pMT2-1121-MREamut, pMT2-1121-MREbmut or pMT2-1121-MREabmut were co-transfected with 1 μg of pcDNA3.1-Basic or pcDNA-HsMTF-like. An internal control vector pRL-TK (25 ng/well) was also prepared and co-transfected using Fugene reagent according to the manufacturer’s instructions. For heavy metal induction, HepG2 cells were treated with 100 μM Zn2+ when cotransfection of expression vector (pcDNA3.1 or pcDNA-HsMTF-like) and reporter vectors (pMT1-1121, pMT2-1121, pMT1-1121-MREmut, pMT2-1121-MREamut, pMT2-1121-MREbmut or pMT2-1121-MREabmut). After 24 h, the cells were harvested, then, firefly and Renilla luciferase activity were measured for each sample using a dual-luciferase reporter assay system (Promega). The firefly luciferase data were corrected for transfection efficiency using Renilla luciferase activity.
Luciferase reporter assay
Cell lysates were harvested and assayed for firefly and Renilla luciferase activities using a dual-luciferase reporter assay system (Promega), according to the manufacturer’s instructions. Luciferase activity was measured using a 96-well microplate luminometer (Promega). The concrete experimental and data treatment methods were the same as previously described [Citation29,30]. Briefly, the experimental data of the relative luciferase activity and the induction ratios were analyzed with two-tailed t-test. The level of significance was set to p < 0.05.
Results
Structural characterization of HsMT promoters
To investigate the regulatory mechanisms of HsMT1 and HsMT2, their promoter sequences were cloned and analyzed. By using two different PCR strategies as described in the Materials and methods, we obtained two fragments of 1,121 bp and 1,270 bp in size of the promoter regions of HsMT1 and HsMT2, respectively. Their nucleotide sequences have been submitted to the GenBank database under Accession Numbers KX279831 and KX279832, respectively.
Bioinformatics analysis of the two promoter regions was performed, and various transcription factor binding sites (TFBs) were predicted. Analysis of the two regions revealed that both promoters had high A + T content (61.5% for HsMT1 and 60.5% for HsMT2, respectively) and contained a canonical TATA sequence (TATAAA) (Figure ). One copy of metal response element (MRE, TGCGCAC) in the HsMT1 promoter was identified at −588 to −594 bp upstream of the start codon. On the other hand, two MREs (MREa and MREb) in HsMT2 promoter were identified. The proximal (MREa) and distal MRE (MREb) were located from −135 to −141 bp (TGCACAC) and from -540 to -546 bp (TGCGCAC) upstream of the start codon, respectively. In addition to the MREs, several other TFBs, which were also found in the human or mouse MT promoters, were predicted by software (Figure ). HsMT1 promoter regions showed several TFBs including TATA box, interleukin-6 (NF-IL6, TKNNGNAAK), GATA factor (GATA, WGATAR), hepatocyte nuclear factor 3 (HNF-3, TRTTTGY), CCAAT/enhancer binding protein (C/EBP, ATTGC) and erythoid cell-specific nuclear factor (NF-E1, WGATAR) (Figure ). Apart from the TFBs in HsMT1 promoter regions, HsMT2 had three more TFBs such as the activator protein-3 (AP-3, TGTGGATT), c-Myb (TAACTG) and Oct-1 (ATGCAAAT) (Figure ). These TFBs contained stress/immune-related binding sites, such as C/EBP and NF-IL6. In addition, it had been reported that the binding sites of HNF-3 and GATA were related with cellular proliferation/apoptosis, differentiation, embryonic development and organ morphogenesis [Citation31]. Alignment of the HsMT1 and HsMT2 promoter regions showed 36.5% identity.
Analysis of the activities of the HsMT1 and HsMT2 promoters
To evaluate the activities of both the HsMT1 and HsMT2 promoters to Zn2+, Cu2+, and Cd2+, a luciferase reporter assay was conducted by transfecting each reporter plasmid (pMT1-1121 and pMT2-1121) and the reference pRL-TK vector into HepG2 cells. It was worth pointing out that this experiment just intended to check whether two promoters could respond to metal ions in HepG2 cells. After treatment with Zn2+ (50, 100, and 150 μM), Cu2+ (50, 100, and 150 μM), and Cd2+ (0.5, 1.0, and 1.5 μM), cell lysates were prepared and assayed for luciferase activity (Figure ). The uninduced basal luciferase activity of pMT1-1121 or pMT2-1121 divided by the activity of the empty vector pGL3-Basic was set as 1.0. The induction ratio was calculated using adjustments accordingly with the uninduced basal activity.
Figure (A) shows that the promoter activity of pMT1-1121 increased by 2.4-fold (p < 0.01), 2.0-fold (p < 0.01), and 1.8-fold (p < 0.01) induction with stimulation using 150 μM Cu2+, 1.0 μM Cd2+, and 150 μM Zn2+, respectively. However, promoter activity only increased by 1.2-fold in the presence of 1.5 μM Cd2+. Nevertheless, 150 μM Zn2+ was identified as a potent inducer for HsMT2 expression, which induced a 3.2-fold increase in expression relative to that of the control (Figure (B)), followed by 150 μM Cu2+ (3.0-fold increase) and 1.0 μM Cd2+ (2.5-fold increase). Furthermore, the application of a high concentration of Cd2+ (1.5 μM) exerted an inhibitory effect on the expression of HsMT2, which showed only a 1.2-fold increase compared to that in the control.
Figure 2. Transcriptional activity of the HsMT1 5′-promoter regions (A) and HsMT2 5′- promoter regions (B) after induction using Zn2+ (50, 100, and 150 μM), Cu2+ (50, 100, and 150 μM), and Cd2+ (0.5, 1.0, and 1.5 μM). The background activity of the pGL3-Basic vector were set as 1.0 and all the other activities were adjusted accordingly. The number on the bars indicates the induction ratio compared with uninduced basal activity. Significant differences across control are indicated with ** at p < 0.05.
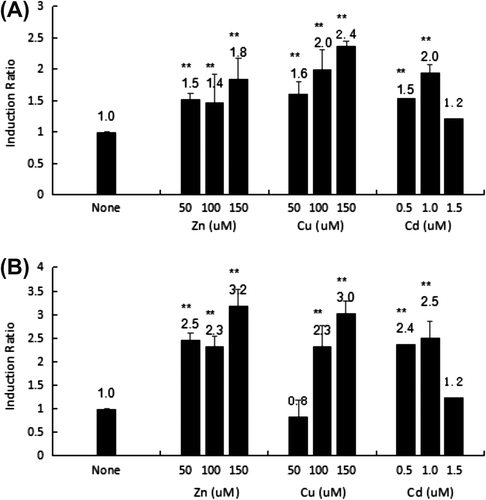
Deletion analysis of HsMT1 and HsMT2 promoters
To further study the role of MREs that were identified within the HsMT promoter regions, sets of promoters of different lengths were obtained and inserted into the luciferase reporter plasmids. A total of five deletion constructs were obtained (Fig. (A)): (1) pMT1-1121 with the obtained promoter region of HsMT1; (2) pMT1-587 referring to pMT1-1121 from which distal segments had been deleted; (3) pMT2-1121 containing the obtained promoter regions of HsMT2; (4) pMT2-541 without the distal MRE element from pMT2-1121; (5) pMT2-136 showing a deletion of the proximal MRE from pMT2-541. Each construct and the reference pRL-TK vector were transfected into HepG2 cells, treated with 100 μM Zn2+, 100 μM Cu2+, and 1.0 μM Cd2+ for 12 h, and then assayed for luciferase activity. Figure (B) shows that the three metals significantly increased the activity of the longest promoters, namely, pMT1-1121 and pMT2-1121. When the MRE was deleted from pMT1-1121, the activity of pMT1–587 decreased by 15.9, 32.3, and 32.6% in the presence of Zn2+, Cu2+, and Cd2+, respectively. For the HsMT2 promoter, pMT2–541, which underwent a deletion of the distal MRE from pMT2-1121, showed a decrease in induction from 2.0 to 1.0 (46.7%), from 1.9 to 0.9 (52.9%), and from 2.1 to 1.1 (44.7%) after treatment with Zn2+, Cu2+, and Cd2+, respectively. The two MRE deletion construct of pMT2-136 also resulted in a 47.4, 42.7, and 48.4% decrease in activity in the presence of Zn2+, Cu2+, and Cd2+, respectively. Figure (B) shows that irrespective of the deletion of one or two MREs from pMT2-1121, minimal promoter activity was observed.
Figure 3. Transcription activities of each deletion mutants of the HsMT1 and HsMT2 promoters in the presence of 100 μM Zn2+, 100 μM Cu2+, and 1.0 μM Cd2+, respectively. (A) Schematic diagram of the deletion mutants, the main cis-acting elements are marked. (B) Induction ratio of the five deletion mutants in the presence of 100 μM Zn2+, 100 μM Cu2+, and 1.0 μM Cd2+ , calculated by the ratio of the induced promoter activity to the uninduced basal promoter activity. The background activity of the pGL3-Basic vector were set as 1.0 and all the other activities were adjusted accordingly. Significant differences across control are indicated with ** at p < 0.05.
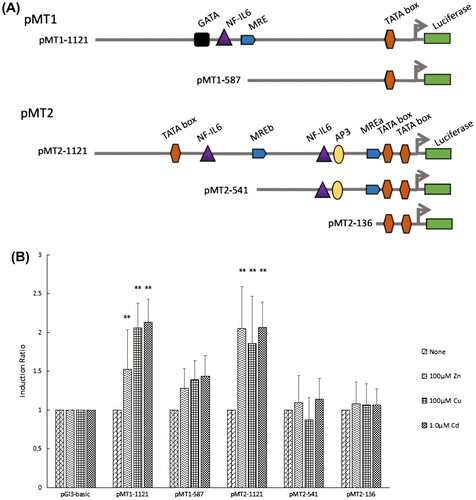
Cloning and characterization of the HsMTF-like cDNA
MTF is the known transcription factor that could activate the transcription of MT genes in vertebrates under heavy metals conditions. Therefore, it promotes us to search and clone its homologs in the mussel for further understanding the possible regulation mechanisms of MT genes. Consequently, the HsMTF-like cDNA showed a length of 2,033 bp and contained an open reading frame of 1,551 bp that encodes a putative polypeptide of 516 amino acids, with an estimated molecular weight of 57.32 kDa. Multiple sequence alignment indicated that the amino acid sequence of HsMTF-like was shorter than that of other species reported to date (Figure ). Further analysis revealed that the HsMTF-like possessed six putative zinc fingers regions that shared moderate similarity to that of human (39.44%), mouse (39.44%), pufferfish (38.89%), and zebrafish (38.89%). No transcription activation domains, including acidic-rich, proline-rich or serine/threonine-rich domains, were observed. The nucleotide sequence of HsMTF was submitted to GenBank as Accession Number KY211621.
Figure 4. Alignment of the deduced amino acid sequences of HsMTF-like and MTF-1s of other species in NCBI. Their GenBank accession numbers are as follows: hMTF: Homo sapiens X78710; zMTF: Danio rerio AF458116; fMTF: Fugu rubripes AJ131393; mMTF: Mus musculus X71327. F1-F6 represent six zinc fingers regions of HsMTF-like. Zinc fingers 1 to 6 are indicated by numbered brackets above the corresponding sequences.
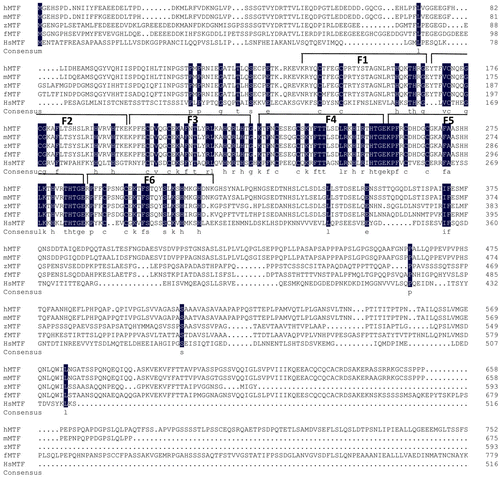
HsMTF-like modulates the promoter activity of HsMT1 and HsMT2
To explore whether the expression of HsMTF-like could modulate the promoter activities of HsMT1, the expression vectors (pcDNA-HsMTF-like) and pGL3-Basic reporter vectors (pMT1-1121, pMT1-1121, pMT1-1121-MREmut, pMT2-1121-MREamut, pMT2-1121-MREbmut and pMT2-1121-MREabmut) were constructed, respectively (Figure (A)). Thus, the expression vectors were co-transfected with pGL3-Basic reporter vectors into HepG2 cells. At 24 h post transfection, the cell lysates were collected and assayed for luciferase activity. Figure (B) showed that co-transfection of pcDNA-HsMTF-like and pMT1-1121 resulted in a significant increase in luciferase activity compared to that in the control group. However, co-transfection of pcDNA-HsMTF-like with pMT1-1121-MREmut did not result in an increase in luciferase activity. Furthermore, co-transfection of expression vectors and wild-type reporter vectors could yield strong basal transcription that could be further boosted by zinc treatment (Figure (C)–(E)). As shown in Figure (D), the addition of HsMTF-like transcription factor significantly up-regulated 1.9-fold HsMT1 promoter activity. Moreover, this activity could be further induced to 6.3-fold when incubation with Zn2+. However, these phenomenon was unable to be observed when the MRE in HsMT1 promoter was mutated. Likewise, in Figure (E), the HsMT2 promoter activity increased 2.8-fold under the addition of HsMTF-like, and sharply enhanced to 9.1-fold when Zn2+ was further added. However, as shown in Figure (E), the effect of HsMTF-like on the HsMT2 promoter is little when MREb was mutated alone (pMT2-1121-MREbmut) or when both MREa and MREb were mutated (pMT2-1121-MREabmut). Interestingly, the MREa-mutated HsMT2 promoter (pMT2-1121-MREamut) was able to present most activity (66.7%) of the wild-type (pMT2-1121).
Figure 5. Effects of HsMTF-like on the promoter activity of HsMT1 in HepG2 cells. (A). Schematic diagram of site-directed mutagenesis of the MRE motif in the HsMT1 and HsMT2 promoters. (B). The cells were co-transfected with promoter reporter plasmids containing a MRE (pMT1-1121) or a site-mutated MRE motif (pMT1-1121-MREmut) together with the HsMTF-like expression vector (pcDNA-HsMTF-like). The cells co-transfected with pGL3-Basic and pcDNA3.1 were used as control and its relative luciferase activities was arbitrarily set to 1.0 and all the other activities were adjusted accordingly. (C & D). HepG2 cells were treated with 100 μM Zn2+ in growth medium when cotransfection of expression vectors (pcDNA3.1 or pcDNA-HsMTF-like) and reporter genes (pMT1-1121, pMT1-1121-MREmut or pMT2-1121). The relative luciferase activity of the samples cotransfected with the pMT1-1121 and pcDNA-HsMTF-like (C) or pcDNA3.1 (D) was arbitrarily set to 1.0 and all the other activities were adjusted accordingly. Each bar represented the mean ± S.D of triplicates. Significant differences across control are indicated with ** at p < 0.05. (E). HepG2 cells were treated with 100 μM Zn2+ in growth medium when cotransfection of expression vectors (pcDNA3.1 or pcDNA-HsMTF-like) and reporter genes (pMT2-1121, pMT2-1121-MREabmut, pMT2-1121-MREamut or pMT2-1121-MREbmut). The relative luciferase activity of the samples cotransfected with the pMT2-1121 and pcDNA3.1 was arbitrarily set to 1.0 and all the other activities were adjusted accordingly. Each bar represented the mean ± S.D of triplicates. Significant differences across control are indicated with ** at p < 0.05.
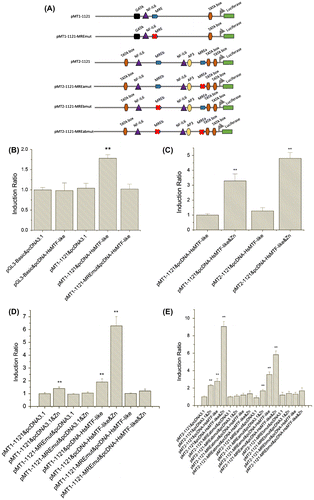
Discussion
Structural characterization of HsMT promoters
MTs are multi-functional proteins which have been studied intensively in vertebrate organisms [Citation32]. Vertebrate MT promoter regions may contain two or more MREs that share a core consensus sequence ‘TGCRCNC’ and mediate metal responses [Citation9]. To understand the potential regulatory elements involved in the transcription of HsMTs, two promoter regions obtained by genome walking or genome walker kit were validated by alignment to the corresponding overlapping fragments in the HsMT1 and HsMT2 cDNA sequences [Citation24]. The promoters of HsMT1 and HsMT2 show high A + T content (61.5 and 60.5%, respectively) and are similar to that of the rainbow trout [Citation28] and crucian carp [Citation30] MT genes, whereas those of mammalian MT genes are generally G + C rich. Both HsMT promoters harbored canonical TATAA boxes that are similar to that of rainbow trout and crucian carp [Citation30], and stone loach [Citation27], whereas icefish [Citation28] and pike [Citation27] possess variant TTTAA substitutes. The alignment of HsMT1 and HsMT2 promoter regions showed only 36.5% identity, which is significantly lower than that of the ccMT-I and ccMT-II promoters (64%) [Citation29]. Furthermore, the position and number of putative cis-acting elements markedly differed in the HsMTs promoters. Similar features have been observed in icefish, which harbored MT-I and MT-II promoters [Citation28].
Compared to the putative cis-acting elements in the promoter regions of HsMTs, the number and position of the MREs show major differences between the two isoforms. The structure of the two promoter sequences revealed that HsMT1 contained one MRE copy (TGCGCAC), whereas HsMT2 harbored two copies of the MREs (TGCACAC and TGCGCAC) upstream of the ATG start codon. In Pacific oyster, one and two MRE copies have been identified in CgMT4 [Citation19] and CgMT1 [Citation33], respectively. It has been reported that two copies of MREs might occupy more MTFs than having one copy such as that in Pacific oyster [Citation19]. Meanwhile, the main nucleotide difference among the three MRE-core is the fourth base, which is an A in the HsMT2 proximal MRE and a G in the HsMT1 MRE and the distal MRE HsMT2. Previous studies have demonstrated that the A nucleotide is associated with greater MTF-1 binding activity toward the MRE elements from rat liver nuclear extracts [Citation34]. Furthermore, researchers have reported that limited concentrations of MTF are bound to MREs harboring an A at the fourth position such as the rainbow trout MT-B MREa sequence (TGCACAC, underlined base indicated the fourth position) [Citation6] and the two copies of MRE (TGCACAC) in CgMT1 [Citation35]. Once the concentrations of MTF increase in cells during metal stress, binding could occur between MREs harboring a G at the fourth position and MTF. Thus, a significantly higher MT expression is induced [Citation6]. As mentioned above, the presence of two copy numbers and critical sequence of MREs (TGCACAC and TGCGCAC) in the promoter region of HsMT2 may indicate that low concentration of the MTF would induce its expression, and may elicit high inducible expression level in response to different environmental stress.
Bioinformatics analysis has revealed that some other potential TFBs such as C/EBP, c-Myb, AP-3, GATA, NF-E1, Oct-1, and NF-IL6 occur in both HsMTs promoters. These factors play regulatory roles in the transcription MT genes such as C/EBP in prostate cancer cells [Citation36], TATA boxes in human MT-I gene expression [Citation37], and NF-IL6 in hepatocytes [Citation5,38]. It thus could be inferred that the transcription of HsMT1 and HsMT2 are likely regulated by various signal pathways that are similar to its mammalian counterparts.
HsMT promoters activity in HepG2 cells
Assessment of the promoter activity of HsMT1 and HsMT2 revealed that both were induced by various concentrations of heavy metals in human hepatoblastoma cells. Furthermore, the results show that Cu2+ serves as much stronger inducer of HsMT1 expression compared to Zn2+ and Cd2+, whereas the highest HsMT2 expression was observed with Zn2+ in human hepatoblastoma cells. This response also followed a dosage-dependent fashion, although the addition of a high concentration of Cd2+ resulted in a decrease in promoter activity. It is possible that Cd2+ is a non-essential heavy metal that is highly toxic to cells when applied at higher concentrations. However, species-specific differences in promoter activity have also been reported such as that in the crucian carp ccMT-I gene, in which a high concentration of Cu2+ results in a decrease in promoter activity [Citation29].
Analysis of truncated promoters shows that the removal of the MRE of HsMT1 and the distal (>500 bp) MRE of HsMT2 results in a significant reduction in metal inducibility. In the present study, the highest levels of HsMT1 and HsMT2 promoter activity were observed using the longest constructs and without the deletion of any putative cis-regulatory elements. This finding suggests that the metal inducibility of the two promoters is dependent on the presence of the MRE in HsMT1 and the distal MRE in HsMT2. Mutant pMT2-541 with a proximal MRE alone showed minimal activity in the presence of metals, which is similar to that in the mutant pMT2-136 that did not possess any MREs. The induction of the rainbow trout MT-A gene is thus required for the cooperation of both the proximal and distal MREs [Citation27,30]. Previous studies have also revealed that two or more copies of MRE are necessary for metal induction in vertebrates. For example, zebrafish MT-II (zMT-II) requires MREc and MREd [Citation26], mouse MT-I needs at least two copies of MREs [Citation39,40], and human MT-IIA is dependent on four or more MREs [Citation41] to achieve significant level of metal ion induction. However, independent and/or cooperative roles of the proximal and distal MREs in HsMT2 should be further explored in future. Taken together, both HsMT1 and HsMT2 promoters that contain MREs could be responsive to metal ions, and MREs possibly play critical roles in the induction of MT expression.
HsMTF-like regulates HsMT1 and HsMT2 expression via MREs
To further study this particular regulatory mechanism, we searched for trans-acting factors that regulate MT expression such as the metal responsive transcription factor. Previous studies have indicated that MTF-1 functions as the main activator of MT transcription by binding to the MRE core sequences within the MT promoter regions [Citation28,42]. A mussel cDNA encoding a protein reminiscent of human MTF-1 has been identified in the gonad transcriptome of H. schlegelii [Citation25]. As an important transcription factor, MTF-1 is involved in the expression of genes that have multiple MREs in their promoters [Citation9,10]. The sizes of MRE-binding proteins vary from 28 to 116 kDa in mouse and human [Citation9]. Our study have shown that the HsMTF-like gene encodes a smaller size of protein (57.32 kDa) compared to its homologs in human, mouse, or fish. We deduce that HsMTF-like protein may be an isoenzyme of MTF1 family. A similar phenomenon had also been reported in tilapia, which possesses two forms of MTF-1s (long and short MTF-1s, namely, MTF-1L and MTF-1S) [Citation9]. Zinc-finger domains bind to DNA and recognize the sequence of MRE [Citation43]. Bioinformatics analysis indicates that the zinc fingers of HsMTF-like are moderately similar to that of the other species. Furthermore, we deduce that six zinc fingers in HsMTF-like interact with the MREs of the HsMTs promoter for transcriptional regulation.
The present study observed HsMTs expression after co-transfection of the HsMTF-like expression vector and HsMTs promoter reporter vectors. A significant reduction in HsMT1 expression was detected when the MRE was mutated. Meanwhile, mutation of the two MREs (or only MREb) nearly abolished HsMT2 promoter activity. It is indicated that the stimulatory effect of HsMTF-like on the HsMTs promoters activity is mediated by MRE motifs. At the same time, it is deduced that HsMTF-like have an effect on HsMTs expression through binding to MREs. Furthermore, the MREa- or MREb-mutated promoters of HsMT2 presented different activity. As shown in Figure (E), the MREb-mutated promoter showed little activity, but the MREa-mutated promoter had most activity of the non-mutated promoter. This supports the conclusions in the previous reports that the activity of MREs in the promoter region is different and depends on its sequence and position [Citation44]. Similar regulation mechanism has also been reported in CgMT1 expression in Pacific oyster which is regulated by CgMTF [Citation45]. Meanwhile, MTF orthologs are also found in oyster genome, its protein binds to MREs and directly regulate MT expression under heavy metals exposure [Citation19]. The CgMT1 has also contained two MREs and shows different contributions for the activation of CgMT1 gene although they have the same sequence, TGCACAC [Citation45].
Numerous metal ions can activate the promoter of the MT gene via MREs, but only zinc is specific for the binding and activation of MTF-1 [Citation46]. Interestingly, cotransfection of pcDNA-HsMTF-like and pMT1-1121 or pMT2-1121 yielded the strong basal transcription that could be further boosted by zinc treatment. This phenomenon partly indicate that HsMTF-like is a zinc sensor and zinc may play important roles for HsMTs induction. This is consistent with the previous report that MTF-1 directly bind with zinc and induce MT gene expression in vertebrates [Citation16]. Therefore, it was speculated that MTFs in mollusks shared the conserved mechanism for MTs activation in vertebrates. As had already been reported, similar evolutionarily conserved mechanism could be seen in invertebrate (e.g. Drosophila [Citation8]) and vertebrate (e.g. human [Citation13]). Certainly, further investigation is required for molluscan MT regulation mechanisms.
In sum, we cloned and analyzed two metallothionein promoters of the freshwater pearl mussel, H. schlegelii. Both promoters showed differences in structural organization and metal inducibility. The mussel HsMTF-like was determined to be capable of modulating HsMTs transcription, and the MREs in its promoter mediate this activation process.
Author contributions
Conceived and designed the experiments: Yijiang Hong and Chengyuan Wang. Performed the experiments: Chengyuan Wang, Fuxing Shu, Kou Peng, Junqing Sheng and Jianwu Shi. Analyzed the data: Chengyuan Wang, Junhua Wang, Di Wu, Beijuan Hu and Shaoqing Jian. Prepared the paper: Yijiang Hong and Chengyuan Wang.
Disclosure statement
No potential conflict of interest was reported by the authors.
Funding
This work was supported by the Earmarked Fund for Modern Agro-industry Technology Research System [grant number CARS-49], [grant number JXARS-10]; the Graduate Innovative Special Fund of Jiangxi Province [grant number YC2016-B011]; the Natural Science Foundation of China [grant number 31660337]; the Key Scientific and Technological Program of Jiangxi Province, China [grant number 20152ACF60013], [grant number KJLD12001].
References
- Kaegi JHR, Schaeffer A. Biochemistry of metallothionein. Biochemistry. 1988;27:8509–8515.10.1021/bi00423a001
- Kagi JHR. Overview of metallothionein. Methods Enzymol. 1991;205:613–626.10.1016/0076-6879(91)05145-L
- Roesijadi G. Metal transfer as a mechanism for metallothionein-mediated metal detoxification. Cell Mol Biol (Noisy-le-grand). 2000;46(2):393–405.
- Sarkar A, et al. Molecular Biomarkers: Their significance and application in marine pollution monitoring. Ecotoxicology. 2006;15(4):333–340.10.1007/s10646-006-0069-1
- Haq F. Signaling events for metallothionein induction. Mutation Res. 2003;533(1–2):211–226.10.1016/j.mrfmmm.2003.07.014
- Susan LA, Samson LG. Metal-responsive elements of the rainbow trout metallothionein-B gene function for basal and metal-induced activity. J Biol Chem. 1995;270:6864–6871.
- Andrews GK. Cellular zinc sensors:MTF-1 regulation of gene expression. Biometals. 2001;14:223–237.10.1023/A:1012932712483
- Zhang B, Egli D, Georgiev O. The drosophila homolog of mammalian zinc finger factor MTF-1 activates transcription in response to heavy metals. Mol Cell Biol. 2001;21(14):4505–4514.10.1128/MCB.21.14.4505-4514.2001
- Samson SLA, Gedamu L. Molecular analyses of metallothionein gene regulation. Nucleic Acids Res. 1997;59:257–288.
- Seguin C. A nuclear factor requires Zn2+ to bind a regulatory MRE element of the mouse gene encoding metallothionein-1. Gene. 1991;97:295–300.
- Westin G, Schaffner W. A zinc-responsive factor interacts with a metal-regulated enhancer element (MRE) of the mouse metallothionein-I gene. The EMBO Journal. 1988;7:3763–3770.
- Radtke F, Heuchel R, Georgiev O, et al. Cloned transcription factor MTF-1 activates the mouse metallothionein I promoter. The EMBO J. 1993;12:1355–1362.
- Brugnera E, Georgiev O, Radtke F, et al. Cloning, chromosomal mapping and characterization of the human metal-regulatory transcription factor MTF-1. Nucleic Acids Res. 1994;22(15):3167–3173.10.1093/nar/22.15.3167
- der Maur AA, Belser T, Elgar G, et al. Characterization of the transcription factor MTF-1 from the Japanese pufferfish (Fugu rubripes) reveals evolutionary conservation of heavy metal stress response. Biol Chem. 1999;380:175–185.
- Chen W, John JA, Lin CH, et al. Molecular cloning and developmental expression of zinc finger transcription factor MTF-1 gene in zebrafish, Danio rerio. Biochem Biophys Res Commun. 2002;291(4):798–805.10.1006/bbrc.2002.6517
- Chen W, John JA, Lin CH, et al. Expression pattern of metallothionein, MTF‐1 nuclear translocation, and its dna‐binding activity in zebrafish (Danio rerio) induced by zinc and cadmium. Environ Toxicol Chem. 2007;26:110–117.10.1897/06-153R.1
- Cheung AP, Au CY, Chan WW, et al. Characterization and localization of metal-responsive-element-binding transcription factors from tilapia. Aquat Toxicol. 2010;99(1):42–55.10.1016/j.aquatox.2010.03.017
- Ferencz A, Hermesz E. Identification and characterization of two mtf-1 genes in common carp. Comp Biochem Physiol C Toxicol Pharmacol. 2008;148(3):238–243.10.1016/j.cbpc.2008.06.001
- Meng J, Zhang L, Li L, et al. Transcription factor CgMTF-1 regulates CgZnT1 and CgMT expression in Pacific oyster (Crassostrea gigas) under zinc stress. Aquat Toxicol. 2015;165:179–188.10.1016/j.aquatox.2015.05.023
- Bittel D, Dalton T, Samson SL, et al. The DNA binding activity of metal response element-binding transcription factor-1 is activated in vivo and in vitro by zinc, but not bu other transition metals. Biol Chem. 1998;273:7127–7133.10.1074/jbc.273.12.7127
- Drechsel V, Schauer K, Šrut M, et al. Regulatory plasticity of earthworm wMT-2 gene expression. Int J Mol Sci. 2017;18(6):1113.
- Hockner M, Dallinger R, Sturzenbaum SR. Metallothionein gene activation in the earthworm (Lumbricus rubellus). Biochem Biophys Res Commun. 2015;460(3):537–542.10.1016/j.bbrc.2015.03.065
- Boening D. An evaluation of bivalves as biomonitors of heavy metals pollution in marine waters. Environ Monit Assess. 1999;55:459–470.10.1023/A:1005995217901
- Wang C, Sheng J, Hong Y, et al. Molecular characterization and expression of metallothionein from freshwater pearl mussel, Hyriopsis schlegelii. Biosci Biotechnol Biochem. 2016;80(7):1327–1335.10.1080/09168451.2016.1153954
- Shi J, Hong Y, Sheng J, et al. De novo transcriptome sequencing to identify the sex-determination genes in Hyriopsis schlegelii. Biosci Biotechnol Biochem. 2015;79(8):1257–1265.10.1080/09168451.2015.1025690
- Yan CHM, Chan KM. Cloning of zebrafish metallothionein gene and characterization of its gene promoter region in HepG2 cell line. Biochim. Biophys. Acta. 2004;1679(1):47–58.10.1016/j.bbaexp.2004.04.004
- Olsson PE, Kille P. Functional comparison of the metal regulated transcriptional control regions of metallothionein genes from cadmium sensitive and tolerant fish species. Biochim Biophys Acta. 1997;1350:325–334.10.1016/S0167-4781(96)00173-X
- Scudiero R, Carginale V, Capasso C, et al. Structural and functional analysis of metal regulatory elements in the promoter region of genes encoding metallothionein isoforms in the Antarctic fish Chionodraco hamatus (icefish). Gene. 2001;274:199–208.10.1016/S0378-1119(01)00609-6
- He P, Xu M, Ren H. Cloning and functional characterization of 5′-upstream region of metallothionein-I gene from crucian carp (Carassius cuvieri). Int J Biochem Cell Biol. 2007;39(4):832–841.10.1016/j.biocel.2007.01.008
- Ren H, Xu M, He P, et al. Cloning of crucian carp (Carassius cuvieri) metallothionein-II gene and characterization of its gene promoter region. Biochem Biophys Res Commun. 2006;342(4):1297–1304.10.1016/j.bbrc.2006.02.082
- Lee SY, Nam YK. Transcriptional responses of metallothionein gene to different stress factors in Pacific abalone (Haliotis discus hannai). Fish Shellfish Immunol. 2016;58:530–541.10.1016/j.fsi.2016.09.030
- Takahashi S. Positive and negative regulators of the metallothionein gene (Review). Mol Med Rep. 2015;12(1):795–799.10.3892/mmr.2015.3459
- Arnaud Tanguy CM. Dario Moraga, Cloning of a metallothionein gene and characterization of two other cDNA sequences in the Pacific oyster Crassostrea gigas (CgMT1). Aquat Toxicol. 2001;55:35–47.10.1016/S0166-445X(01)00160-6
- Searle PF. Zinc dependent binding of a liver nuclear factor to metal response element MRE-a of the mouse metallothionein-I gene and variant sequences. Nucleic Acids Res. 1990;18:4683–4690.10.1093/nar/18.16.4683
- Arnaud Tanguy DM. Cloning and characterization of a gene coding for a novel metallothionein in the Pacific oyster Crassostrea gigas (CgMT2): a case of adaptive response to metal-induced stress? Gene. 2001;273:123–130.10.1016/S0378-1119(01)00577-7
- Yin H, Smith M, Glass J. Stable expression of C/EBPalpha in prostate cancer cells down-regulates metallothionein and increases zinc-induced toxicity. Prostate. 2005;62(3):209–216.10.1002/(ISSN)1097-0045
- Shworak NW, O’Connor T, Wong NC, et al. Distinct TATA motifs regulate differential expression of human metallothionein I genes MT-IF and MT-IG. J Biol Chem. 1993;268(32):24460–24466.
- Schroeder JJ, Cousins RJ. Interleukin 6 regulates metallothionein gene expression and zinc metabolism in hepatocyte monolayer cultures. Proc Natl Acad Sci. 1990;87:3137–3141.10.1073/pnas.87.8.3137
- Searle PF, Stuart GW, Palmiter RD, et al. Building a metal-responsive promoter with synthetic regulatory elements. Mol Cell Biol. 1985;1480–1489.10.1128/MCB.5.6.1480
- Stuart GW, Searle PF, Palmiter RD. Identification of multiple metal regulatory elements in mouse metallothionein-I promoter by assaying synthetic sequences. Nature. 1985;317(6040):828–831.10.1038/317828a0
- Koizumi S, Suzuki K, Ogra Y, et al. Transcriptional activity and regulatory protein binding of metal-responsive elements of the human metallothionein-IIA gene. Eur J Biochem. 1999;259:635–642.
- Hockner M, Stefanon K, Schuler D, et al. Coping with cadmium exposure in various ways: the two Helicid snails Helix pomatia and Cantareus aspersus share the metal transcription factor-2, but differ in promoter organization and transcription of their Cd-metallothionein genes. J Exp Zool A Ecol Genet Physiol. 2009;311A(10):776–787.10.1002/jez.564
- Murphy BJ, Andrews GK, Bittel D, et al. Activation of metallothionein gene expression by hypoxia involves metal response elements and metal transcription factor-1. Cancer Res. 1999;59:1315–1322.
- Giedroc DP, Chen X, Apuy JL. Metal response element (MRE)-binding transcription factor-1 (MTF-1): structure, function, and regulation. Antioxid Redox Signal. 2001;3(4):577–596.10.1089/15230860152542943
- Qiu J, Liu Y, Yu M, et al. Identification and functional characterization of MRE-binding transcription factor (MTF) in Crassostrea gigas and its conserved role in metal-induced response. Mol Biol Rep. 2012;40(4):3321–3331.
- Dong G, Chen H, Qi M, et al. Balance between metallothionein and metal response element binding transcription factor 1 is mediated by zinc ions (Review). Mol Med Rep. 2015;11(3):1582–1586.10.3892/mmr.2014.2969