Abstract
Green tea leaves fermented with Aspergillus luchuensis var kawachii kitahara (Cha-Koji) are a health food containing live A. luchuensis. In this study, we examined the effects of Cha-Koji on the immune system and the enteric environment. First, we designed a clinical trial; after ingesting Cha-Koji daily for 28 days, blood parameters and the fecal composition of the participants were analyzed. Similarly, mice were administered (oral administration) with Cha-Koji suspension or its vehicle for 14 days. Thereafter, both humans and mice were examined by analyzing their immune cell phenotypes and intestinal microbiota. Regulatory T cell (Treg) numbers were significantly increased after administering Cha-Koji. An increase of Clostridium subcluster XIVa, that were known to be rich in butyrate-producing bacterium, was observed in human feces, but not in mice. These results suggest that Cha-Koji has the ability to increase Treg production in both humans and mice, irrespective of the presence of enteric butyrate.
“Cha-Koji” increases regulatory T cells in mice and humans.
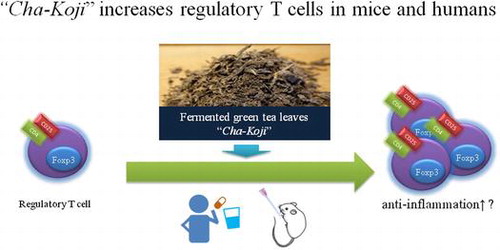
Aspergillus is traditionally used in Japanese fermented food products such as sake (Japanese rice wine), shochu (clear liquor), miso (bean paste), and soy sauce. Although Aspergillus is a well-known cause of aspergillosis and considered a toxigenic fungus in Europe [Citation1], Aspergillus oryzae and Aspergillus luchuensis do not produce mycotoxins [Citation2]. The US Food and Drug Administration (FDA) recognizes Koji as Generally Regarded as Safe (GRAS). Furthermore, the Brewing Society of Japan have designated A. oryzae and A. luchuensis as national fungi. These fungal species are safe and commonly prevalent in Japanese food life. Previous studies have reported the effects of A. oryzae on metabolism, microbiota, and the immune system [Citation3–6]. However, there are no reports on the effects of A. luchuensis in humans. Cha-Koji is prepared using green tea leaves fermented with A. luchuensis, supplemented with several Lactobacillus species (L. sakei, L. acidophilus, L. casei, L. plantarum) and B. longum. Cha-Koji has been commercially sold for over 5 years, and although some consumers have reported weak allergic reactions, no hazardous phenomena were reported. We speculate that Cha-Koji may exert effects on immune tolerance.
Regulatory T cells (Tregs), which are well known for maintaining immune tolerance [Citation7], are a special subset of T cells that prevent other immune cells from attacking the body’s own tissues and other harmless environmental materials such as food and commensal organisms. It is said that many diseases, such as atopic disease [Citation8], inflammatory bowel disease [Citation9], and ischemic heart disease (IHD) [Citation10,11] can be treat by controlling Tregs.
Changing the body’s microbiota through the administration of probiotics can induce the expression of Tregs. For example, it has been reported that Clostridiales species, which produce butyrate, increase the expression of Tregs [Citation12–14]. Zimmer et al. showed that fungal proteases derived from A. oryzae induce the production of tolerogenic human dendritic cells, resulting in increased Tregs [Citation15]. Therefore, we hypothesized that Cha-Koji has the ability to increase the production of Tregs.
The purpose of this study was to investigate whether Cha-Koji affects the intestinal microbiota and increases Treg numbers or frequency in humans and mice. From an immunological viewpoint, we also analyzed the spleen and mesenteric lymph nodes (MLNs) in mice.
Materials and methods
Cha-Koji
Green tea leaves fermented with A. luchuensis var kawachii kitahara (Cha-Koji) were provided by the Genkoji Research Institute Co., Ltd. (Kagoshima, Japan). Lactobacillus. sakei HS-1 (1 × 106 CFU/g), Lactobacillus. acidophilus LAGL1 (1 × 106 CFU/g), Lactobacillus. casei LC107 (2.5 × 106 CFU/g), Lactobacillus. plantarum LP375 (2.5 × 106 CFU/g), and Bifidobacterium. longum BL730 (2.5 × 106 CFU/g) were added to the Cha-Koji samples. The samples also contained 36.9 g/100 g dietary fiber and 0.8 mg/100 g epigallocatechin-3-gallate (EGCG; measured at Japan Food Research Laboratories, Tokyo, Japan). Human subjects ingested 2.14 g of Cha-Koji per day with water for 28 days. Mice were administered 150 μL per day of 5 mg/ml Cha-Koji in phosphate buffered saline (PBS) by oral gavage.
Reagents for flow cytometry
The reagents used for this study were as follows: antibodies for humans: anti-CD3-Pacific Blue (17A2), anti-CD4-FITC (SK3), anti-CD25-Alexa700 (BC96), and anti-CD127-PE (A019D5); antibodies for mice: anti-CD3-Alexa700 (17A2), anti-CD4-Pacific blue (GK 1.5), anti-FOXP3-PE (150D) (BioLegend, San Diego, CA, USA), and anti-CD25-BV650 (BD Biosciences, San Jose, CA, USA). To detect dead cells, we used 1 μg/μL propidium iodide (Sigma Aldrich, St. Louis, MO, USA). To stain FOXP3, we used the Foxp3/Transcription Factor Staining Buffer set (Thermo Fisher Scientific, Waltham, MA, USA). Immunofluorescence analysis was performed using the BD LSRFortessa flow cytometer (Becton Dickinson, Franklin Lakes, NJ, USA) and FlowJo software (v. 7.6.5).
Clinical parameters
Ten healthy adults (eight males and two females, age 36.1 ± 11.0 years, height 169 ± 8.1 cm, weight 65.1 ± 10.8 kg, mean ± SD) participated in this study. The exclusion criteria were as follows: no allergies, no serious heart, liver, renal, or autoimmune diseases, periodic use of any medicines, and no regular consumption of fermented foods. Written informed consent was obtained from all subjects after they were given an explanation of the study. This clinical study protocol was approved by the Institutional Review Board for Clinical Research, Tokai University (16R-130). Participants ingested 2.14 g of Cha-Koji per day for 28 days. Nine participants completed this study. One female individual was excluded from further analysis because she traveled to a foreign country during the experimental period. Blood and fecal samples were collected before and after the experiment and stored as described below.
Human PBMCs and feces
Human PBMCs were isolated immediately after blood samples were obtained by density gradient cell separation using a BD Vacutainer CRT (BD Biosciences). PBMCs were suspended in the cell preservative solution TC-Protector (DC Pharma, Wolfen, Germany) and frozen at −80 °C on the first day, and then stored at −196 °C in liquid nitrogen thereafter. All PBMCs were thawed and the proportion of Tregs in each sample was measured by flow cytometry. Fecal samples were collected and stored at −80 °C. Next, we measured the concentration of fecal butyrate and analyzed the microbiota for all samples. Measurements were performed by Techno Suruga Laboratory Co., Ltd. (Shizuoka, Japan). Short-chain fatty acids (SCFAs) in feces were analyzed using a modified method, as described previously by García-Villalba et al. [Citation16]. The microbial composition of feces was determined using Terminal Restriction Fragment Length Polymorphism (T-RFLP) as previously described by Jin et al. [Citation17].
Staining protocol and detection of Tregs in mice
All samples were prepared as 1 × 106 peripheral mononuclear blood cells (PBMCs) per test and suspended in 40 μL of PBS. Cells were incubated at 4 °C in the dark for 60 min with the following antibodies: 0.2 μL of anti-CD3, 2.0 μL anti-CD4, 0.5 μL of anti-CD25, and 0.4 μL of anti-CD127. Cells were washed twice with 1 mL PBS and resuspended in 200 μL of PBS. Before analysis, 2 μL of propidium iodide solution was added. To detect Tregs, first, we excluded dead cells by selecting cells with low concentrations of propidium iodide, and the area of lymphocytes was analyzed in a forward scatter (FSC) and side scatter (SSC) view. Next, cells positive for both CD3 and CD4 were selected. Then, Tregs were detected as CD25bright and CD127dull populations.
Mice
C57BL/6 mice (female, 8–10 weeks old) were obtained from CLEA Japan, Inc. (Tokyo, Japan). Mice were maintained under specific pathogen-free (SPF) conditions with a 12-h light-dark cycle in the Department of Laboratory Animal Science at Tokai University; the mice were adapted to their environment over one week. After adaptation, the mice were randomly divided into two groups (n = 16 per group), and fed Cha-Koji suspension or PBS. Each group of mice was fed 150 μL Cha-Koji suspension (containing 0.75 mg Cha-Koji) or PBS daily for 14 days. At the end of the experimental period, mice were sacrificed by exsanguination under 2% Isoflurane anesthesia. Tissue samples, the spleen, and the mesenteric lymph node (MLN) were removed immediately. This experimental protocol was approved by the Institutional Animal Care and Use Committee at Tokai University (172006).
PBMCs, splenocytes, and mesenteric lymphocytes of mice
Murine PBMCs were collected by density gradient cell separation, using the HISTPAQUE-1083 (Sigma Aldrich). The spleen and MLN were removed and separated by gently pressing the organs through a 40-μm cell strainer. Red blood cells were lysed with ammonium chloride buffer. Cell numbers were measured with a CDA-1000 counter (Sysmex, Kobe, Japan).
Staining protocol and detection of Tregs in mice
All samples were prepared as 1 × 106 peripheral mononuclear blood cells (PBMCs) per test and suspended in 40 μL of PBS. Cells were incubated at 4 °C in the dark for 40 min with the following antibodies: 1 μL of anti-CD3, anti-CD4, and anti-CD25. Then, cells were washed twice with 1 mL of PBS and exposed to the Foxp3/Transcription Factor Staining Buffer kit with 5 μL of Anti-Foxp3 antibody. After Foxp3 staining, cells were resuspended in 200 μL of PBS and analyzed by a flow cytometer. To detect Tregs, cells positive for both CD3 and CD4 were selected. Then CD25bright and Foxp3-positive Treg populations were detected.
Measurement of SCFAs and microbiota of murine cecum feces
Murine feces were collected from the cecum under sterile conditions and stored at -80 °C. The concentration of murine SCFAs and the microbiota were analyzed by TechnoSuruga Laboratory Co., Ltd. (Shizuoka, Japan), as described above. As some samples were potentially contaminated, only ten fecal samples were analyzed.
Statistical analysis
All data are shown as the mean ± SEM. The Wilcoxon signed-rank test was performed for the human study, and the Mann-Whitney U test was performed for the mouse study. Graph generation and statistical analysis were performed using Prism software version 5 (GraphPad). Values of *p < 0.05 or **p < 0.01 were considered to represent statistical significance.
Results
Cha-Koji elevates Treg production in humans
Our analysis of Tregs (Figure and Figure S1) showed that the ratio of Tregs to lymphocytes among PBMCs was significantly increased after administering Cha-Koji (pre: 1.50 ± 0.30%, post: 1.95 ± 0.35%, p < 0.01; Figure and Figure S2). There were no significant changes detected via the general blood biochemical examination, and the participants had no complaints of health problems (Table ).
Figure 1. Regulatory T cells (Tregs) were detected in CD4+, CD25bright, and CD127dull cells among human peripheral blood mononuclear cells (PBMCs).
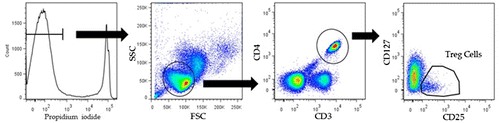
Figure 2. The change in Tregs in PBMCs after 28 days of Cha-Koji ingestion (triangles) compared with those at the start of the experiment (circles). (A) The change in the ratio of Tregs to T cells (B) The change in the number of Tregs.
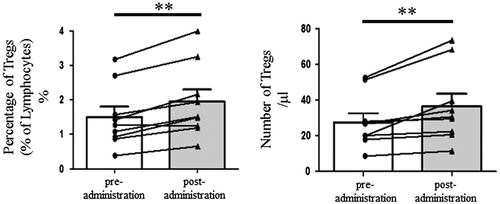
Table 1. The results of blood biochemical examinations.
Alteration of gut microbiota and fecal butyrate levels after ingesting Cha-Koji
To ascertain whether Cha-Koji changed the composition of the intestinal microbiota, we evaluated the fecal microbiota of participants. Interestingly, the percentage of Clostridium subcluster XIVa, which is related to butyrate production, was significantly increased (p < 0.05; Table ). Measurement of SCFAs in feces showed that Cha-Koji administration for 28 days resulted in an overall decrease in acetate (pre: 29.89 ± 3.36 μmol/g, post: 27.37 ± 1.93 μmol/g), and propionate (pre: 12.31 ± 1.56 μmol/g, post: 10.51 ± 1.28 μmol/g) levels, as well as an increase in butyrate (pre: 7.21 ± 1.13 μmol/g, post: 9.10 ± 1.40 μmol/g) levels; however, these results were not statistically significant (Figure ).
Table 2. Changes in the bacterial profile of feces in human participants after 4 weeks Cha-Koji administration.
Cha-Koji increases Treg cells in murine PBMCs and spleens
To further investigate the effects of Cha-Koji, we also measured the number and subtypes of immune cells in mice (Figure ). Cha-Koji administration resulted in a significant increase in the Treg ratio for all T cells in PBMCs (PBS: 1.34 ± 0.12%, Cha-Koji: 1.71 ± 0.12%, p < 0.05; Figure (A)) and splenocytes (PBS: 1.18 ± 0.08%, Cha-Koji: 1.39 ± 0.06%, p < 0.05; Figure (B)). In splenocytes, the ratio of Tregs to CD4+ T cells was also increased (data not shown). However, the ratio of Tregs in MLNs did not increase (Figure S3).
Cha-Koji changes the microbial profile of mice but does not increase butyrate levels
We additionally measured the cecal contents of mice and analyzed their microbiota and SCFAs. Interestingly, the proportion of Clostridium subcluster XIVa did not differ between groups. Clostridium cluster XI in the Cha-Koji group showed a significant increase compared with that in the PBS group (Table ). This result was different from that of the human trial. Measurement of the SCFA content in cecum samples showed no changes in acetate, butyrate, or propionate levels (Figure ).
Table 3. Changes in the bacterial profile of cecum contents in mice after 14 days Cha-Koji administration.
Discussion
The purpose of this study was to investigate the effects of Cha-Koji in humans and mice. We found that oral administration of Cha-Koji, green tea leaves fermented with A. luchuensis, resulted in increased Tregs in human PBMCs, murine PBMCs and splenocytes. Moreover, we found that Cha-Koji affected the composition of the intestinal microbiota of humans and mice. An increase in the proportion of Clostridium subcluster XIVa was particularly observed in human feces. Furthermore the proportion of Clostridium cluster XI was increased in murine cecum contents. This is the first report showing that the consumption of food containing live A. luchuensis elicits an increase in Tregs and alters the intestinal microbiota in humans and mice, which may prevent Treg-related diseases.
In recent years, SCFAs have received much attention. Specifically, butyrate is known to inhibit histone deacetylases and induce the production of Tregs [Citation13,18]. Bacteria that are classified under Clostridium subcluster XIVa are known to produce butyrate. In this study, we found that the ingestion of Cha-Koji increased the abundance of Clostridium subcluster XIVa in human feces. We anticipated that this effect also occurs in mice. However, in the mouse study, the ratio of Tregs among PBMCs and splenocytes was increased, while the abundance of Clostridium subcluster XIVa and the fecal concentration of butyrate was unchanged. This may be attributable to differences in the intestinal microbiota between humans and mice. For example, Segmented filamentous bacteria, which is known to maintain murine Th17 cells, have not been detected in the human [Citation19]. In our study, Lactobacillales and Clostridium cluster IV were not found in mice. As above, the intestinal microbiota of humans and mice differs from each other; accordingly, the changes in the intestinal microbiota caused by Cha-Koji may vary between mice and humans. Furthermore the difference in the relative amount of dietary fiber from Cha-koji to daily intake between human and mice might attribute to this different result. As many Clostridiales are known to utilize dietary fiber for their energy source, an increase in dietary fiber intake is expected to cause an increase of Clostridiales. In mice, dietary fiber in their chow is almost 100–150 mg/day. Cha-Koji administration added 0.28 mg of dietary fiber intake. This amount is calculated as 0.28% increase and within the error margin. In human, the daily average intake of dietary fiber by Japanese adults is about 15 g [Citation20]. Cha-Koji administration added 777 mg of dietary fiber intake. This amount corresponds to approximately 5% of daily dietary fiber intake. Thus, the relative difference in dietary fiber intake might result in the variation of microbiota change. Furthermore, the increase in Tregs in response to Cha-Koji ingestion may not be caused by butyrate.
Probiotics such as Lactobacillus spp. and Bifidobacterium spp., which are added to Cha-Koji, have been reported to regulate Tregs in humans and mice [Citation21–23]. The presence of these probiotics may, therefore, be one of the causes of the observed increase in Tregs. However, almost all previous studies have used probiotics at a concentration of more than 1 × 106 CFU/dose, whereas in our study, all bacteria, except A. luchuensis, were administered at concentrations of under 1 × 104 CFU/dose to mice. There is no evidence showing an enhancement of Tregs at such a low dose.
However, a previous study demonstrated that fungal proteases from A. oryzae induce the production of tolerogenic dendritic cells [Citation15]. Moreover, Stephen-Victor et al. reported that α-(1,3)-glucan polysaccharide from A. fumigatus stimulated the polarization of Tregs by inducing PD-L1 expression in human dendritic cells [Citation24]. These data suggest that the metabolites of A. Luchuensis or components of Cha-Koji might cause an increase in the production of Tregs. In our preliminary test, administration of heat killed Cha-koji had not induced the increase of Tregs (data not shown). We think this outcome supports our consideration.
In addition to probiotics, EGCG, which is rich in green tea leaves, may also affect Tregs. Many investigators reported that EGCG plays a role in regulating Tregs in vivo [Citation25–29]. However, because Cha-Koji contained EGCG at a very low concentration in this study (0.8 mg/100 g), its influence could not be determined. Although numerous substances capable of inducing the production of Tregs are present in Cha-Koji, the mechanisms underlying the effects of Cha-Koji observed in the present study remain to be elucidated. Kwon et al. reported that mixed probiotics were the most effective treatment to promote the production of Tregs [Citation30]. It suggests that the complex of probiotics present in foods such as Cha-Koji is suitable for increasing Treg production.
There were several limitations to this study. As the present investigation was a pilot study, the overall sample size for the human participants was relatively small. Additionally, we could not include a placebo group in the human study. Furthermore, a diet containing only A. luchuensis was not administered to any of the groups. Therefore, it is unclear whether A. luchuensis was necessary to induce Tregs. Tregs are generally classified into two types. One is naturally occurring regulatory T cell (nTreg) derived from thymus. Another is inducible regulatory T cell (iTreg) derived from peripheral tissues (e.g. intestinal tract). In this study, it is unclear which type of Treg was increased. Furthermore, their antigenic specificity remains unclear. In our study Tregs in MLNs, which was considered to be rich in Cha-Koji derived antigens, were not increased (Figure S3). It is possible that the specificity of Tregs induced by Cha-Koji administration was not limited to Cha-Koji derived antigens reaction. Further studies are needed to elucidate these points.
Despite these limitations, the present study demonstrates that Cha-Koji consumption increased Tregs in both humans and mice, with a potential changing its intestinal microbiota. These effects may prevent the onset of Treg-related diseases such as atopic disease, inflammatory bowel disease and ischemic heart disease.
Conclusion
In summary, our study provides evidence that Cha-Koji, which comprises green tea leaves fermented with A. luchuensis, modulates the composition of the intestinal microbiota and increases Tregs among PBMCs in humans. Furthermore, Cha-Koji induced an increase in Tregs among murine PBMCs and splenocytes. These findings suggest that Cha-Koji may have positive effects on human health. Future studies should aim to further elucidate these effects in a mouse disease model.
Author Contribution
B. Y. and S. I. conceived and designed the experiments. B. Y., Y. S., T. Y., N. M., N. W. and S. I. performed the experiments. B. Y. wrote the paper. B. Y., Y. S., T. Y., N. M., N. W., T. S., S. I. and S. I discussed the results, commented on the manuscript, and approved the manuscript submission.
Funding
This research was partially supported by the Research Project for Improving Quality in Healthcare and Collecting Scientific Evidence on Integrative Medicine from Japan Agency for Medical Research and Development, AMED [grant number 17lk0310037h0001].
Supplemental data
Supplemental data for this article can be accessed https://doi.org/10.1080/09168451.2018.1443789
Disclosure statement
No potential conflict of interest was reported by the authors.
cha-koji_supplemental_data.pdf
Download PDF (255 KB)Acknowledgments
We received extensive technical support and advice from Dr. Yoshiaki Okada and Yumiko Iida during the flow cytometry studies. Katsuko Naito and Akemi Kamijo fed Cha-Koji to the mice. Yoshiko Shinozaki collected mouse blood. The above are all members of the Support Center for Medical Research and Education of Tokai University. We would like to thank Editage (www.editage.jp) for English language editing.
References
- Medina A, Valle-Algarra FM, Mateo R, et al. Survey of the mycobiota of Spanish malting barley and evaluation of the mycotoxin producing potential of species of Alternaria, Aspergillus and Fusarium. Int J Food Microbiol. 2006;108:196–203.10.1016/j.ijfoodmicro.2005.12.003
- Hong SB, Lee M, Kim DH, et al. Aspergillus luchuensis, an industrially important black Aspergillus in East Asia. PLoS One. 2013;8(5):e63769. DOI:10.1371/journal.pone.0063769. PubMed PMID: 23723998; PubMed Central PMCID: PMC3665839.
- Jang EK, Kim NY, Ahn HJ, et al. Gamma-Aminobutyric Acid (GABA) production and Angiotensin-I Converting Enzyme (ACE) inhibitory activity of fermented soybean containing sea tangle by the co-culture of lactobacillus brevis with aspergillus oryzae. J Microbiol Biotechnol. 2015 Aug;25(8):1315–1320. DOI:10.4014/jmb.1412.12038 . PubMed PMID: 25876604.
- Kataoka K, Kibe R, Kuwahara T, et al. Modifying effects of fermented brown rice on fecal microbiota in rats. Anaerobe. 2007 Oct–Dec;13(5–6):220–227. DOI:10.1016/j.anaerobe.2007.07.001. PubMed PMID: 17826198.
- Nemoto H, Ikata K, Arimochi H, et al. Effects of fermented brown rice on the intestinal environments in healthy adult. J Med Investig. 2011 Aug;58(3–4):235–245 . PubMed PMID: 21921425.10.2152/jmi.58.235
- Yang Y, Iwamoto A, Kumrungsee T, et al. Consumption of an acid protease derived from Aspergillus oryzae causes bifidogenic effect in rats. Nutr Res. 2017 Aug;44:60–66. DOI:10.1016/j.nutres.2017.06.004. PubMed PMID: 28821318.
- Sakaguchi S, Sakaguchi N, Asano M, et al. Immunologic self-tolerance maintained by activated T cells expressing IL-2 receptor alpha-chains (CD25). Breakdown of a single mechanism of self-tolerance causes various autoimmune diseases. J Immunol. 1995 Aug 01;155(3):1151–1164. PubMed PMID: 7636184.
- Leung DY, Boguniewicz M, Howell MD, et al. New insights into atopic dermatitis. J Clin Investig. 2004 Mar;113(5):651–657. DOI:10.1172/JCI21060. PubMed PMID: 14991059; PubMed Central PMCID: PMC351324.
- Bouma G, Strober W. The immunological and genetic basis of inflammatory bowel disease. Nat Rev Immunol. 2003 Jul;3(7):521–533. DOI:10.1038/nri1132 . PubMed PMID: 12876555.
- Ait-Oufella H, Salomon BL, Potteaux S, et al. Natural regulatory T cells control the development of atherosclerosis in mice. Nat Med. 2006 Feb;12(2):178–180. DOI:10.1038/nm1343 . PubMed PMID: 16462800.
- Klingenberg R, Gerdes N, Badeau RM, et al. Depletion of FOXP3+ regulatory T cells promotes hypercholesterolemia and atherosclerosis. J Clin Investig. 2013 Mar;123(3):1323–1334. DOI:10.1172/JCI63891. PubMed PMID: 23426179; PubMed Central PMCID: PMC3582120.
- Atarashi K, Tanoue T, Shima T, et al. Induction of colonic regulatory T cells by indigenous clostridium species. Science. 2011 Jan 21;331(6015):337–341. DOI:10.1126/science.1198469. PubMed PMID: 21205640; PubMed Central PMCID: PMC3969237.
- Furusawa Y, Obata Y, Fukuda S, et al. Commensal microbe-derived butyrate induces the differentiation of colonic regulatory T cells. Nature. 2013 Dec 19;504(7480):446–450. DOI:10.1038/nature12721 . PubMed PMID: 24226770.
- Asarat M, Apostolopoulos V, Vasiljevic T, et al. Short-chain fatty acids regulate cytokines and Th17/Treg cells in human peripheral blood mononuclear cells in vitro. Immunol Investig. 2016;45(3):205–222. DOI:10.3109/08820139.2015.1122613 . PubMed PMID: 27018846.
- Zimmer A, Luce S, Gaignier F, et al. Identification of a new phenotype of tolerogenic human dendritic cells induced by fungal proteases from aspergillus oryzae. J Immunol. 2011 Apr 01;186(7):3966–3976. DOI:10.4049/jimmunol.1003184 . PubMed PMID: 21368225.
- Garcia-Villalba R, Gimenez-Bastida JA, Garcia-Conesa MT, et al. Alternative method for gas chromatography-mass spectrometry analysis of short-chain fatty acids in faecal samples. J Separat Sci. 2012 Aug;35(15):1906–1913. DOI:10.1002/jssc.201101121 . PubMed PMID: 22865755.
- Jin JS, Touyama M, Hisada T, et al. Effects of green tea consumption on human fecal microbiota with special reference to Bifidobacterium species. Microbiol Immunol. 2012 Nov;56(11):729–739. DOI:10.1111/j.1348-0421.2012.00502.x . PubMed PMID: 22924537.
- Arpaia N, Campbell C, Fan X, et al. Metabolites produced by commensal bacteria promote peripheral regulatory T-cell generation. Nature. 2013 Dec 19;504(7480):451–455. DOI:10.1038/nature12726. PubMed PMID: 24226773; PubMed Central PMCID: PMC3869884.
- Schnupf P, Gaboriau-Routhiau V, Sansonetti PJ, et al. Segmented filamentous bacteria, Th17 inducers and helpers in a hostile world. Curr Opin Microbiol. 2017 Feb;35:100–109. DOI:10.1016/j.mib.2017.03.004 . PubMed PMID: 28453971.
- Ministry of Health Law. Trends in mean daily intakes of total dietary fiber, 2001–2015 (by Gender and Age) Tokyo [cited 2018 Jan 16]. Available from: http://www.mhlw.go.jp/seisakunitsuite/bunya/kenkou_iryou/kenkou/kenkounippon21/en/eiyouchousa/kekka_eiyou_chousa_koumoku.html.
- Iemoli E, Trabattoni D, Parisotto S, et al. Probiotics reduce gut microbial translocation and improve adult atopic dermatitis. J Clin Gastroenterol. 2012 Oct;46(Suppl):S33–S40. DOI:10.1097/MCG.0b013e31826a8468 . PubMed PMID: 22955355.
- Lenoir M, del Carmen S, Cortes-Perez NG, et al. Lactobacillus casei BL23 regulates Treg and Th17 T-cell populations and reduces DMH-associated colorectal cancer. J Gastroenterol. 2016 Sep;51(9):862–873. DOI:10.1007/s00535-015-1158-9 . PubMed PMID: 26749362.
- Wang K, Dong H, Qi Y, et al. Lactobacillus casei regulates differentiation of Th17/Treg cells to reduce intestinal inflammation in mice. Can J Veterin Res. 2017 Apr;81(2):122–128. PubMed PMID: 28408780; PubMed Central PMCID: PMC5370538.
- Stephen-Victor E, Karnam A, Fontaine T, et al. Aspergillus fumigatus cell wall alpha-(1,3)-glucan stimulates regulatory T cell polarization by inducing PD-L1 expression on human dendritic cells. J Infect Dis. 2017 Sep 08. DOI:10.1093/infdis/jix469 . PubMed PMID: 28968869.
- Wong CP, Nguyen LP, Noh SK, et al. Induction of regulatory T cells by green tea polyphenol EGCG. Immunol Lett. 2011 Sep 30;139(1–2):7–13. DOI:10.1016/j.imlet.2011.04.009. PubMed PMID: 21621552; PubMed Central PMCID: PMC4125122.
- Wu D, Wang J, Pae M, et al. Green tea EGCG, T cells, and T cell-mediated autoimmune diseases. Mol Aspects Med. 2012 Feb;33(1):107–118. DOI:10.1016/j.mam.2011.10.001 . PubMed PMID: 22020144.
- Wang J, Pae M, Meydani SN, et al. Green tea epigallocatechin-3-gallate modulates differentiation of naive CD4(+) T cells into specific lineage effector cells. J Mol Med. 2013 Apr;91(4):485–495. DOI:10.1007/s00109-012-0964-2 . PubMed PMID: 23064699.
- Byun JK, Yoon BY, Jhun JY, et al. Epigallocatechin-3-gallate ameliorates both obesity and autoinflammatory arthritis aggravated by obesity by altering the balance among CD4+ T-cell subsets. Immunol Lett. 2014 Jan–Feb;157(1–2):51–59. DOI:10.1016/j.imlet.2013.11.006. PubMed PMID: 24239847.
- Mahmoud F, Al-Ozairi E, Haines D, et al. Effect of diabetea tea consumption on inflammatory cytokines and metabolic biomarkers in type 2 diabetes patients. J Ethnopharmacol. 2016 Dec 24;194:1069–1077. DOI:10.1016/j.jep.2016.10.073 . PubMed PMID: 27989874.
- Kwon HK, Lee CG, So JS, et al. Generation of regulatory dendritic cells and CD4+Foxp3+ T cells by probiotics administration suppresses immune disorders. Proc Natl Acad Sci USA. 2010 Feb 02;107(5):2159–2164. DOI:10.1073/pnas.0904055107. PubMed PMID: 20080669; PubMed Central PMCID: PMC2836639.