Abstract
As part of continued efforts for the development of new tyrosinase inhibitors, (Z)-5-(substituted benzylidene)-2-iminothiazolidin-4-one derivatives (1a – 1l) were rationally synthesized and evaluated for their inhibitory potential in vitro. These compounds were designed and synthesized based on the structural attributes of a β-phenyl-α,β-unsaturated carbonyl scaffold template. Among these compounds, (Z)-5-(3-hydroxy-4-methoxybenzylidene)-2-iminothiazolidin-4-one (1e, MHY773) exhibited the greatest tyrosinase inhibition (IC50 = 2.87 μM and 8.06 μM for monophenolase and diphenolase), and outperformed the positive control, kojic acid (IC50 = 15.59 and 31.61 μM). The kinetic and docking studies demonstrated that MHY773 interacted with active site of tyrosinase. Moreover, a melanin quantification assay demonstrated that MHY773 attenuates α-melanocyte-stimulating hormone (α-MSH) and 3-isobutyl-1-methylxanthine (IBMX)-induced melanin contents in B16F10 melanoma cells. Taken together, these data suggest that MHY773 suppressed the melanin production via the inhibition of tyrosinase activity. MHY773 is a promising for the development of effective pharmacological and cosmetic agents for skin-whitening.
Binding mode and affinity of MHY773 with tyrosinase was investigated by enzyme kinetics and docking simulations. MHY773 showed potent tyrosinase inhibitory activity.
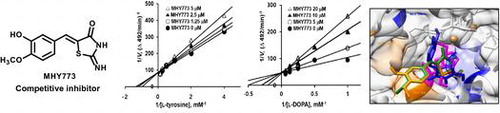
Melanin is synthesized by melanocytes distributed in the innermost layer of the epidermis [Citation1]. Melanin synthesis is stimulated by a variety of molecules and conditions, such as α-melanocyte stimulating hormone (α-MSH), cyclic AMP (cAMP) elevating agents, including forskolin, glycyrrhizin, and 3-isobutyl-1-methylxanthine (IBMX), UVB radiation, and the placental total lipid fraction [Citation2–4]. Melanin, can act as a photoprotective agent; however, excessive accumulation can cause hyperpigmentation, which reduces the quality of food, and can cause age spots and melisma spots on the skin [Citation5,6]. Most skin-whitening products target melanin production, and tyrosinase inhibitors are the most commonly used agents [Citation7,8]. When the skin is exposed to UV light, melanogenesis increase substantially via tyrosinase activation. Tyrosinase (EC1.14.18.1), a multifunctional copper-containing enzyme in animals and plants, catalyzes the first 2 steps of melanin biosynthesis from l-tyrosine i.e. the hydroxylation of l-tyrosine by monophenolase action and oxidation of l-dihydroxyphenylalanine (l-DOPA) to o-dopaquinone [Citation7]. These quinones are highly reactive compounds that can polymerize spontaneously to form melanins, which determine the color of mammalian skin and hair [Citation9]. Various dermatological disorders, such as age spots, freckles, melasma, and ephelide, are caused by the excessive accumulation of epidermal pigmentation [Citation10]. Hence, effective tyrosinase inhibitors have been reported to potentially improve food quality and to ameliorate skin hyperpigmentation in medical applications [Citation11].
Extensive research has been devoted to the search for effective and harmless tyrosinase inhibitors, and a number of naturally occurring and synthetic tyrosinase inhibitors have been reported [Citation9,11,12]. However, these inhibitors are rarely used in practice owing to their weak individual activities or safety concerns. Undoubtedly, it is still necessary to find and develop new tyrosinase inhibitors with better activity and minimal side effects. Potential active inhibitors, such as kojic acid and arbutin, have not yet been demonstrated to be clinically efficient inhibitors [Citation13,14]. Hydroquinone, a widely used skin-lightening agent, is still controversial with respect to its biosafety owing to its cytotoxic and mitogenic properties [Citation15]. Hence, there is still a considerable demand for novel tyrosinase inhibitors that are safer and effective.
In this study, considering the important roles of the β-phenyl-α,β-unsaturated carbonyl skeleton for tyrosinase inhibition [Citation16,17], twelve 2-iminothiazolidin-4-one derivatives (1a – 1l) were synthesized (Figure ), and their inhibitory activity against tyrosinase was evaluated. In addition, we examined the melanin contents in B16F10 melanoma cells and performed enzyme kinetics and in silico molecular modeling studies with mushroom tyrosinase.
Materials and methods
Chemicals and instrumentation
Mushroom tyrosinase (EC 1.14.18.1), α-MSH, IBMX, l-tyrosine, l-dihydroxyphenylalanine (l-DOPA), dimethyl sulfoxide (DMSO), and kojic acid were purchased from Sigma-Aldrich (St. Louis, MO, USA.). Dulbecco’s modified Eagle’s medium (DMEM), fetal bovine serum (FBS), streptomycin, and amphotericin were purchased from Gibco Life Technologies Inc. (Carlsbad, CA, U.S.A.). Thin-layer chromatography (TLC) and silica gel 60 (mesh 230–400) was carried out on silica gel F254-precoated plates from Merck Millipore (Darmstadt, Germany). All anhydrous solvents were distilled over CaH2 or Na/benzophenone prior to use. All melting points were determined in an open capillary on a Stuart capillary melting point apparatus (Staffordshire, UK) and are uncorrected. NMR spectra were recorded using Varian Unity INOVA 400 (400 MHz for 1H, 100 MHz for 13C) and Varian Unity AS 500 (500 MHz for 1H) instruments. Chemical shift values (δ) are reported with reference to the respective residual solvent or deuterated peaks (δH 2.50 and δC 39.51 for DMSO). Low-resolution mass spectrometry data were obtained on a using an Expression CMS mass spectrometer (Advion, Ithaca, NY, USA).
General procedure for the synthesis of (Z)-5-(substituted benzylidene)-2-iminothiazolidin-4-one analogues (1a – 1l)
The desired compounds, (Z)-5-(substituted benzylidene)-2-iminothiazolidin-4-one analogues (1a – 1l) (Figure ), were prepared by Knoevenagel condensation. With one exception, refluxing a solution of appropriate benzaldehydes and pseudothiohydantoin in acetic acid in the presence of NaOAc produced (Z)-5-benzylidene-2-iminothiazolidin-4-ones as a single stereoisomer in yields of 41.4–94.1%. A Knoevenagel condensation between 2,4-dimethoxybenzaldehyde and pseudothiohydantoin under the same conditions gave a mixture of (E)- and (Z)-5-(2,4-dimethoxybenzylidene)-2-iminothiazolidin-4-ones. These compounds could not be easily separated by conventional silica gel column chromatography. Milder reaction conditions capable of accomplishing the Knoevenagel condensation were needed to synthesize only (Z)-stereoisomer. Interestingly, heating a solution of 2,4-dimethoxybenzaldehyde and pseudothiohydantoin in ethanol:H2O (1:1) in the presence of 1.0 equiv. of piperidine as a base catalyst at 80 °C afforded the corresponding (Z)-stereoisomer (1l) as a sole product. A suspension of an appropriate benzaldehyde (300 mg, 1.53–2.46 mmol), pseudothiohydantoin (1.1 eq.), and sodium acetate (3.0 eq.) in acetic acid (1 mL/1 mmol of benzaldehyde) was refluxed for 3–7 h. The reaction mixture was cooled and water was added. The resulting precipitates were filtered, and washed with water and, if necessary, a small amount of methylene chloride or ethyl acetate, to produce (Z)-5-(substituted benzylidene)-2-iminothiazolidin-4-one products (1a – 1k) in 41.4–94.1% yields. The resulting precipitates were filtered, and washed with water, ethyl acetate and methylene chloride to give (Z)-5-(2,4-dimethoxybenzylidene)-2-iminothiazolidin-4-one (1l) as a single isomer in a yield of 21.0%. The structures of all final products were confirmed by 1H and 13C NMR spectroscopy and mass spectroscopy.
(Z)-5-(4-Hydroxybenzylidene)-2-iminothiazolidin-4-one (1a, MHY762)
Orange colored solid; reaction time, 3 h; yield, 61.8%; melting point, >300 °C; 1H-NMR (500 MHz, DMSO-d6) δ 10.10 (s, 1H, OH), 9.29 (br s, 1H, NH), 9.04 (s, 1H, NH), 7.49 (s, 1H, vinylic H), 7.40 (d, 2H, J = 9.0 Hz, 2′-H, 6′-H), 6.88 (d, 2H, J = 8.5 Hz, 3′-H, 5′-H); 13C-NMR (100 MHz, DMSO-d6) δ 181.4 (C-4), 176.1 (C-2), 159.6 (C-4′), 132.1 (C-2′, C-6′), 130.2 (benzylic C), 126.0 (C-5), 125.5 (C-1′), 116.8 (C-3′, C-5′); LRMS (ESI-) m/z 219 (M-H)-.
(Z)-5-(3,4-Dihydroxybenzylidene)-2-iminothiazolidin-4-one (1b, MHY764)
Brown solid; reaction time, 6 h; yield, 62.0%; melting point,>300 °C; 1H-NMR (500 MHz, DMSO-d6) δ 9.60 (br s, 1H, OH), 9.33 (br s, 2H, OH, NH), 9.02 (s, 1H, NH), 7.40 (s, 1H, vinylic H), 6.96 (s, 1H, 2′-H), 6.89 (d, 1H, J = 8.5 Hz, 6′-H), 6.83 (d, 1H, J = 8.5 Hz, 5′-H); 13C-NMR (100 MHz, DMSO-d6) δ 181.4 (C-4), 176.2 (C-2), 148.3 (C-4′), 146.4 (C-3′), 130.6 (benzylic C), 126.0 (C-1′), 125.8 (C-5), 123.5 (C-6′), 116.8 (C-5′), 116.6 (C-2′); LRMS (ESI-) m/z 235 (M-H) -.
(Z)-5-(4-Hydroxy-3-methoxybenzylidene)-2-iminothiazolidin-4-one (1c, MHY768)
Orange colored solid; reaction time, 7 h; yield, 92.4%; melting point, 263.9–265.6 °C; 1H-NMR (400 MHz, DMSO-d6) δ 9.69 (s, 1H, OH), 9.27 (br s, 1H, NH), 9.00 (s, 1H, NH), 7.48 (s, 1H, vinylic H), 7.11 (d, 1H, J = 2.0 Hz, 2′-H), 6.99 (dd, 1H, J = 2.0, 8.4 Hz, 6′-H), 6.86 (d, 1H, J = 8.4 Hz, 5′-H), 3.78 (s, 3H, 3′-OCH3); 13C-NMR (100 MHz, DMSO-d6) δ 181.3 (C-4), 176.0 (C-2), 149.1 (C-3′), 148.6 (C-4′), 130.5 (benzylic C), 126.2 (C-5), 126.0 (C-1′), 123.9 (C-6′), 116.7 (C-5′), 114.1 (C-2′), 56.2 (3′-OCH3); LRMS (ESI-) m/z 249 (M-H) -.
(Z)-5-(3-Ethoxy-4-hydroxybenzylidene)-2-iminothiazolidin-4-one (1d, MHY770)
Orange colored solid; reaction time, 7 h; yield, 84.9%; melting point, 248.8–251.2 °C; 1H-NMR (500 MHz, DMSO-d6) δ 9.63 (s, 1H, OH), 9.28 (br s, 1H, NH), 9.01 (s, 1H, NH), 7.49 (s, 1H, vinylic H), 7.11 (s, 1H, 2′-H), 7.02 (d, 1H, J = 8.5 Hz, 6′-H), 6.89 (s, 1H, J = 8.0 Hz, 5′-H), 4.06 (q, 2H, J = 7.0 Hz, 3′-OCH2), 1.35 (t, 3H, J = 7.0 Hz, CH3); 13C-NMR (100 MHz, DMSO-d6) δ 181.3 (C-4), 176.0 (C-2), 149.4 (C-3′), 147.7 (C-4′), 130.5 (benzylic C), 126.1 (C-1′), 126.0 (C-5), 124.1 (C-6′), 116.8 (C-5′), 115.1 (C-2′), 64.5 (3′-OCH2), 15.3 (CH3); LRMS (ESI-) m/z 263 (M-H) -.
(Z)-5-(3-Hydroxy-4-methoxybenzylidene)-2-iminothiazolidin-4-one (1e, MHY773)
Brown solid; reaction time, 7 h; yield, 78.9%; melting point, 282.8–285.7 °C; 1H-NMR (500 MHz, DMSO-d6) δ 9.38 (s, 1H, OH), 9.30 (br s, 1H, NH), 9.06 (s, 1H, NH), 7.44 (s, 1H, vinylic H), 7.04 (dd, 1H, J = 1.0, 8.5 Hz, 6′-H), 7.01 (d, 1H, J = 8.5 Hz, 2′-H), 6.99 (d, 1H, J = 1.0 Hz, 5′-H), 3.81 (s, 3H, 4′-OCH3); 13C-NMR (100 MHz, DMSO-d6) δ 181.3 (C-4), 176.2 (C-2), 149.9 (C-4′), 147.5 (C-3′), 130.1 (benzylic C), 127.4 (C-5), 126.9 (C-1′), 123.1 (C-6), 116.1 (C-2), 113.0 (C-5), 56.3 (4′-OCH3); LRMS (ESI-) m/z 249 (M-H) -.
(Z)-2-Imino-5-(4-methoxybenzylidene) thiazolidin-4-one (1f, MHY775)
Yellow solid; reaction time, 3 h; yield, 50.7%; melting point, 285.9–288.5 °C; 1H-NMR (500 MHz, DMSO-d6) δ 9.34 (br s, 1H, NH), 9.09 (s, 1H, NH), 7.53 (d, 2H, J = 8.5 Hz, 2′-H, 6′-H), 7.51 (s, 1H, vinylic H), 7.07 (d, 2H, J = 9.0 Hz, 3′-H, 5′-H), 3.80 (s, 3H, 4′-OCH3); 13C-NMR (100 MHz, DMSO-d6) δ 181.3 (C-4), 176.1 (C-2), 160.9 (C-4′), 131.9 (C-2′, C-6′), 129.7 (benzylic C), 127.1 (C-5), 127.1 (C-1′), 115.4 (C-3′, C-5′), 56.1 (4′-OCH3); LRMS (ESI-) m/z 233 (M-H) -.
(Z)-5-(3,4-Dimethoxybenzylidene)-2-iminothiazolidin-4-one (1g, MHY777)
Dark yellow solid; reaction time, 6 h; yield, 82.4%; melting point, 274.2–276.3 °C; 1H NMR (400 MHz, DMSO-d6) δ 9.32 (br s, 1H, NH), 9.05 (s, 1H, NH), 7.52 (s, 1H, vinylic H), 7.14 (d, 1H, J = 2.0 Hz, 2′-H), 7.12 (dd, 1H, J = 2.0, 8.4 Hz, 6′-H), 7.06 (d, 1H, J = 8.4 Hz, 5′-H), 3.77 (s, 3H, OCH3), 3.77 (s, 3H, OCH3); 13C-NMR (100 MHz, DMSO-d6) δ 181.2 (C-4), 176.0 (C-2), 150.7 (C-3′), 149.5 (C-4′), 130.1 (benzylic C), 127.4 (C-1′), 127.3 (C-5), 123.5 (C-6′), 113.4 (C-5′), 112.7 (C-2′), 56.3 (3′-OCH3), 56.1 (4′-OCH3); LRMS (ESI-) m/z 263 (M-H) -.
(Z)-5-(3,5-Dihydroxybenzylidene)-2-iminothiazolidin-4-one (1h, MHY782)
Dark brown solid; reaction time, 4 h; yield, 41.4%; melting point,>300 °C; 1H-NMR (500 MHz, DMSO-d6) δ 9.58 (s, 2H, OH), 9.37 (br s, 1H, NH), 9.13 (s, 1H, NH), 7.35 (s, 1H, vinylic H), 6.42 (s, 2H, 2′-H, 6′-H), 6.27 (s, 1H, 4′-H); 13C-NMR (100 MHz, DMSO-d6) δ 181.1 (C-4), 176.4 (C-2), 159.5 (C-3′, C-5′), 136.3 (C-1′), 130.3 (benzylic C), 129.6 (C-5), 108.2 (C-2′, C-6′), 104.8 (C-4′); LRMS (ESI-) m/z 235 (M-H) -.
(Z)-5-(2-Hydroxybenzylidene)-2-iminothiazolidin-4-one (1i, MHY787)
Brown solid; reaction time, 7 h; yield, 69.3%; melting point, 210.1–211.5 °C; 1H-NMR (500 MHz, DMSO-d6) δ 8.13 (s, 1H, vinylic H), 8.02–7.09 (brs, 3H, OH, 2 × NH), 7.72 (d, 1H, J = 6.5 Hz, 6′-H), 7.57 (t, 1H, J = 7.5 Hz, 5′-H), 7.43 (d, 1H, J = 8.0 Hz, 3′-H), 7.32 (t, 1H, J = 7.0 Hz, 4′-H); 13C-NMR (100 MHz, DMSO-d6) δ 180.3 (C-4), 176.2 (C-2), 159.2 (C-2′), 152.5 (benzylic C), 137.8 (C-5), 132.2 (C-4′), 128.5 (C-6′), 125.6 (C-5′), 119.9 (C-1′), 116.8 (C-3′).
(Z)-5-(3,4,5-Trimethoxybenzylidene)-2-iminothiazolidin-4-one (1j, MHY790)
Orange colored solid; reaction time, 3 h; yield, 94.1%; melting point, 253.3–254.8 °C; 1H NMR (500 MHz, DMSO-d6) δ 9.40 (br s, 1H, NH), 9.11 (s, 1H, NH), 7.55 (s, 1H, vinylic H), 6.91 (s, 2 H, 2′-H, 6′-H), 3.82 (s, 6 H, 3′-OCH3, 5′-OCH3), 3.70 (s, 3 H, 4′-OCH3); 13C-NMR (100 MHz, DMSO-d6) δ 181.0 (C-4), 176.1 (C-2), 153.8 (C-3′, C-5′), 139.3 (C-4′), 130.3 (C-1′), 130.0 (benzylic C), 129.1 (C-5), 107.6 (C-2′, C-6′), 60.8 (4′-OCH3), 56.6 (3′-OCH3, 5′-OCH3); LRMS (ESI-) m/z 293 (M-H) -.
(Z)-5-(4-Hydroxy-3,5-dimethoxybenzylidene)-2-iminothiazolidin-4-one (1k, MHY792)
Dark orange colored solid; reaction time, 6 h; yield, 87.7%; melting point, 254.3–256.6 °C; 1H-NMR (400 MHz, DMSO-d6) δ 9.30 (br s, 1H, NH), 9.08 (s, 1H, OH), 9.01 (s, 1H, NH), 7.49 (s, 1H, vinylic H), 6.85 (s, 2H, 2′-H, 6′-H), 3.78 (s, 6H, 3′-OCH3, 5′-OCH3); 13C-NMR (100 MHz, DMSO-d6) δ 181.2 (C-4), 176.0 (C-2), 148.8 (C-3′, C-5′), 138.3 (C-4′), 130.8 (benzylic C), 126.5 (C-5), 124.9 (C-1′), 108.0 (C-2′, C-6′), 56.6 (3′-OCH3, 5′-OCH3); LRMS (ESI-) m/z 279 (M-H) -.
(Z)-5-(2,4-Dimethoxybenzylidene)-2-iminothiazolidin-4-one (1l, MHY801)
Yellow solid; reaction time, 7 h; yield, 21.0%; melting point, 249.1–250.7 °C; 1H-NMR (400 MHz, DMSO-d6) δ 9.13 (br s, 2H, NH), 7.75 (s, 1H, vinylic H), 7.32 (d, 1H, J = 8.4 Hz, 6′-H), 6.66 (dd, 1H, J = 2.0, 8.0 Hz, 5′-H), 6.62 (d, 1H, J = 2.0 Hz, 3′-H), 3.84 (s, 3H, 2-OCH3), 3.79 (s, 3H, 4-OCH3); 13C-NMR (100 MHz, DMSO-d6) δ 181.0 (C-4), 176.2 (C-2), 162.8 (C-4′), 160.0 (C-2′), 129.8 (benzylic C), 127.0 (C-5), 124.2 (C-6′), 116.0 (C-1′), 106.8 (C-5′), 99.3 (C-3′), 56.5 (2′-OCH3), 56.2 (4′-OCH3); LRMS (ESI-) m/z 263 (M-H) -.
Tyrosinase inhibitory activity assay
The inhibitory activities of twelve compounds with different concentration ranges (final concentration: 50 μM for screening; 0.5, 1, and 5 μM for inhibitory concentration) were evaluated against mushroom tyrosinase (1000 U) using a modified spectrophotometric method developed by No et al. and Kang et al. [Citation18,19]. The tyrosinase inhibition rate (%) was calculated as (1−Abssample / Abscontrol) × 100%. The IC50 values was determined by a regression analysis of a dose-response curve at which 50% target activity was lost.
Kinetic analysis of mushroom tyrosinase
To determine the kinetic mechanisms, two complimentary methods based on Lineweaver-Burk and Dixon plots were used [Citation20–22]. Using Lineweaver-Burk double reciprocal plots [a plot of 1/enzyme velocity (1 / V) vs. 1/substrate concentration (1 / [S])], the type of inhibition was determined using various concentrations of l-tyrosine (0.25, 0.5, 1, 2, 4 and 8 mM) and l-DOPA (1, 2, 4, 8 and 16 mM) as a substrate in the absence and presence of various concentrations of MHY773 (0–20 μM). The Dixon plot for the inhibition of tyrosinase was obtained in the presence of various concentrations of l-tyrosine (0.5, 1, 2 and 4 mM) and l-DOPA (1, 2, 4 and 8 mM). The concentrations of MHY773 were as follows: 0–20 μM. The enzymatic procedures consisted of the same, aforementioned tyrosinase assay methods. The inhibition constant (Ki) was determined using the Dixon plots.
In silico docking simulation of tyrosinase inhibition
To determine the possible molecular mechanism and the most active groups of MHY773 for the inhibition of tyrosinase, molecular docking simulations were performed using AutoDock4.2.6. X-ray crystallographic structures show quaternary ligand binding to aromatic residues in the active-site gorge of tyrosinase (PDB ID: 2Y9X) from the RCSB Protein Data Bank (http://www.rcsb.org/adb) [Citation23]. The 3D structure of three compounds were created using ChemDraw Ultra. Because the docking programs use a set of predefined 3D grids for the target protein and a systematic search technique, we selected the previously defined 3D grids for tyrosinase interactions with tropolone at the binding pocket [Citation24]. Afterward, docking simulations were performed between tyrosinase and three compounds or kojic acid. A pharmacophore is an ensemble of ligands required for interactions with a specific receptor. A pharmacophore model was generated using LigandScout 4.0. [Citation25]. Based on the atom types, the chemical features of tyrosinase were defined in terms of pharmacophore elements, such as hydrogen bond acceptors, hydrogen bond donors, positive ionizable areas, hydrophobic interactions, and aromatic rings.
Cell culture
Mouse melanoma B16F10 cells were obtained from the Korea Cell Line Bank, cultured in DMEM supplemented with 10% FBS and 1% streptomycin, and then incubated at 37 °C with 5% CO2.
Assessment of melanin contents
The cellular melanin content was determined as described previously [Citation26]. B16F10 cells were seeded in 6-well culture plates at 2.5 × 104 cells/well with 5% CO2 for 24 h. To determine the inhibitory effect of MHY773 on melanogenesis, fresh medium was replaced with medium containing MHY773 at 1, 5, and 10 μM, with kojic acid at 5 μM as a positive control, and 0.5 μM α-MSH and 200 μM IBMX for 72 h. Cells were washed twice with PBS and cells in each well were pooled for each test. After centrifugation, cell pellets were disrupted in 100 μL of 1N NaOH, and then incubated at 60 °C for 1 h and mixed to solubilize the melanin. Absorbance at 405 nm was compared with a standard curve for synthetic melanin. The production of melanin was calculated by normalizing the melanin values against the protein content (absorbance/μg protein). For this purpose, the protein content was determined using the BCA protein assay reagent (Thermo Scientific, Rockford, IL, U.S.A.).The results from three individual experiments are shown.
Statistical analysis
All data are presented as means ± S.E.M. Data were analyzed by one-way ANOVA followed by the Bonferroni post hoc test. A *p-value of < 0.05 was considered statistically significant.
Results and discussion
We investigated the inhibitory effects of the iminothiazolidine derivatives on the monophenolase activity of tyrosinase on the substrate l-tyrosine. The results for tyrosinase inhibition by the newly synthesized derivatives are summarized in Table . MHY773 (1e) exhibited the most potent inhibitory activity against tyrosinase with 92.93% inhibition at 50 μM, compared to 79.57% for the positive control kojic acid. Compounds 1a, 1b, and 1h exhibited moderate mushroom tyrosinase inhibitory activity. Compound 1i showed weak mushroom tyrosinase inhibitory activity. In contrast, 1c, 1d, 1f, 1g, 1j, 1k, and 1l were inactive at the concentrations tested. Based on the structure-activity relationship, compound 1e, bearing 2-substituents (R2-hydroxyl and R3-methoxy groups) on the phenyl ring, was a potent inhibitor (Table ). Notably, the structural modifications to compound 1c, in which the positions of the hydroxyl and methoxy substituents of 1e are transposed, showed no inhibitory activity. Additionally, the insertion of an ethoxy group on the phenyl ring (1d) resulted in a complete loss of inhibitory activity against tyrosinase. Compound 1i, with a hydroxyl substituent at R1 on the phenyl ring, also showed no activity. However, compound 1a bearing a hydroxyl substituent at R3 showed moderate inhibitory activity. Moreover, compound 1b with an additional hydroxyl substituent at R2 also exhibited moderate inhibitory activity. Interestingly, compound 1h containing a hydroxyl substituent at both R2 and R4 showed moderate inhibitory activity. Compound 1f with one methoxy group, compounds 1g and 1l bearing two methoxy groups as substituents, or compound 1j with three methoxy groups or two methoxy groups and a hydroxyl group as the substituent of phenyl ring (1k) showed no activity on tyrosinase. Our results suggest that the number and location of methoxy and hydroxyl groups on the phenyl ring are very closely related to the capacity of these compounds for tyrosinase inhibition.
Table 1. Substitution pattern of the substituted (Z)-5-(substituted benzylidene)-2-iminothiazolidin-4-one derivatives (1a–1l).![]()
The tyrosinase inhibitory activity (IC50) and Ki values are shown in Table . Among the tested compounds 1a – 1l, MHY773 (1e) was found to be most effective in inhibiting the first step of oxidation. To further determine the detailed tyrosinase activity of MHY773, we conducted two step enzyme reaction including l-tyrosine and l-DOPA as substrates. As observed in Table , the IC50 values of MHY773 were estimated to be 2.87 ± 0.19 μM and 8.06 ± 0.41 μM on the monophenolase and diphenolase, respectively. As observed in Table , MHY773 was more effective in inhibiting the first step of oxidation (l-tyrosine oxidation). To obtain insights into the mechanism by which the MHY773 inhibited tyrosinase, we performed kinetics analyses using Lineweaver-Burk and Dixon plots, as summarized in Table . According to Table and Figure (A) and (C), a graphical analysis of the Lineweaver-Burk plot for MHY773 against tyrosinase indicated competitive inhibition, suggesting that this compound can competitively bind to the substrate-binding site to inhibit enzyme activity. Moreover, we used Dixon plots to determine the Ki by obtaining secondary plots of the slope vs. the concentration of MHY773, with a Ki value of 2.24 and 2.85 μM, on the monophenolase and diphenolase, respectively (Table ; Figure (B) and (D)). As the Ki value represents the concentration needed to form an enzyme–inhibitor complex, a lower Ki value may indicate more effective inhibitors against tyrosinase in the development of preventive and therapeutic agents.
Table 2. Tyrosinase inhibitory activities and enzyme kinetic analysis of MHY773.
Figure 2. Lineweaver-Burk and Dixon plots of tyrosinase inhibition by MHY773. (A) Tyrosinase inhibition with l-tyrosine as a substrate at various concentrations of MHY773: 0 μM (filled circles), 1.25 μM (open circles), 2.5 μM (filled triangles) and 5 μM (open triangles). (B) Tyrosinase inhibition by MHY773 at various concentrations of l-tyrosine: 0.5 mM (filled circles), 1 mM (open circles), 2 mM (filled triangles) and 4 mM (open triangles). (C) Tyrosinase inhibition with l-DOPA as a substrate at various concentrations of MHY773: 0 μM (filled circles), 5 μM (open circles), 10 μM (filled triangles) and 20 μM (open triangles). (D) Tyrosinase inhibition by MHY773 at various concentrations of l-DOPA: 1 mM (filled circles), 2 mM (open circles), 4 mM (filled triangles) and 8 mM (open triangles).
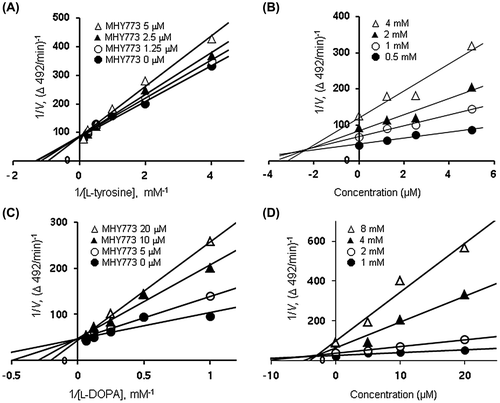
To understand the mechanism underlying the inhibition of tyrosinase by MHY773, the binding modes of MHY775 and MHY777 were compared to that of MHY773 were examined at the catalytic sites of tyrosinase. By docking simulations, we docked the 3D structure of mushroom tyrosinase respectively with MHY773, MHY775 and MHY777, supporting the hypothesis that three compounds interacts with amino acid residues in the active site (Figure (A)).
Figure 3. Computational structure prediction for mushroom tyrosinase and docking simulation with MHY773, MHY775, MHY777 and kojic acid (MHY773, MHY775, MHY777 and kojic acid are shown as blue, green, orange, and pink, respectively) (A). Predicted 3D structure of mushroom tyrosinase. Possible hydrogen bonding interactions between tyrosinase residues and three compounds (B) and kojic acid (C). Estimated binding free energy of the ligand-receptor complex of three compounds and kojic acid (D). We searched for H-binding interactions between tyrosinase and kojic acid or three compounds in the simulated docked structures; green lines indicate H-bonding interactions and yellow curves and lines indicate hydrophobic bonding interactions.
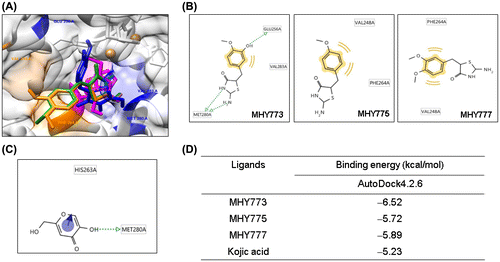
According to docking results, MHY773 formed H-bond interactions with two residues, MET280 and GLU256 (Figure (B)), together with one hydrophobic interaction with VAL283. Similarly, kojic acid also found to be interacted via a single H-bond with MET280 (Figure (C)). On the other hand, MHY775 and MHY777 did not form H-bond interaction, however, these compounds showed hydrophobic interactions, with VAL248 and PHE264, respectively (Figure (B)). Docking results confirm that MHY773 showed higher binding affinity (–6.52 kcal/mol) and formed both H- and hydrophobic interactions with active site of mushroom tyrosinase, while MHY775 and MHY777 oriented differently as well as did not form H-bond interactions and exhibited lower binding energy (–5.72 and –5.89 kcal/mol, respectively) than MHY773 (Figure (D)). Therefore, these might be the reasons why MHY773 showed higher mushroom tyrosinase inhibitory activity compared to MHY775 and MHY777.
Thus, docking simulations are in agreement and supporting experimental results that MHY773 was a potent mushroom tyrosinase inhibitor whereas, MHY775 and MHY777 were not active against tyrosinase. Furthermore, comparative structure activity analysis revealed that three compounds have iminothiazolidine functional group, while MHY773 has a hydroxyl group, whereas MHY777 contains a methoxy group but MHY775 has not any functional group at R2 position. So, these structural differences showed that substitutions greatly affected the inhibition activity, which is made clear by the fact that MHY773 is a potent tyrosinase inhibitor but MHY775 and MHY777 did not exhibit tyrosinase inhibitory activity. Recently, Wendt et al. (2016) [Citation27] also reported that presence or lack of functional groups and its substitutions greatly affected the inhibition activity against tyrosinase. From above results, functional groups at R2 position and amino acid residue in the active site regarding three iminothiazolidine compounds might play an important for tyrosinase inhibitory activity.
Skin-whitening agents that inhibit tyrosinase or other enzymes must first penetrate the melanocyte cell wall. Thus, MHY773 was further tested in B16F10 melanoma cells to confirm its activity in melanocytes. Prior to the investigation of the pharmacological activity of MHY773 on α-MSH and IBMX-induced melanogenesis-potential, the dose dependence of the cytotoxic effects of MHY773 and kojic acid on B16F10 cells was assessed using the EZ-Cytox assay. In our study, both MHY773 and kojic acid at concentrations up to 10 μM did not cause any cytotoxic effects during incubation for up to 72 h (data not shown). The effects of MHY773 on α-MSH and IBMX-stimulated melanin synthesis are summarized in Figure . To evaluate the inhibitory effect of MHY773 on melanin production, cells were pretreated with the indicated concentrations (1, 5, and 10 μM) of MHY773 for 2 h and then treated with both 0.5 μM α-MSH and 200 μM IBMX for 72 h. As shown in Figure , the melanin content in cells treated with MHY773 in the presence of α-MSH and IBMX decreased in a concentration-dependent manner, specifically, the content was 216.75% at 1 μM, 170.39% at 5 μM, and 153.72% at 10 μM, compared to that of the α-MSH and IBMX-induced control group (261.58%). The results of this study suggested that MHY773 can inhibit mushroom tyrosinase potential and also exhibits potent inhibitory effects on melanogenesis in B16F10 cells.
Figure 4. Effect of MHY773 on α-MSH and IBMX-induced cellular melanin contents in B16F10 melanoma cells. Cells were incubated in the presence of various concentrations of MHY773 (1, 5 and 10 μM) or kojic acid (5 μM) for 2 h, and then stimulated with 0.5 μM α-MSH and 200 μM IBMX for 72 h. Values are given as means ± S.E.M. of three independent experiments. ###p < 0.001 compared with the control group and **p < 0.01 and ***p < 0.001 compared with α-MSH and IBMX-induced cells.
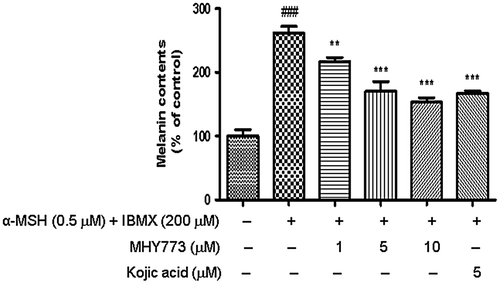
In this study, we describe the synthesis and tyrosinase inhibitory activity of (Z)-5-(substituted benzylidene)-2-iminothiazolidin-4-one derivatives (1a – 1l). Among them, MHY773 (1e) was approximately 5-fold more potent than kojic acid, which is often used as a positive control. In particular, structure-activity relationship analysis indicated the introduction of the β-phenyl-α,β-unsaturated carbonyl skeleton bearing R2-hydroxyl and R3-methoxy groups was crucial for the inhibition of tyrosinase activity. In addition, competitive inhibition and Ki values of 2.24 and 2.85 μM respective on the monophenolase and diphenolase were observed for MHY773 on tyrosinase. A docking simulation with MHY773 confirmed that MHY773 binds strongly to mushroom tyrosinase and also suggested that GLU256 and MET280 play a key role in the determination of the binding affinity of MHY773 via hydrogen bonding interactions. Thus, enzyme kinetics and docking simulation results suggested that MHY773 binds to the active site of tyrosinase, thereby inhibiting tyrosinase activity. MHY773 also inhibited melanin synthesis in B16F10 melanoma cells. However, the results of this study suggest that the mechanisms by which MHY773 inhibits tyrosinase and melanogenesis should be investigated in further studies.
Author contributions
H.J.J. and M.J.L. wrote the manuscript; H.J.J., Y.J.P., S.G.N., A.K.L., and K.M.M. performed the experiments and analyzed the data; Y.J.P., S.J.K., J.Y., S.U., and P.C. synthesized the compounds; H.J.J., E.K.L., E.J.B., Y.S.J., H.R.M., and H.Y.C. designed the study and edited the manuscript.
Disclosure statement
No potential conflict of interest was reported by the authors.
Funding
This work was supported by the Pusan National University [2017 Post-Doctoral Development Program]; National Research Foundation of Korea (NRF) funded by the Korean government (MSIP) [grant number 2009-0083538].
Acknowledgements
We thank Aging Tissue Bank for providing materials and research information.
References
- Spritz RA, Hearing VJ Jr., Hirschhorn K. Genetic disorders of pigmentation. In advanced human genetics. Springer: US; 1994.10.1007/978-1-4757-9062-7
- Wong G, Pawelek J. Melanocyte-stimulating hormone promotes activation of pre-existing tyrosinase molecules in Cloudman S91 melanoma cells. Nature. 1975;255:644–646.10.1038/255644a0
- Halaban R, Pomerantz SH, Marshall S, et al. Tyrosinase activity and abundance in cloudman melanoma cells. Arch. Biochem Biophys. 1984;230:383–387.10.1016/0003-9861(84)90121-8
- Hunt G, Todd C, Cresswell JE, et al. Alpha-melanocyte stimulating hormone and its analogue Nle4DPhe7 alpha-MSH affect morphology, tyrosinase activity and melanogenesis in cultured human melanocytes. J Cell Sci. 1994;107:205–211.
- Friedman M. Food browning and its prevention: an overview. J Agric Food Chem. 1996;44:631–653.10.1021/jf950394r
- Matsuura R, Ukeda H, Sawamura M. Tyrosinase inhibitory activity of citrus essential oils. J Agric Food Chem. 2006;54:2309–2313.10.1021/jf051682i
- Kim YJ, Uyama H. Tyrosinase inhibitors from natural and synthetic sources: structure, inhibition mechanism and perspective for the future. Cell Mol Life Sci. 2005;62:1707–1723.10.1007/s00018-005-5054-y
- Parvez S, Kang M, Chung HS, et al. Naturally occurring tyrosinase inhibitors: mechanism and applications in skin health, cosmetics and agriculture industries. Phytother Res. 2007;21:805–816.10.1002/(ISSN)1099-1573
- Solano F, Briganti S, Picardo M, et al. Hypopigmenting agents: an updated review on biological, chemical and clinical aspects. Pigment Cell Res. 2006;19:550–571.10.1111/pcr.2006.19.issue-6
- Chang TS. An updated review of tyrosinase inhibitors. Int J Mol Sci. 2009;10:2440–2475.10.3390/ijms10062440
- Seo SY, Sharma VK, Sharma N. Mushroom tyrosinase: recent prospects. J Agric Food Chem. 2003;51:2837–2853.10.1021/jf020826f
- Khan MT. Novel tyrosinase inhibitors from natural resources - their computational studies. Curr Med Chem. 2012;19:2262–2272.10.2174/092986712800229041
- Halder RM, Richards GM. Topical agents used in the management of hyperpigmentation. Skin Therapy Lett. 2004;9:1–3.
- Burnett CL, Bergfeld WF, Belsito DV, et al. Final report of the safety assessment of kojic acid as used in cosmetics. Int J Toxicol. 2010;29:244S–273S.10.1177/1091581810385956
- Draelos ZD. Skin lightening preparations and the hydroquinone controversy. Dermatol Ther. 2007;20:308–313.10.1111/dth.2007.20.issue-5
- Kim HR, Lee HJ, Choi YJ, et al. Benzylidene-linked thiohydantoin derivatives as inhibitors of tyrosinase and melanogenesis: importance of the β-phenyl-α, β-unsaturated carbonyl functionality. Med Chem Commun. 2014;5:1410–1417.10.1039/C4MD00171K
- Son S, Kim H, Yun HY, et al. (E)-2-Cyano-3-(substituted phenyl)acrylamide analogs as potent inhibitors of tyrosinase: A linear β-phenyl-α,β-unsaturated carbonyl scaffold. Bioorg Med Chem. 2015;23:7728–7734.10.1016/j.bmc.2015.11.015
- No JK, Soung DY, Kim YJ, et al. Inhibition of tyrosinase by green tea components. Life Sci. 1999;65:241–246.
- Kang KH, Lee B, Son S, et al. (Z)-2-(Benzo[d]thiazol-2-ylamino)-5-(substituted benzylidene)thiazol-4(5H)-one derivatives as novel tyrosinase inhibitors. Biol Pharm Bull. 2015;38:1227–1233.10.1248/bpb.b15-00300
- Lineweaver H, Burk D. The determination of enzyme dissociation constants. J Am Chem Soc. 1934;56:658–666.10.1021/ja01318a036
- Dixon M. The determination of enzyme inhibitor constants. Biochem J. 1953;55:170–171.10.1042/bj0550170
- Cornish-Bowden AC. A simple graphical method for determining the inhibition constants of mixed, uncompetitive and non-competitive inhibitors. Biochem J. 1974;137:143–144.10.1042/bj1370143
- Ismaya WT, Rozeboom HJ, Weijn A, et al. Crystal structure of Agaricus bisporus mushroom tyrosinase: identity of the tetramer subunits and interaction with tropolone. Biochemistry. 2011;50:5477–5486.10.1021/bi200395t
- Huey R, Morris GM, Olson AJ, et al. A semiempirical free energy force field with charge-based desolvation. J Comput Chem. 2007;28:1145–1152.10.1002/(ISSN)1096-987X
- Wolber G, Langer T. LigandScout: 3-D pharmacophores derived from protein-bound ligands and their use as virtual screening filters. J Chem Inf Model. 2005;45:160–169.10.1021/ci049885e
- Bilodeau ML, Greulich JD, Hullinger RL, et al. BMP-2 stimulates tyrosinase gene expression and melanogenesis in differentiated melanocytes. Pigment Cell Res. 2001;14:328–336.10.1034/j.1600-0749.2001.140504.x
- Wendt F, Näther C, Tuczek F. Tyrosinase and catechol oxidase activity of copper (I) complexes supported by imidazole-based ligands: structure-reactivity correlations. J Biol Inorg Chem. 2016;21:777–792.10.1007/s00775-016-1370-y