ABSTRACT
We evaluated the distribution of astaxanthin in rat brains after a single dose administration and after feeding 0.1% astaxanthin diet for 5 days. Astaxanthin was detected in the hippocampus and cerebral cortex 4 and 8 h after a single dose. Astaxanthin concentration in rat brains was higher after consumption of astaxanthin diet for 5 days than after a single dose.
Astaxanthin (3,3′-dihydroxy-β,β′-carotene-4,4′-dione), a natural lipophilic pigment of carotenoids, is biosynthesized by microalgae and bacteria and is widely distributed in marine organisms (). Human diets often contain astaxanthin-containing seafoods such as salmon, trout, shrimp, lobster, and crab. Astaxanthin derived from microalgae, especially Haematococcus pluvialis, is commercially available as a dietary supplement in many countries [Citation1]. Since oxidative stress and inflammatory response are involved in the development of various diseases, much attention has been paid to antioxidants as potential agents for preventing and alleviating the diseases. Astaxanthin is a strong antioxidant and may prevent oxidative stress-related diseases. Recently, the health benefits of astaxanthin supplements have been the subject of in vitro and clinical studies. The pharmacological effects of astaxanthin include antitumor, anticancer, antidiabetic, anti-atherosclerotic, and anti-inflammatory activities [Citation2–Citation4]. We recently found that dietary astaxanthin accumulates in the skin. By blocking the effects of UV radiation on filaggrin metabolism and desquamation in the epidermis and the extracellular matrix in the dermis, astaxanthin prevents photoaging [Citation5].
Several studies demonstrate that dietary astaxanthin affects brain functions [Citation6]. Oral administration of astaxanthin has been shown to be neuroprotective in various animal models. Yook et al. reported that astaxanthin dose-dependently increases spatial memory in adult mice by enhancing adult hippocampal neurogenesis and promoting the maturation of newborn neurons [Citation7]. Astaxanthin supplementation ameliorates memory impairment and neuronal oxidative stress in aluminum chloride-treated mice [Citation8] and mice undergoing repeated cerebral ischemia/reperfusion [Citation9]. In clinical trials, subjects displayed improvements in cognitive and learning performance after treatment with astaxanthin [Citation10,11]. The efficacy of astaxanthin can be attributed to its potential to prevent neuronal damage in oxidative stress-induced neurodegenerative disorders [Citation6].
Absorption of carotenoids from the diet occurs by passive diffusion into the intestinal epithelium and is facilitated by lysophosphatidylcholine produced by pancreatic phospholipase A2 [Citation12]. Recent studies suggest that the carotenoid uptake is partially mediated by scavenger receptor class B type 1 (SR-B1) [Citation13]. Xanthophylls, including astaxanthin, are oxygenated carotenoids. In dietary sources, xanthophylls are mostly present in an esterified form. In mammals, fatty acid esters of xanthophylls may be hydrolyzed in the small intestine. In fact, a free form of astaxanthin can be found in the plasma of rodents and humans after ingestion of the mono- and diester forms [Citation5,14]. Following absorption, carotenoids are incorporated into chylomicrons, transported to the liver via lymph and blood, and partly re-secreted with both high density lipoprotein (HDL) and low density lipoprotein (LDL) [Citation15].
Dietary astaxanthin is taken up by many tissues in rats [Citation16,17]. Research has found that astaxanthin can cross the blood-brain barrier [Citation6]. However, there is little evidence on the accumulation of dietary astaxanthin in the brain, especially distribution to different parts of the brain [Citation17]. In this study, we evaluated the distribution of astaxanthin in rat brains after single administration of astaxanthin and after repeated ingestion of a diet containing 0.1% astaxanthin.
Male Sprague-Dawley rats (9 weeks old) were purchased from SLC (Shizuoka, Japan). They were housed individually in standard polycarbonate cages at 24 ± 1 °C with a 12-h light/dark cycle. All animal care and experimental procedures of this study were performed in accordance to protocols approved by the Kyoto University animal committee (approval No. 28-37 and 28-90). The rats had free access to AIN-93G diet (Oriental Yeast, Tokyo, Japan) and water for a week. After the rats were acclimated, astaxanthin (equivalent to 100 mg/kg body weight of astaxanthin free form) was administered by gastrogavage (n = 4). A commercially available oil extracted from Haematococcus pluvialis (ASTOTS, FUJIFILM Co., Tokyo, Japan), which is composed of mainly triacylglycerol containing with 20% astaxanthin, was dissolved in soybean oil. After 4 and 8 h, blood was collected from the abdominal aorta, and the rats were sacrificed under isoflurane anesthesia. To avoid contamination with blood, the brain was carefully perfused with abundant cold saline via the heart before dissection. Following dissection, the brain was divided into the hippocampus, cerebral cortex, and remaining parts. The brain sections were immediately deep frozen in liquid nitrogen and then stored at −80 °C in a deep freezer until analysis.
The rats were acclimated for 1 week with free access to AIN-93G diet (Oriental Yeast, Tokyo, Japan) and water. The rats were divided into two groups (n = 6) after acclimatization. The control group was fed AIN-93G diet, and the experimental group was fed AIN-93G diet with 0.1% astaxanthin added (ASTOTS, equivalent to astaxanthin free form). After 5 days, blood was collected from the abdominal aorta, and the rats were sacrificed under isoflurane anesthesia. Brain samples were prepared and stored as described above. Total lipids were extracted from each brain part, as well as from plasma samples, by using chloroform and methanol [Citation18]. After evaporation of the collected chloroform phase, the residue was dissolved in an aliquot of methanol and then subjected to HPLC analysis for the quantification of astaxanthin [Citation12]. The concentration of astaxanthin was determined as all-trans- plus cis-isomers. Multiple comparisons were performed with Tukey’s test after one-way ANOVA (p < 0.05).
Astaxanthin was not detected in the brain and plasma of the rats before administration. After oral administration, all-trans-astaxanthin was only detectable in the hippocampus and cerebral cortex of the rat brain using HPLC analysis. Because astaxanthin was found in plasma after oral ingestion, we carefully perfused the brain with saline to avoid contamination with blood prior to analysis. Cis-isomers of astaxanthin were found about 20–30% in the plasma. Compared with plasma concentrations, the astaxanthin concentration in the brain was much lower. There were no differences in the brain and plasma astaxanthin concentrations between 4 and 8 h after its administration (). Eight hours after administration, the astaxanthin concentration in the cerebral cortex was significantly higher than that in the other part of brain.
Figure 2. Astaxanthin concentration of the brain (a) and plasma (b) in rats after oral administration of a single dose. Data are presented as means ± SEM (n = 4). Values with different letters are significantly different (p < 0.05).
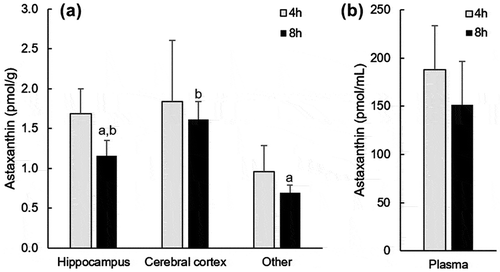
Feed consumption, feed conversion, and final body weight were unaffected after consumption of 0.1% astaxanthin diet for 5 days (data not shown). Astaxanthin was not detected in the brain and plasma of the control rats. Based on food consumption, the calculated intake of astaxanthin per day was ~70 mg/kg body weight. Astaxanthin was not detected in the brains of control rats. The concentration of astaxanthin in the brains and plasma of rats fed the astaxanthin diet for 5 days was ~10 times and ~2–3 times, respectively, higher than the concentrations after a single astaxanthin dose (). This shows that dietary astaxanthin accumulated in the brain, especially in the cerebral cortex. Since noticeable behavior and appearance changes were not observed in any rats fed astaxanthin, toxicity was unlikely due to accumulation of astaxanthin in the brain.
Table 1. Accumulation of astaxanthin in rat plasma and brain after 5 days of 0.1% astaxanthin diet.
Although astaxanthin can cross the blood-brain barrier, little is known about the accumulation of dietary astaxanthin in the brain [Citation16]. In this study, we found that dietary astaxanthin accumulated in the hippocampus and cerebral cortex of rat brains after single and repeated ingestion.
Astaxanthin is more potent than other natural antioxidants such as alpha-tocopherol and beta-carotene [Citation1]. Many studies support the use of astaxanthin to delay or ameliorate cognitive impairment associated with normal aging or to alleviate the pathophysiology of various neurodegenerative diseases. Dietary astaxanthin accumulation in the hippocampus may also exert neuroprotective effects, because the hippocampus is essential for learning and memory and plays a role in cognitive processes. In this study, astaxanthin concentration was higher in the cerebral cortex than in the other parts of the brain. The cerebral cortex is responsible for higher brain functions such as perception, voluntary movement, thought, reasoning, memory, and psychomotor function. For example, processing and psychomotor speed are related to the functions of the frontal and temporal lobe, respectively [Citation19–Citation21]. Thus, accumulation of dietary astaxanthin in the cerebral cortex may also affect the maintenance and improvement of cognitive functions.
Author contribution
Y.M., S.S. and T.S. designed the experiments. Y.M. and T.K. performed experiments. All authors discussed the data. Y.M. and T.S wrote the paper.
Disclosure statement
FUJIFILM Corporation funded this study and provided support for the author T.S. S.S. is a member of FUJIFILM Corporation, a sponsor and funder of this study. The other authors state no conflict of interest.
References
- Ambati RR, Phang SM, Ravi S, et al. Astaxanthin: sources, extraction, stability, biological activities and its commercial applications – a review. Mar Drugs. 2014;12:128–152.10.3390/md12010128
- Chew BP, Park JS, Wong MW, et al. A comparison of the anticancer activities of dietary beta-carotene, canthaxanthin and astaxanthin in mice in vivo. Anticancer Res. 1999;19:1849–1853.
- Pashkow FJ, Watumull DG, Campbell CL. Astaxanthin: a novel potential treatment for oxidative stress and inflammation in cardiovascular disease. Am J Cardiol. 2008;101:58D–68D.10.1016/j.amjcard.2008.02.010
- Kishimoto Y, Yoshida H, Kondo K. Potential anti-atherosclerotic properties of astaxanthin. Mar Drugs. 2016;14:35.10.3390/md14020035
- Komatsu T, Sasaki S, Manabe Y, et al. Preventive effect of dietary astaxanthin on UVA-induced skin photoaging in hairless mice. PLoS One. 2017;12:e0171178.10.1371/journal.pone.0171178
- Grimmig B, Kim SH, Nash K, et al. Neuroprotective mechanisms of astaxanthin: a potential therapeutic role in preserving cognitive function in age and neurodegeneration. GeroScience. 2017;39:19–32.10.1007/s11357-017-9958-x
- Yook JS, Okamoto M, Rakwal R, et al. Astaxanthin supplementation enhances adult hippocampal neurogenesis and spatial memory in mice. Mol Nutr Food Res. 2016;60:589–599.10.1002/mnfr.201500634
- Al-Amin MM, Reza HM, Saadi HM, et al. Astaxanthin ameliorates aluminum chloride-induced spatial memory impairment and neuronal oxidative stress in mice. Eur J Pharmacol. 2016;777:60–69.10.1016/j.ejphar.2016.02.062
- Xue Y, Qu Z, Fu J, et al. The protective effect of astaxanthin on learning and memory deficits and oxidative stress in a mouse model of repeated cerebral ischemia/reperfusion. Brain Res Bull. 2017;131:221–228.10.1016/j.brainresbull.2017.04.019
- Katagiri M, Satoh A, Tsuji S, et al. Effects of astaxanthin-rich Haematococcus pluvialis extract on cognitive function: a randomised, double-blind, placebo-controlled study. J Clin Biochem Nutr. 2012;51:102–107.10.3164/jcbn.D-11-00017
- Ito N, Saito H, Seki S, et al. Effects of composite supplement containing astaxanthin and sesamin on cognitive functions in people with mild cognitive impairment: a randomized, double-blind, placebo-controlled trial. J Alzheimers Dis. 2018;62:1767–1775.
- Sugawara T, Kushiro M, Zhang H, et al. Lysophosphatidylcholine enhances carotenoid uptake from mixed micelles by Caco-2 human intestinal cells. J Nutr. 2001;131:2921–2927.10.1093/jn/131.11.2921
- Kotake-Nara E, Nagao A. Absorption and metabolism of xanthophylls. Mar Drugs. 2011;9:1024–1037.10.3390/md9061024
- Miyazawa T, Nakagawa K, Kimura F, et al. Plasma carotenoid concentrations before and after supplementation with astaxanthin in middle-aged and senior subjects. Biosci Biotechnol Biochem. 2011;75:1856–1858.10.1271/bbb.110368
- Furr HC, Clark RM. Intestinal absorption and tissue distribution of carotenoids. J Nutr Biochem. 1997;8:364–377.10.1016/S0955-2863(97)00060-0
- Petri D, Lundebye AK. Tissue distribution of astaxanthin in rats following exposure to graded levels in the feed. Comp Biochem Physiol C. 2007;145:202–209.
- Choi HD, Kang HE, Yang SH, et al. Pharmacokinetics and first-pass metabolism of astaxanthin in rats. Br J Nutr. 2011;105:220–227.10.1017/S0007114510003454
- Bligh EG, Dyer WJ. A rapid method for total lipid extraction and purification. Can J Biochem Physiol. 1959;37:911–917.10.1139/y59-099
- Kochunov P, Coyle T, Lancaster J, et al. Processing speed is correlated with cerebral health markers in the frontal lobes as quantified by neuroimaging. NeuroImage. 2010;49:1190–1199.10.1016/j.neuroimage.2009.09.052
- Piazzini A, Turner K, Chifari R, et al. Attention and psychomotor speed decline in patients with temporal lobe epilepsy: A longitudinal study. Epilepsy Res. 2006;72:89–96.10.1016/j.eplepsyres.2006.04.004
- Sunga C, Jones JE, Jackson DC, et al. Age-accelerated psychomotor slowing in temporal lobe epilepsy. Epilepsy Res. 2013;103:231–236.10.1016/j.eplepsyres.2012.08.011