ABSTRACT
We previously reported that the dried peel powder of Citrus kawachiensis exerted anti-inflammatory effects in the brain in several animal models. Hyperglycemia induces inflammation and oxidative stress and causes massive damage in the brain; therefore, we herein examined the anti-inflammatory and other effects of the dried peel powder of C. kawachiensis in the streptozotocin-induced hyperglycemia mice model and in the type 2 diabetic db/db mice model. The C. kawachiensis administration inhibited microglial activation in the hippocampus in the streptozotocin-injected mice. Moreover, The C. kawachiensis treatment inhibited astroglial activation in the hippocampus and the hyperphosphorylation of tau at 231 of threonine and 396 of serine in hippocampal neurons, and also relieved the suppression of neurogenesis in the dentate gyrus of the hippocampus in the db/db mice. It was suggested that the dried peel powder of C. kawachiensis exerts anti-inflammatory and neuroprotective effects in the brain.
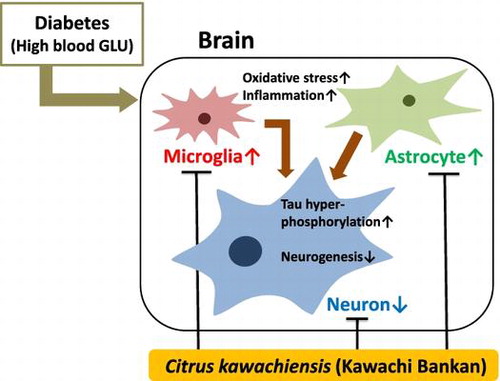
The dried peel powder of C. kawachiensis exerts neuroprotective and anti-inflammatory effects in the db/db mice brain.
Diabetes is accompanied by chronic hyperglycemia, the consequences of which include multiple pathophysiological processes, such as protein glycation, reactive oxygen species production, and inflammation [Citation1]. Chronic inflammation, characterized by elevated circulating levels of proinflammatory markers, including IL-1, appears to play a critical role in the pathogenesis of type 2 diabetes and its associated complications [Citation2]. Hyperglycemia induces inflammation and neuronal dysfunction not only in peripheral tissues, but also in the brain, and alters various conditions that are linked to a number of disorders [Citation3]. Diabetes was recently identified as a risk factor of Alzheimer’s disease (AD), which is a neurodegenerative disease with a prevalence that increases with aging [Citation4–6]. The AD brain is frequently associated with severe inflammation, oxidative stress, hyperphosphorylation of tau protein, beta-amyloid accumulation, neuronal dysfunction, and memory impairment [Citation7]. Studies on hyperglycemia use the well-established streptozotocin (STZ)-induced diabetes model, a glucosamine-nitrosourea compound. After uptake into insulin-secreting pancreatic beta cells by abundantly expressed GLUT-2 transporters, STZ induces cell death through deoxyribonucleic acid (DNA) methylation [Citation8], resulting in chronic hyperglycemia and hypoinsulinemia. Severe inflammation, oxidative stress, tau hyperphosphorylation, and neuronal dysfunction have been observed in the brain of the STZ-induced hyperglycemia model [Citation9,10]. There are many animal models of type 2 diabetes mellitus, including db/db mice, which have a mutation in the leptin receptor gene [Citation11]. Leptin is a mediator of the long-term regulation of the energy balance by suppressing food intake and, thus, inducing weight loss [Citation12], and a mutation or deficiency in its receptor causes obesity and hyperglycemia. Strong inflammatory responses, oxidative stress, tau hyperphosphorylation, and neuronal dysfunction have also been confirmed in the brain of db/db mice [Citation13,14].
We have demonstrated that the dried peel powder of Citrus kawachiensis, one of the citrus products of Ehime, Japan, exerted the suppression of microglial activation in the hippocampus in the brain of a lipopolysaccharide (LPS)-injected systemic inflammation animal model [Citation15]. It was known that microglial activation is responsible for inflammatory and oxidative stress reactions in the central nervous system. Its administration C. kawachiensis also attenuated hyper-tau phosphorylation and the inhibition of neurogenesis in the brain of an aged mice model [Citation16]. The dried peel powder of C. kawachiensis contains various biologically active substances including naringin (NGI; 44.02 ± 0.491 mg/g dried peel powder), narirutin (4.46 ± 0.0563 mg/g), auraptene (AUR; 4.07 ± 0.033 mg/g), and 3,5,6,7,8,3′,4′-heptamethoxyflavone (HMF; 0.27 ± 0.0039 mg/g) [Citation15,17]. We previously reported that AUR exerted anti-inflammatory and neuroprotective effects [Citation18–20], similar to HMF [Citation21–23].
In the present study, we examined that the dried peel powder of C. kawachiensis exerted anti-inflammatory and neuroprotective effects in the brain of the STZ-induced hyperglycemia mouse model and db/db mice.
Materials and methods
Sample preparation
The dried peel powder of C. kawachiensis was prepared as described in our previous study [Citation16], and the powder was used as the test sample.
Experiment schedule-Experiment 1
Nine-week-old male C57BL/6 mice were purchased from Japan SLC (Hamamatsu, Japan), and the animal experiment was performed under the approved protocol (#9-002; September 2, 2009) by the Animal Care and Use Committee of Matsuyama University. The environment of animal facility was the same as described in our previous study [Citation16].
In experiments, mice were randomly divided into the following three groups (n = 7 each): vehicle (saline) treatment and oral administration of distilled water group, named CON, STZ treatment and oral administration of distilled water group, named STZ, and STZ treatment and oral administration of the dried C. kawachiensis peel sample group, named STZ + CK. On day 1, saline or STZ (165 mg/kg; Wako, Osaka, Japan) was intraperitoneally administered depending on the groups. On day 8, blood glucose concentration was measured, and mice with the concentration over 300 mg/dL were used in further experiment. The dried peel of C. kawachiensis sample was suspended in distilled water, and the test sample-administered group received 1.2 g of dried peel/kg/day per orally once a day for seven days. On day 15, mice were transcardially perfused with heparinized ice-cold phosphate-buffered saline (PBS) and brains were removed and postfixed as described in our previous study [Citation22].
Experiment schedule-Experiment 2
Five-week-old male C57BLKS/J lar-+Leprdb/+Leprdb mice and C57BLKS/J lar- m+/m+ mice were purchased from Japan SLC, and the animal experiment was performed under the approved protocol (#16-013; April 1, 2017) by the Animal Care and Use Committee of Matsuyama University.
On week 10, mice were randomly divided into the following four groups (n = 12 each) and started experiment diet feeding: basic diet, F-1 (Table ; Funabashi Farm Co., Ltd., Funabashi, Japan),-fed m+/m + group, named m/m, F-1 diet-fed + Leprdb/+Leprdb group, named db/db, 0.5 wt% dried peel powder of C. kawachiensis mixed F-1 diet-fed +Leprdb/+Leprdb group, named CK-0.5%, and 1 wt% mixed diet-fed +Leprdb/+Leprdb group, named CK-1%. On week 24, mice were deeply anesthetized with diethyl ether, and approximately 1 mL of blood was collected by cardiac puncture from the right ventricle and heparinized. Mice were then rapidly perfused with heparinized ice-cold PBS through the left ventricle, after which their brains were removed and postfixed.
Table 1. Composition of nutritional factors and calorie in the 100 g of F-1 diet.
Blood glucose measurement
In experiment 1, blood glucose concentrations were measured using a Blood Glucose Monitoring System (Glucose Pilot; Iwai Chemicals Company, Tokyo, Japan) with a blood drop from the tail vein on day 8 and day 15. In experiment 2, on week 24, blood was collected by cardiac puncture from the right ventricle and heparinized, and plasma was obtained as the supernatant after centrifugation at 1200 × g at 4 °C for 15 min. Blood glucose concentrations were measured using the Glucose CII-test WAKO kit (Wako).
Immunofluorescence for confocal microscopy
The immunofluorescence was performed as described in our previous study [Citation16]. Frozen brains were sagittally sectioned (lateral 1.5 ~ 2.0 mm from midline) at a thickness of 30 μm using a cryostat (CM3050S; Leica Microsystems, Heidelberger, Germany). The sections were incubated with each primary antibody (Table ). Images were captured with a confocal fluorescence microscopy system (LSM510 for Experiment 1 and LSM800 for Experiment 2; Zeiss, Oberkochen, Germany). The location of the captured images and quantification is shown with a square in each figure. Fluorescence signals in the images were quantified using ImageJ software (NIH, Bethesda, MD) as described previously [Citation16,22]. Positive signals were quantified using “measure” or “analyze particles” tool in Image J software, and using the tool, positive signals were evaluated over a criteria size in the suitable area.
Table 2. Summary of antibodies used for immunofluorescence and immunohistochemistry.
Immunohistochemistry for optical microscopy
The immunohistochemistry was performed as described in our previous study [Citation16]. Thirty-micrometer-thick sagittal sections were incubated with Iba1 antibody (Table ) to stain microglia. The location of the captured images and quantification is shown with a square in each figure. Positive signals were quantified using “binary” and “analyze particle” tool in Image J software, and using the tool, positive signals were counted over a criteria size in the suitable area.
Statistical analysis
Data for individual groups are expressed as means ± SEM. Data were analyzed by an unpaired t-test between two groups and one-factor ANOVA followed by Dunnett’s Multiple Comparison Test among three groups (Prism 6; GraphPad Software, La Jolla, CA). A value of p < 0.05 was considered to be significant.
Results
Suppressive effects of C. kawachiensis on microglial activation in the STZ model
Blood glucose concentrations, on day 15, were significantly elevated in the STZ group (Figure ; ***p < 0.001); however, no change was observed in the STZ + CK group. Hyperglycemia induces inflammation and oxidative stress in the brain [Citation9,10], and it was reported that microglial activation is responsible for inflammatory and oxidative stress reactions in the central nervous system [Citation24]. The integrated densities of microglial cells immunostained with the Iba1 antibody in captured micrographs were quantified using Image J software, and positive signals (cell body and projections) were counted over a criteria size to exclude background noise. Iba1-positive signals significantly increased in the stratum lacunosum-moleculare in the hippocampus of the STZ group (Figure (A) and (B); *p < 0.05). However, this was significantly suppressed in the STZ + CK group (Figure (A)), and the expression of Iba1-positive signals significantly decreased (Figure (B); ###p < 0.001). The integrated densities of astroglial cells immunostained with the GFAP antibody were not significantly different in the hippocampus (Figure (C)).
Figure 1. Blood glucose concentrations in experiment 1. Values are means ± SEM. The symbol shows a significant difference between the following conditions: CON vs STZ (***p < 0.001).
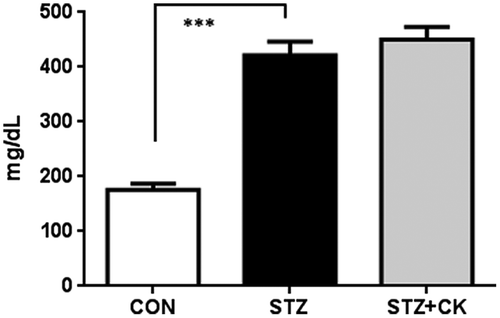
Figure 2. Effects of the dried peel powder of C. kawachiensis on Iba1 immunoreactivity in the hippocampus in experiment 1. (A) Sagittal sections were stained with the anti-Iba1 antibody. The scale bar shows 50 μm. (B) Quantitative analysis of Iba1-positive signals using ImageJ software. (C) Quantitative analysis of GFAP-positive signals using ImageJ software. (D) The location of the captured images and quantification is shown with a square in the figure. Values are means ± SEM. Symbols show significant differences between the following conditions: CON vs STZ (*p < 0.05), and STZ vs STZ + CK (### p < 0.001).
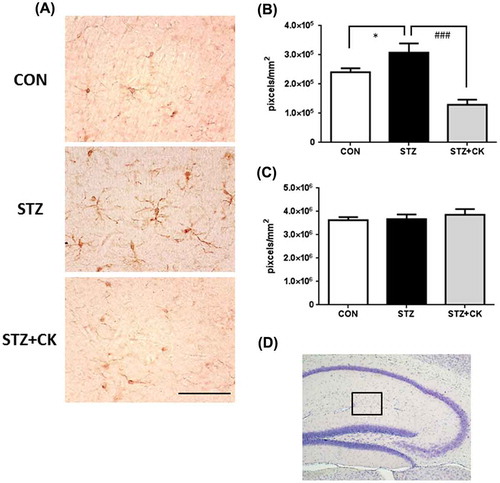
Effects of C. kawachiensis on tau hyper-phosphorylation in the STZ model
Tau is a microtubule-associated cytoskeletal protein in neurons. Neuropathological studies of AD staging strongly support the presence of a strong correlation the formation of neurofibrillary tangle (NFT) formation through its multiple phosphorylative and conformational changes [Citation25]. Tau hyperphosphorylation was previously shown to induce inflammation and oxidative stress [Citation26,27], and this was confirmed in the brains of STZ-injected mice [Citation28]. We evaluated tau phosphorylation levels at 231 of threonine (p-Thr231) and 396 of serine (p-Ser396) in the hippocampus in this experiment (Figures ). The phosphorylation levels of p-Thr231 in the hippocampus were significantly higher in the STZ group than in the CON group (Figure (A, B); *p < 0.05). The strong positive signals were confirmed in the CA3 pyramidal cell layer and in the stratum lacunosum-moleculare in the hippocampus. The integrated densities of the immune-positive signals were quantified using Image J software, and the positive signals only in the hippocampus were evaluated. However, suppressive effects on tau phosphorylation were significant in the STZ + CK group (Figure (A, B); #p < 0.05). Similar to p-Thr231, the phosphorylation levels of p-Ser396 in the hippocampus were significantly higher in the STZ group than in the CON group (Figure (A, B); **p < 0.01). The strong positive signals were confirmed in the hippocampal mossy fiber and in the stratum lacunosum-moleculare. The integrated densities of the immune-positive signals were quantified using Image J software, and the positive signals only in the hippocampus were evaluated. Furthermore, suppressive effects on tau phosphorylation were significant in the STZ + CK group (Figure (A, B); #p < 0.05).
Figure 3. Effects of the dried peel powder of C. kawachiensis on the expression of phosphorylated Tau at 231 of threonine in the hippocampus in experiment 1. (A) Sagittal sections were stained with the anti-pThr231 (red) and NeuN (green) antibodies. The scale bar shows 100 μm. (B) Quantitative analysis of pThr231-positive signals using ImageJ software. Values are means ± SEM. Symbols show significant differences between the following conditions: CON vs STZ (*p < 0.05), and STZ vs STZ + CK (# p < 0.05).
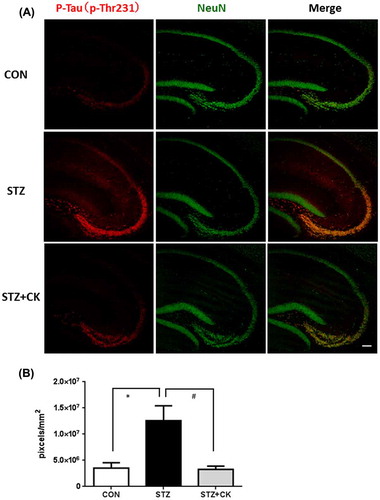
Figure 4. Effects of the dried peel powder of C. kawachiensis on the expression of phosphorylated Tau at 396 of serine in the hippocampus in experiment 1. (A) Sagittal sections were stained with the anti-pSer396 (red) and NeuN (green) antibodies. The scale bar shows 100 μm. (B) Quantitative analysis of pSer396-positive signals using ImageJ software. Values are means ± SEM. Symbols show significant differences between the following conditions: CON vs STZ (**p < 0.01), and STZ vs STZ + CK (# p < 0.05).
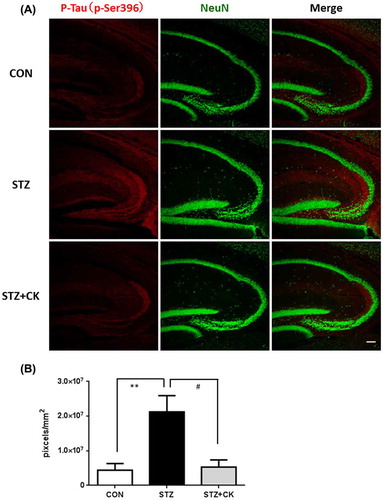
Enhancement of neurogenesis by C. kawachiensis in the hippocampus in the STZ model
The subgranular zone (SGZ) of the hippocampal dentate gyrus (DG) is one of the areas in which neurogenesis occurs in the brain [Citation29], and adult neurogenesis is known to play an important role in learning and memory. A previous study reported that a treatment with STZ suppressed neurogenesis in the DG [Citation30]. We demonstrated that the treatment with C. kawachiensis stimulated neurogenesis in the DG using DCX, a marker for immature neurons, and Figure shows the immunoreactivity of DCX in the SGZ. DCX-positive cells (green) were rarely recognized in the STZ group, but markedly increased in number in the STZ + CK group (Figure (A)). We evaluated the intensity of DCX-positive neurons (cell body and projections) in the SGZ, and the results obtained showed significantly suppressed expression in the STZ group (Figure (B); ***p < 0.001). However, the amelioration of this suppression was noted in the STZ + CK group (Figure (B); #p < 0.05).
Figure 5. Effects of the dried peel powder of C. kawachiensis on doublecortin immunoreactivity in the hippocampus in experiment 1. (A) Sagittal sections were stained with the anti-DCX antibody. The location of the high magnification image within the white square was indicated with the arrow. The thick white arrowheads indicate representative DCX-positive cells in the SGZ of DG. The scale bar shows 50 μm. (B) Intensity levels of DCX-positive signals in the hippocampus. (C) The location of the captured images is shown with a square in the figure. Values are means ± SEM. Symbols show significant differences between the following conditions: CON vs STZ (***p < 0.001), and STZ vs STZ + CK (# p < 0.05).
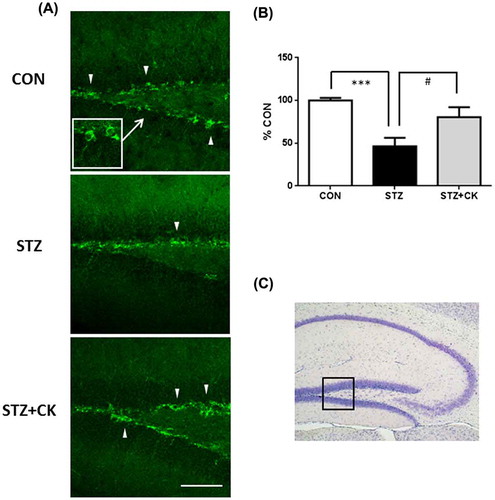
Suppressive effects of C. kawachiensis on astroglial activation in db/db mice
Blood glucose concentrations was significantly higher in db/db mice than in m/m mice (Figure ; ***p < 0.001); however, no significant change was observed in any of the CK-treated groups. Astroglial activation has been reported in the brain of db/db mice [Citation31], and inflammatory and oxidative stress reactions are known to activate astrocytes [Citation32]. The integrated densities of activated astrocytes were immunostained with the GFAP antibody in captured micrographs were quantified using Image J software, and positive signals (cell body and projections) were counted over a criteria size to exclude background noise. GFAP-positive signals significantly increased in number in the stratum lacunosum-moleculare and DG in the hippocampus in the db/db group (Figure (A, B); **p < 0.01). However, this increase was markedly suppressed in the CK-0.5% and CK-1% groups (Figure (A)), and the expression of GFAP-positive signals also significantly decreased (Figure (B); #p < 0.05, ##p < 0.01). The integrated densities of microglial cells immunostained with the Iba1 antibody were not significantly different in the hippocampus (Figure (C)).
Figure 6. Blood glucose concentrations in experiment 2. Values are means ± SEM. The symbol shows a significant difference between the following conditions: m/m vs db/db (***p < 0.001).
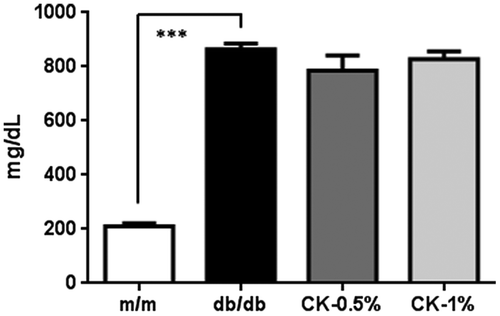
Figure 7. Effects of the dried peel powder of C. kawachiensis on GFAP immunoreactivity in the hippocampus in experiment 2. (A) Sagittal sections were stained with the anti-GFAP antibody. The scale bar shows 100 μm. (B) Quantitative analysis of GFAP-positive signals using ImageJ software. (C) Quantitative analysis of Iba1-positive signals using ImageJ software. (D) The location of the captured images and quantification is shown with a square in the figure. Values are means ± SEM. Symbols show significant differences between the following conditions: m/m vs db/db (**p < 0.01), and db/db vs CK-0.5% or CK-1% (# p < 0.05, ## p < 0.01).
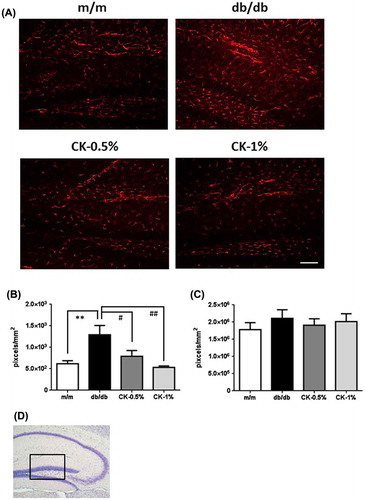
Effects of C. kawachiensis on tau hyper-phosphorylation in db/db mice
Tau hyperphosphorylation was confirmed in the brains of db/db mice [Citation38] and STZ-treated animals. We evaluated tau phosphorylation levels at 231 of threonine (p-Thr231) and 396 of serine (p-Ser396) in the hippocampus in this experiment (Figures ). The phosphorylation levels of p-Thr231 in the hippocampus were slightly higher in the db/db group than in the m/m group (Figure (A, B); p = 0.097). The strong positive signals were confirmed in the CA3 pyramidal cell layer and in the stratum lacunosum-moleculare in the hippocampus. The integrated densities of the immune-positive signals were quantified using Image J software, and the positive signals only in the CA3 pyramidal cell layer were evaluated. However, suppressive effects on tau phosphorylation were significant in the CK-0.5% and CK-1% groups (Figure (A, B); ##p < 0.01, ###p < 0.001). Similar to p-Thr231, the phosphorylation levels of p-Ser396 in the hippocampus were significantly higher in the db/db group than in the m/m group (Figure (A, B); ***p < 0.001). The strong positive signals were confirmed in the hippocampal mossy fiber and in the stratum lacunosum-moleculare. The integrated densities of the immune-positive signals were quantified using Image J software, and the positive signals only in hippocampal mossy fiber were evaluated. Furthermore, suppressive effects on tau phosphorylation were significant in the CK-1% group (Figure (A, B); ##p < 0.01).
Figure 8. Effects of the dried peel powder of C. kawachiensis on the expression of phosphorylated Tau at 231 of threonine in the hippocampus in experiment 2. (A) Sagittal sections were stained with the anti-pThr231 (green) and NeuN (red) antibodies. White arrowheads indicate the CA3 pyramidal cell layer. The scale bar shows 100 μm. (B) Quantitative analysis of pThr231-positive signals in the pyramidal cell layer using ImageJ software. Values are means ± SEM. Symbols show significant differences between the following conditions: db/db vs CK-0.5% or CK-1% (## p < 0.01, ### p < 0.001).
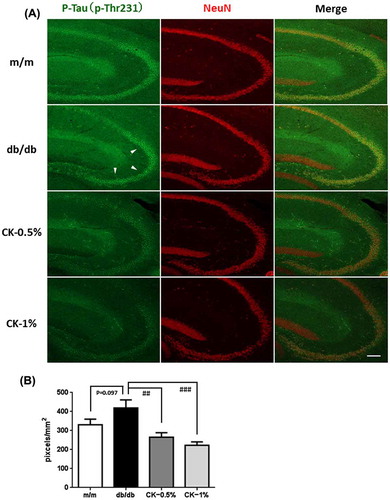
Figure 9. Effects of the dried peel powder of C. kawachiensis on the expression of phosphorylated Tau at 396 of serine in the hippocampus in experiment 2. (A) Sagittal sections were stained with the anti-pSer396 (green) and NeuN (red) antibodies. White arrowheads indicate hippocampal mossy fiber. The scale bar shows 100 μm. (B) Quantitative analysis of pSer396-positive signals in hippocampal mossy fiber using ImageJ software. Values are means ± SEM. Symbols show significant differences between the following conditions: m/m vs db/db (***p < 0.001), and db/db vs CK-1% (## p < 0.01).
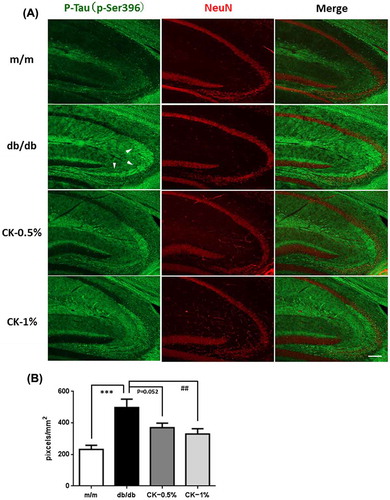
Enhancement of neurogenesis by C. kawachiensis in the hippocampus of db/db mice
A previous study reported that DCX-positive neurogenesis in the hippocampal DG was limited in a rat model of type 2 diabetes [Citation33] or a high-fat diet-fed mouse model [Citation34]. We demonstrated that treatments with C. kawachiensis stimulated neurogenesis in the DG using DCX, and Figure shows the immunoreactivity of DCX in the SGZ. DCX-positive cells (green) were rarely recognized in the db/db group, but markedly increased in number in the CK-0.5% and CK-1% groups (Figure (A)). We evaluated the intensity of DCX-positive neurons (cell body and projections) in the SGZ, and the results obtained showed significantly suppressed expression in the db/db group (Figure (B); ***p < 0.001); however, the amelioration of this suppression was observed in the CK-0.5% and CK-1% groups (Figure (B); ##p < 0.01, ###p < 0.001).
Figure 10. Effects of the dried peel powder of C. kawachiensis on doublecortin immunoreactivity in the hippocampus in experiment 2. (A) Sagittal sections were stained with the anti-DCX antibody. The location of the high magnification image within the white square was indicated with the arrow. The thick white arrowheads indicate representative DCX-positive cells in the SGZ of DG. The scale bar shows 100 μm. (B) Intensity levels of DCX-positive signals in the hippocampus. (C) The location of the captured images is shown with a square in the figure. Values are means ± SEM. Symbols show significant differences between the following conditions: m/m vs db/db (***p < 0.001), and db/db vs CK-0.5% or CK-1% (## p < 0.01, ### p < 0.001).
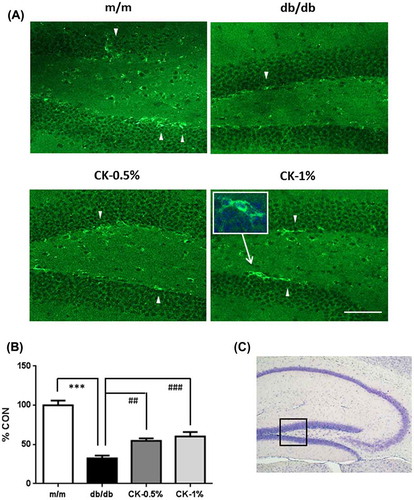
Discussion
Diabetes is accompanied by hyperglycemia, and induces inflammation and oxidative stress in central nervous tissue [Citation1]. These physiological changes result in neuronal dysfunction in the brain; diabetes is currently regarded as one of the risk factors for dementia, such as AD and vascular dementia [Citation4–6]. Previous studies implicated inflammation and oxidative stress in the tau hyperphosphorylation of neurons in the brain [Citation26,27]; therefore, in order to clarify the influence of C. kawachiensis on anti-inflammatory and neuroprotective effects, we performed each experiment using two hyperglycemia models.
STZ-treated mice (Experiment 1) and db/db mice (Experiment 2) both showed significantly elevated blood glucose concentrations, whereas no effect was noted in the C. kawachiensis-treated group. The peel of C. kawachiensis contains higher concentrations of AUR and NGI than other citrus fruits [Citation17]. Previous studies reported that the administration of 100 mg/kg of NGI or 0.2% of AUR resulted in suppressive effects on blood glucose concentrations in STZ-treated rats or high-fat diet-induced obese mice, respectively [Citation35,36]. These findings suggest that NGI and AUR reduce blood glucose concentrations. However, the treatment with the peel of C. kawachiensis did not reduce blood glucose concentrations in the present study because 1.2 g/kg/day of the peel of C. kawachiensis or a 1% peel-containing diet included lower doses of NGI and AUR than these studies.
Microglial and astrocyte activation, which are related to immune response cells in the brain, has been confirmed in hyperglycemia models [Citation13,31]. A treatment with the peel of C. kawachiensis suppressed microglial and astrocyte activation in these experiments, and we previously demonstrated that the activation of these cells was inhibited in the LPS-injected systemic inflammation model and SAMP8 mouse aging model using treatments with the peel of C. kawachiensis [Citation15,16]. Microglial activation was observed in the STZ-treated model, but not in db/db mice; however, astroglial activation occurred in db/db mice, but not in those treated with STZ. Microglia are activated earlier than astrocytes in a manner that is dependent on disease conditions [Citation43]; therefore, we considered that microglial activation was occurred in the early phase of the STZ treatment (on day 15; seven days after the STZ treatment), while astrocyte activation was observed in db/db mice (on week 24) in our two experiments.
Tau phosphorylation in neurons are important for maintaining microtubule function, is regulated by kinases and phosphatases balance; however, enhancement of inflammation and oxidative stress induce its hyperphosphorylation, and this is observed in the hyperglycemic brain [Citation26,27]. Tau hyperphosphorylation induces microtubule dysfunction, leading to the formation of NFT, which are often observed in the AD brain [Citation37]. Therefore, strategies to suppress tau hyperphosphorylation under AD and diabetes-related dementia conditions are important. There are several strong phosphorylation sites in tau, such as Thr231 and Ser396, in hyperglycemic animal brains [Citation28,38], and inflammation and oxidative stress may induce a kinase and phosphatase imbalance [Citation39]. We focused on phosphorylation sites at Thr231 and Ser396 in the present study, and C. kawachiensis treatment exerted suppressive effects on tau phosphorylation in the hippocampus in both STZ-treated and db/db mice. Neurogenesis in the SGZ of the DG in the hippocampus is of particular importance for hippocampal-dependent memory function [Citation40,41], and is suppressed by a number of conditions, including depression, AD, and aging. For these reasons, stimulate neurogenesis may contribute to neuronal and brain functional activation. Hyperglycemia was known to suppress neurogenesis [Citation33,34], and staining for neurogenesis marker DCX in the SGZ revealed that the C. kawachiensis treatment enhanced its expression in experiments 1 and 2 in the present study.
Extensive research has been conducted on hyperglycemia animal models using food materials and ingredients. Polyphenol-rich oriental plums were shown to ameliorate cognitive decline and reduce the expression of amyloid-beta protein, tau hyperphosphorylation, and oxidative stress in diabetic rats [Citation42]. Moreover, cortical and hippocampal atrophy was reduced in db/db mice, and tau hyperphosphorylation and microglia expression were reduced after a mango leaf extract treatment [Citation13]. NGI, a citrus flavonoid, ameliorated cognitive deficits via oxidative stress, proinflammatory factors, and the PPARγ signaling pathway in the STZ-injected rat model [Citation35]. When C. kawachiensis was administered to STZ-injected mice, it (1) diminished microglial activation in the hippocampus; (2) relieved tau phosphorylation; and (3) enhanced neurogenesis in the SGZ of the DG. In addition, when C. kawachiensis was administered to db/db mice, it (1) suppressed astroglial activation in the hippocampus; (2) decreased tau phosphorylation; and 3) enhanced neurogenesis in the SGZ of the DG. Thousands of ingredients are contained in the dried peel powder of C. kawachiensis, and we only have identified a few bioactive compounds, including NGI, AUR, and HMF; therefore, other active compounds still need to be discovered. However, we think NGI, AUR, and HMF, at least in part, contributed to the effects of C. kawachiensis. It was suggested that the dried peel powder of C. kawachiensis shows anti-inflammatory and neuroprotective effects against hyperglycemia-induced changes in the brain, and has potential as a novel neuroprotective agent obtained from food materials.
Author contributions
S. Okuyama designed and performed the research, and wrote the manuscript; W. Shinoka, K. Nakamura, M. Kotani, A. Sawamoto, K. Sugawara, and M. Sudo performed the research; M. Nakajima and Y. Furukawa gave research advice.
Disclosure statement
No potential conflict of interest was reported by the authors.
Acknowledgments
This work was supported by The Tojuro Iijima Foundation for Food Science and Technology, and JSPS KAKENHI grant number 26750058.
References
- Maher P , Dargusch R , Ehren JL , et al . Fisetin lowers methylglyoxal dependent protein glycation and limits the complications of diabetes. PLoS One. 2011;6:e21226.10.1371/journal.pone.0021226
- O’Connor JC , Satpathy A , Hartman ME , et al . IL-1beta-mediated innate immunity is amplified in the db/db mouse model of type 2 diabetes. J Immunol. 2005;174:4991–4997.10.4049/jimmunol.174.8.4991
- Dinel AL , André C , Aubert A , et al . Cognitive and emotional alterations are related to hippocampal inflammation in a mouse model of metabolic syndrome. PLoS One. 2011;6:e24325.10.1371/journal.pone.0024325
- Kim B , Backus C , Oh S , et al . Hyperglycemia-induced tau cleavage in vitro and in vivo: a possible link between diabetes and Alzheimer’s disease. J Alzheimer’s Dis. 2013;34:727–739.
- Kimura N . Diabetes mellitus induces Alzheimer’s disease pathology: histopathological evidence from animal models. Int J Mol Sci. 2016;17:503.10.3390/ijms17040503
- Bosco D , Fava A , Plastino M , et al . Possible implications of insulin resistance and glucose metabolism in Alzheimer’s disease pathogenesis. J Cell Mol Med. 2011;15:1807–1821.10.1111/jcmm.2011.15.issue-9
- McKee AC , Carreras I , Hossain L , et al . Ibuprofen reduces Aβ, hyperphosphorylated tau and memory deficits in Alzheimer mice. Brain Res. 2008;1207:225–236.10.1016/j.brainres.2008.01.095
- Ho N , Balu DT , Hilario MR , et al . Depressive phenotypes evoked by experimental diabetes are reversed by insulin. Physiol Behav. 2012;105:702–708.10.1016/j.physbeh.2011.09.003
- Cai Z , Yan Y , Wang Y . Minocycline alleviates beta-amyloid protein and tau pathology via restraining neuroinflammation induced by diabetic metabolic disorder. Clin Interv Aging. 2013;8:1089–1095.10.2147/CIA
- Sharma R , Buras E , Terashima T , et al . Hyperglycemia induces oxidative stress and impairs axonal transport rates in mice. PLoS One. 2010;5:e13463.10.1371/journal.pone.0013463
- Chen H , Charlat O , Tartaglia LA , et al . Evidence that the diabetes gene encodes the leptin receptor: identification of a mutation in the leptin receptor gene in db/db Mice. Cell. 1996;84:491–495.10.1016/S0092-8674(00)81294-5
- Klok MD , Jakobsdottir S , Drent ML . The role of leptin and ghrelin in the regulation of food intake and body weight in humans: a review. Obes Rev. 2007;8:21–34.10.1111/obr.2007.8.issue-1
- Infante-Garcia C , Jose Ramos-Rodriguez J , Marin-Zambrana Y , et al . Mango leaf extract improves central pathology and cognitive impairment in a type 2 diabetes mouse model. Brain Pathol. 2017;27:499–507.10.1111/bpa.2017.27.issue-4
- Jung GY , Won SB , Kim J , et al . Betaine Alleviates Hypertriglycemia and Tau Hyperphosphorylation in db/db Mice. Toxicol Res. 2013;29:7–14.10.5487/TR.2013.29.1.007
- Okuyama S , Yamamoto K , Mori H , et al . Auraptene in the Peels of Citrus kawachiensis (Kawachi Bankan) Ameliorates Lipopolysaccharide-Induced Inflammation in the Mouse Brain. Evid Based Complement Alternat Med. 2014;2014:408503.
- Okuyama S , Kotani Y , Yamamoto K , et al. The peel of Citrus kawachiensis (kawachi bankan) ameliorates microglial activation, tau hyper-phosphorylation, and suppression of neurogenesis in the hippocampus of senescence-accelerated mice. Biosci Biotechnol Biochem. 2018;82:869–878.
- Amakura Y , Yoshimura M , Ouchi K , et al . Characterization of constituents in the peel of citrus kawachiensis (Kawachibankan). Biosci Biotechnol Biochem. 2013;77:1977–1980.10.1271/bbb.130324
- Okuyama S , Minami S , Shimada N , et al . Anti-inflammatory and neuroprotective effects of auraptene, a citrus coumarin, following cerebral global ischemia in mice. Eur J Pharmacol. 2013;699:118–123.10.1016/j.ejphar.2012.11.043
- Okuyama S , Morita M , Kaji M , et al . Auraptene acts as an anti-inflammatory agent in the mouse brain. Molecules. 2015;20:20230–20239.10.3390/molecules201119691
- Okuyama S , Semba T , Toyoda N , et al . Auraptene and other prenyloxyphenylpropanoids suppress microglial activation and dopaminergic neuronal cell death in a lipopolysaccharide-induced model of Parkinson’s Disease. Int J Mol Sci. 2016;17:E1716.10.3390/ijms17101716
- Okuyama S , Shimada N , Kaji M , et al . Heptamethoxyflavone, a citrus flavonoid, enhances brain-derived neurotrophic factor production and neurogenesis in the hippocampus following cerebral global ischemia in mice. Neurosci Lett. 2012;528:190–195.10.1016/j.neulet.2012.08.079
- Okuyama S , Morita M , Miyoshi K , et al . 3,5,6,7,8,3′,4′-Heptamethoxyflavone, a citrus flavonoid, on protection against memory impairment and neuronal cell death in a global cerebral ischemia mouse model. Neurochem Int. 2014;70:30–38.10.1016/j.neuint.2014.03.008
- Okuyama S , Miyoshi K , Tsumura Y , et al . 3,5,6,7,8,3′,4′-heptamethoxyflavone, a citrus polymethoxylated flavone, attenuates inflammation in the mouse hippocampus. Brain Sci. 2015;5:118–129.10.3390/brainsci5020118
- Liu B , Hong JS . Role of microglia in inflammation-mediated neurodegenerative diseases: mechanisms and strategies for therapeutic intervention. J Pharmacol Exp Ther. 2003;304:1–7.10.1124/jpet.102.035048
- Augustinack JC , Schneider A , Mandelkow EM , et al . Specific tau phosphorylation sites correlate with severity of neuronal cytopathology in Alzheimer’s disease. Acta Neuropathol. 2002;103:26–35.10.1007/s004010100423
- Barron M , Gartlon J , Dawson LA , et al . A state of delirium: Deciphering the effect of inflammation on tau pathology in Alzheimer’s disease. Exp Gerontol. 2017;94:103–107.10.1016/j.exger.2016.12.006
- Wu Z , Nakanishi H . Connection between periodontitis and Alzheimer’s disease: possible roles of microglia and leptomeningeal cells. J Pharmacol Sci. 2014;126:8–13.10.1254/jphs.14R11CP
- Clodfelder-Miller BJ , Zmijewska AA , Johnson GV , et al . Tau is hyperphosphorylated at multiple sites in mouse brain in vivo after streptozotocin-induced insulin deficiency. Diabetes. 2006;55:3320–3325.10.2337/db06-0485
- Aimone JB , Li Y , Lee SW , et al . Regulation and function of adult neurogenesis: from genes to cognition. Physiol Rev. 2014;94:991–1026.10.1152/physrev.00004.2014
- Guo J , Yu C , Li H , et al . Impaired neural stem/progenitor cell proliferation in streptozotocin-induced and spontaneous diabetic mice. Neurosci Res. 2010;68:329–336.10.1016/j.neures.2010.08.012
- Zheng Y , Yang Y , Dong B , et al . Metabonomic profiles delineate potential role of glutamate-glutamine cycle in db/db mice with diabetes-associated cognitive decline. Mol Brain. 2016;9:40.10.1186/s13041-016-0223-5
- Wilms H , Sievers J , Rickert U , et al . Dimethylfumarate inhibits microglial and astrocytic inflammation by suppressing the synthesis of nitric oxide, IL-1β, TNF-α and IL-6 in an in vitro model of brain inflammation. J Neuroinflammation. 2010;7:30.10.1186/1742-2094-7-30
- Yi SS , Hwang IK , Choi JW , et al . Effects of hypothyroidism on cell proliferation and neuroblasts in the hippocampal dentate gyrus in a rat model of type 2 diabetes. Anat Cell Biol. 2010;43:185–193.10.5115/acb.2010.43.3.185
- Hamilton A , Patterson S , Porter D , et al . Novel GLP-1 mimetics developed to treat type 2 diabetes promote progenitor cell proliferation in the brain. J Neurosci Res. 2011;89:481–489.10.1002/jnr.v89.4
- Qi Z , Xu Y , Liang Z , et al . Naringin ameliorates cognitive deficits via oxidative stress, proinflammatory factors and the PPARγ signaling pathway in a type 2 diabetic rat model. Mol Med Rep. 2015;12:7093–7101.10.3892/mmr.2015.4232
- Takahashi N , Senda M , Lin S , et al . Auraptene regulates gene expression involved in lipid metabolism through PPARα activation in diabetic obese mice. Mol Nutr Food Res. 2011;55:1791–1797.10.1002/mnfr.v55.12
- Medeiros R , Baglietto-Vargas D , LaFerla FM . The role of tau in Alzheimer’s disease and related disorders. CNS Neurosci Ther. 2011;17:514–524.10.1111/cns.2011.17.issue-5
- Kim B , Backus C , Oh S , et al . Increased tau phosphorylation and cleavage in mouse models of type 1 and type 2 diabetes. Endocrinology. 2009;150:5294–5301.10.1210/en.2009-0695
- Ballatore C , Lee VM , Trojanowski JQ . Tau-mediated neurodegeneration in Alzheimer’s disease and related disorders. Nat Rev Neurosci. 2007;8:663–672.10.1038/nrn2194
- Winocur G , Wojtowicz JM , Sekeres M , et al . Inhibition of neurogenesis interferes with hippocampus-dependent memory function. Hippocampus. 2006;16:296–304.10.1002/(ISSN)1098-1063
- Shors TJ , Miesegaes G , Beylin A , et al . Neurogenesis in the adult is involved in the formation of trace memories. Nature. 2001;410:372–376.10.1038/35066584
- Lee KT , Chen YH , Lin CI , et al . Consumption of Oriental Plums Improved the Cognitive Performance and Modulated the Cerebral Neurodegeneration-Related Protein Expressions in Rats with Nicotinamide/Streptozotocin-Induced Diabetes. Food Nutr Sci. 2013;4:1145–1154.10.4236/fns.2013.411148
- Webster KM , Sun M , Crack P , et al . Inflammation in epileptogenesis after traumatic brain injury. J Neuroinflammation. 2017;14:10.10.1186/s12974-016-0786-1