ABSTRACT
Advanced glycation end-products (AGEs) elicit inflammatory responses via the receptor for AGEs (RAGE) and participate in the pathogenesis of diabetic complications. An earlier study showed that 3-hydroxypyridinium (3-HP), a common moiety of toxic AGEs such as glyceraldehyde-derived pyridinium (GLAP) and GA-pyridine, is essential for the interaction with RAGE. However, the physiological significance of 3-HP recognition by RAGE remains unclear. We hypothesized that pyridinoline (Pyr), a collagen crosslink containing the 3-HP moiety, could have agonist activity with RAGE. To test this hypothesis, we purified Pyr from bovine achilles tendons and examined its cytotoxicity to rat neuronal PC12 cells. Pyr elicited toxicity to PC12 cells in a concentration-dependent manner, and this effect was attenuated in the presence of either the anti-RAGE antibody or the soluble form of RAGE. Moreover, surface plasmon resonance-based analysis showed specific binding of Pyr to RAGE. These data indicate that Pyr is an intrinsic ligand for RAGE.
Abbreviations: AGEs: advanced glycation end-products; RAGE: receptor for advanced glycation end-products; DAMPs: damage-associated molecular patterns; PRR: pattern recognition receptor; TLR: toll-like receptor; GLAP: glyceraldehyde-derived pyridinium; 3-HP: 3-hydroxypyridinium; Pyr: pyridinoline; HFBA: heptafluorobutyric acid; GST: glutathione S-transferase; SPR: surface plasmon resonance; ECM: extracellular matrix; EMT: epithelial to mesenchymal transition
GRAPHICAL ABSTRACT
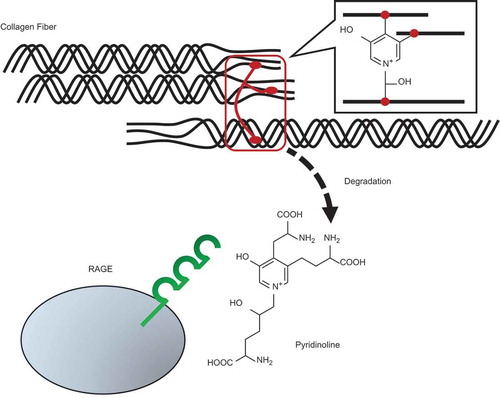
Pyridinoline, a collagen crosslink released during collagen degradation, acts as an intrinsic ligand for RAGE.
The Maillard reaction, also characterized as glycation, is a series of non-enzymatic reactions in which amino groups of proteins are modified by carbonyl groups of reducing sugars and their degradation products. In the advanced stage of the reaction, advanced glycation end-products (AGEs) are formed in lysine and arginine residues of proteins [Citation1]. AGEs accumulate under hyperglycemic conditions and elicit pro-inflammatory responses via interaction with the receptor for AGEs (RAGE) [Citation2,Citation3]. RAGE is a type-1 transmembrane receptor expressed in various types of cells, including macrophages, endothelial cells, and neuronal cells [Citation4]. The AGEs-RAGE axis is associated with the pathogenesis and development of multiple chronic diseases such as diabetic complications and neurodegenerative diseases [Citation5].
In addition to AGEs, damage-associated molecular patterns (DAMPs) such as high mobility group box 1 and S100/calgranulin proteins are also recognized by RAGE and evoke inflammatory responses [Citation6,Citation7]. DAMPs are structurally diverse molecules released by damaged host cells during infection [Citation8]. Thus, RAGE functions as a pattern recognition receptor (PRR), like toll-like receptors (TLRs), and participates in the homeostatic maintenance and host defense against infections. Under diabetic conditions, however, the excessive activation of RAGE by chronic exposure of AGEs causes multiple diabetic complications.
A growing body of evidence has indicated that AGEs formed from α-hydroxyaldehydes, such as glyceraldehyde and glycolaldehyde, are involved in the pathogenesis of chronic diseases [Citation9–Citation11]. Recently, we reported that glyceraldehyde-derived pyridinium (GLAP) [Citation12] and GA-pyridine [Citation13], major AGE structures derived respectively from glyceraldehyde and glycolaldehyde (()), are recognized by RAGE [Citation14]. We also showed that 3-hydroxypyridinium (3-HP), a common moiety in GLAP and GA-pyridine, is critical for the interaction with RAGE [Citation14]. Given that these AGEs accumulate in disorders such as diabetes and renal failure, and in aging, it is possible that under the physiological conditions RAGE recognizes unknown intrinsic ligands containing the 3-HP moiety. Characterization of a novel RAGE ligand would help to elucidate the detailed roles of RAGE and understanding of the mechanisms of RAGE-related diseases.
Figure 1. Structure and preparation of Pyr.
(A) Chemical structures of GLAP, GA-pyridine and Pyr. (B) Typical HPLC chromatograms of isolated Pyr monitored for fluorescence (Ex/Em, 295 nm/395 nm) and absorbance at 295 nm. The purified Pyr solution was injected onto an analytical C18 column at a flow rate of 0.8 mL/min using a HPLC system. (C) The UV-Vis spectrum of the purified Pyr analyzed by a photodiode array detector.
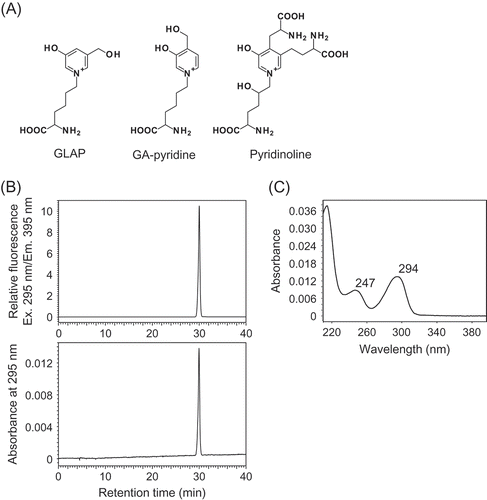
Fujimoto et al. have identified a 3-HP-containing structure, pyridinoline (also termed hydroxylysyl pyridinoline; Pyr), as a collagen crosslink (()) [Citation15]. Pyr is abundant in collagen of cartilages, bones and tendons, and contributes to the flexibility and strength of these tissues [Citation16]. Pyr is released when collagen is degraded during bone turnover, and it has been used as a biomarker of bone absorption in many clinical and experimental studies [Citation17–Citation19]. In contrast, despite the biological implications of Pyr release during collagen degradation or damage, no possible physiological activities or receptors of Pyr molecule have been reported.
In the present study, we aimed to elucidate the biological significance of 3-HP recognition by RAGE, and hypothesized that Pyr is a novel intrinsic ligand for RAGE. We revealed the RAGE-mediated effects of Pyr and direct binding between Pyr and RAGE. Our data demonstrate that Pyr acts on cells as a RAGE ligand.
Materials and methods
Chemicals
The chemicals used in this research were purchased from the following sources: Heptafluorobutyric acid (HFBA) from Sigma-Aldrich (St. Louis, MO, USA); Acetonitrile, trypan blue and Tween 20 from Fujifilm Wako Pure Chemical (Osaka, Japan); Dulbecco’s modified eagle medium (DMEM) from Nissui Pharmaceutical (Tokyo, Japan); Horse serum (HS) and penicillin/streptomycin solution from Thermo Fisher Scientific (Waltham, MA, USA); Fetal bovine serum (FBS) from Biowest (Nuaillé, France); Anti-RAGE monoclonal antibody (MAB11451) and mouse IgG2B isotype control (MAB004) from R&D Systems (Minneapolis, MN, USA); 1-Ethyl-3-(3-dimethylaminopropyl) carbodiimide hydrochloride (EDC), N-hydroxysuccinimide (NHS) and ethanolamine-HCl from GE Healthcare (Buckinghamshire, UK); Desmosine from Elastin Products Company (Owensville, MO, USA). Other chemicals used in this study were of an analytical grade. Water was used after purification by Simplicity UV (Merck, Darmstadt, Germany).
Purification of Pyr
The Pyr used in this study was prepared from commercially available bovine achilles tendons (Sumiyoshi Co., Yamanashi, Japan). Tendons (100 g) were chopped and hydrolyzed in 300 mL of 6 M HCl at 110 °C for 24 h. The hydrolysate was filtered and evaporated, and the pellet was dissolved in water and neutralized with NaOH to pH 4.0. The solution was centrifuged at 9,000 × g for 10 min, and then the supernatant was loaded onto a FPC3500 column (Organo, Tokyo, Japan) equilibrated with water. The resin was washed with 5 column volumes of water and eluted with 5 column volumes of 0.5 M NaCl. The eluent was concentrated and re-dissolved in 0.1% HFBA, and then the sample solution was loaded onto a Sep-Pak C18 cartridge (Waters, Milford, MA, USA) equilibrated with 0.1% HFBA. The cartridge was washed and desalted with 0.1% HFBA, and then the Pyr fraction was eluted from the cartridge with 50% acetonitrile. The eluent was concentrated and put on a preparative ODS column (Inertsil ODS-4, 10 i.d. × 250 mm, GL Sciences, Tokyo, Japan) using a reversed phase-high performance liquid chromatography (HPLC) system. HPLC procedures were performed with a LC-20AT HPLC pump, a SPD-10A UV-Vis detector and a RF-20Axs fluorescence detector (Shimadzu, Kyoto, Japan). The sample was eluted with a linear gradient of 0.1% HFBA and 50% acetonitrile containing 0.1% HFBA (from 100:0 to 60:40 for 70 min) at a flow rate of 3.0 mL/min. The eluent was monitored for absorbance at 295 nm and for fluorescence (Ex/Em, 295 nm/395 nm). The peak containing Pyr was collected and freeze-dried, and this purified Pyr was dissolved in 0.1 M HCl as a stock solution (500 µM), based on the molar extinction coefficient of Pyr as reported previously [Citation20]. The stock solution was stored at below −80 °C. For cytotoxicity tests, the Pyr solution was freeze-dried and then re-dissolved in phosphate-buffered saline (PBS) at 500 µM.
The purity of isolated Pyr was assessed by HPLC analysis. Isolated Pyr was put on an analytical ODS column (Inertsil ODS-4, 4.0 i.d. × 250 mm, GL Sciences, Tokyo, Japan). The HPLC analysis was conducted with a 1525 HPLC pump, a 2996 photodiode array detector, a 2475 multi λ fluorescence detector and a 717 autosampler (Waters, Milford, MA, USA). The sample was separated with a linear gradient of 0.1% HFBA and 50% acetonitrile containing 0.1% HFBA (from 100:0 to 60:40 for 40 min) at a flow rate of 0.8 mL/min. The eluent was analyzed by the photodiode array detector and monitored for fluorescence (Ex/Em, 295 nm/395 nm). The compound was also confirmed by liquid chromatography-mass spectrometry (LC-MS) (data not shown).
Expression and purification of recombinant proteins
cDNA fragments of the human RAGE extracellular V domain (amino acids 23–116) and soluble RAGE (sRAGE, amino acids 23–322) were ligated into a pET21b(+) expression vector containing the glutathione S-transferase (GST) sequence, as described previously [Citation14]. The resulting constructs containing the N-terminal GST tag were designated as pET21b(+)/GST-V and pET21b(+)/GST-sRAGE. Expression and purification of recombinant proteins were carried out as described previously [Citation14].
Cell culture
Rat adrenal phaeochromocytoma PC12 cells were obtained from the Japanese Collection of Research Bioresources Cell Bank (Osaka, Japan). The PC12 cells were cultured with DMEM supplemented with 10% HS, 5% FBS, 10 U/mL penicillin and 10 µg/mL streptomycin in a humidified atmosphere containing 5% CO2 at 37 °C.
Cytotoxicity test
The PC12 cells were seeded at a density of 1 × 105 cells/well in a 96-well plate and cultured at 37 °C for 24 h. The cells were exposed to the medium containing each concentration of Pyr at 37 °C for 72 h, and then cell viability was assessed by the trypan blue exclusion test [Citation21]. To evaluate the effects of RAGE inhibition, cells were pretreated with either the anti-RAGE antibody or the isotype control IgG (50 µl/mL) at 37 °C for 1 h, and then cultured in medium containing 5 µM Pyr at 37 °C for 24 h. To examine the effects of the soluble form of RAGE as a ligand scavenger, recombinant GST-V (50 µg/mL) and 5 µM Pyr were co-incubated at room temperature for 30 min. The PC12 cells were exposed to this mixture at 37 °C for 72 h. The recombinant GST protein was used as a negative control.
Surface plasmon resonance (SPR) experiments
SPR analysis was performed with a Biacore X100 system (GE Healthcare, Buckinghamshire, UK). A CM5 sensor chip (GE Healthcare, Buckinghamshire, UK) was preactivated with EDC and NHS according to the manufacturer’s instructions. Purified GST-sRAGE was dissolved in 10 mM sodium acetate buffer (pH 4.5) at 100 µg/mL, and immobilized on the sensor chip to obtain about 15,000 resonance units (RU). The immobilized surface was blocked with ethanolamine-HCl. Each concentration of Pyr (3.8, 7.7, 15.3, 30.7 and 61.3 µM) or desmosine (0.0625, 0.125, 0.25, 0.5 and 1.0 mM) was dissolved in running buffer (20 mM PBS containing 0.05% Tween 20) and injected over the flow cells for 60 sec at a flow rate of 30 µL/min. After each injection, the surface was regenerated with 10 mM glycine-HCl (pH 2.0) for 60 sec. All SPR sensorgrams were obtained by subtracting the response of the reference flow cell. Kinetic data were obtained using a Biacore X100 Evaluation Software and 1:1 binding as a fitting model.
Statistics
Numeric data are expressed as the means ± SE. The statistical significance of differences was evaluated by Dunnett’s test using IBM SPSS statistics software. Differences with probability values less than 5% were considered to be significant.
Results
Purification of Pyr
Previous studies have indicated that under acidic conditions Pyr shows a maximal absorption at around 295 nm and emits a specific fluorescence (Ex/Em, 295 nm/395 nm) [Citation15]. A remarkable fluorescent peak was observed after solid extraction of the crude Pyr fraction using the Sep-Pak C18 cartridge on the preparative HPLC system. After the preparative HPLC procedures, highly purified Pyr was detected on the analytical HPLC system ()). The UV-Vis spectrum of the compound indicated a maximal absorption at 294 nm (()). LC-MS analysis showed that the protonated molecular ion mass of the compound was 429.199 (calcd. for C18H29N4O8, 429.198). The yield of the HFBA adduct of Pyr was about 10.1 mg per 100 g (fresh weight) of bovine achilles tendons.
RAGE-dependent toxicity of Pyr
We evaluated the toxic effects of Pyr on PC12 cells. As shown in , cell viability was significantly decreased by Pyr in a concentration-dependent manner (). We next examined the RAGE-mediated toxic effects of Pyr. The toxicity of Pyr was attenuated in the presence of the anti-RAGE antibody, while the isotype control antibody exhibited no effects on cell viability (()). The soluble form of RAGE has been shown to inhibit cellular signal transduction following the AGEs-RAGE interaction by trapping ligands [Citation22]. The results of the current study were consistent with this, showing that the toxic effect of Pyr was significantly suppressed by recombinant GST-V (GST-fused RAGE extracellular V domain), but not by the negative control recombinant GST ()).
Figure 2. Cytotoxicity of Pyr.
Viability of PC12 cells 72 h after treatment with different concentrations of Pyr. Cell viability was assessed by trypan blue exclusion test. Data are expressed as the means ± SE. * represents a significant difference compared with the control (p < 0.05, n = 3), evaluated by Dunnett’s test.
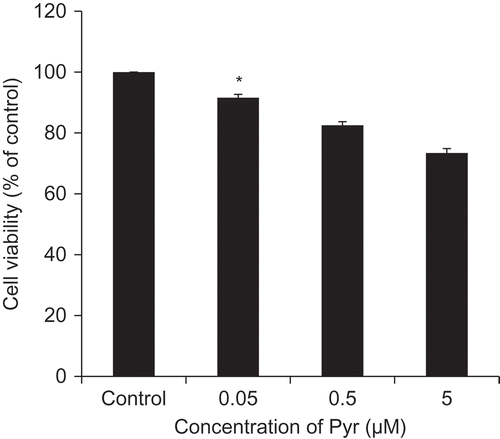
Figure 3. Pyr elicits cytotoxicity to PC12 cells via RAGE.
(a) Effects of the anti-RAGE antibody on the toxicity of Pyr. PC12 cells were treated with the anti-RAGE antibody or its isotype control IgG (50 µg/mL) for 1 h, and then exposed to 5 µM Pyr. Cell viability was assessed by trypan blue exclusion test after 24 h. (B) Effects of the recombinant soluble RAGE protein on the toxicity of Pyr. PC12 cells were exposed to a mixture of purified GST or GST-V (50 µg/mL) and Pyr (5 µM) that had been pre-incubated at room temperature for 30 min. Cell viability was assessed by trypan blue exclusion test after 72 h. Data are expressed as the means ± SE. * represents a significant difference compared with the control (p < 0.05, n = 3), evaluated by Dunnett’s test.
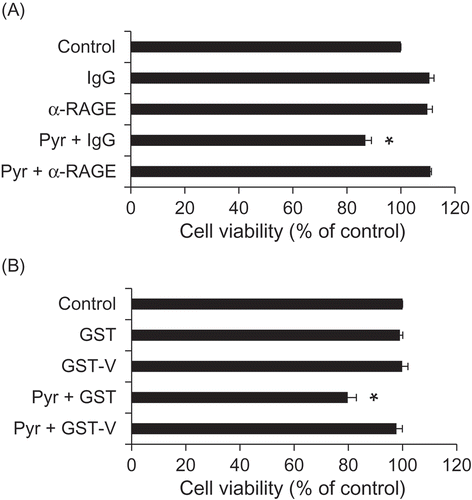
Pyr directly binds to RAGE
We analyzed the physical interaction between Pyr and RAGE using a Biacore system. A SPR assay showed concentration-dependent binding responses after different concentrations of Pyr were injected over the flow cells with purified GST-sRAGE immobilized on the surface of a CM5 sensor chip (
Figure 4. Pyr directly interacts with RAGE.
Binding responses between RAGE and different concentrations of either (A) Pyr or (B) desmosine. Purified GST-sRAGE was covalently immobilized on a CM5 sensor chip to obtain about 15,000 RU using a Biacore system. Each concentration of Pyr (3.8, 7.7, 15.3, 30.7 and 61.3 µM) or desmosine (0.0625, 0.125, 0.25, 0.5 and 1.0 mM) was injected over the flow cells for 60 sec at a flow rate of 30 µL/min. All SPR sensorgrams were compensated by subtracting the response of the reference flow cell.
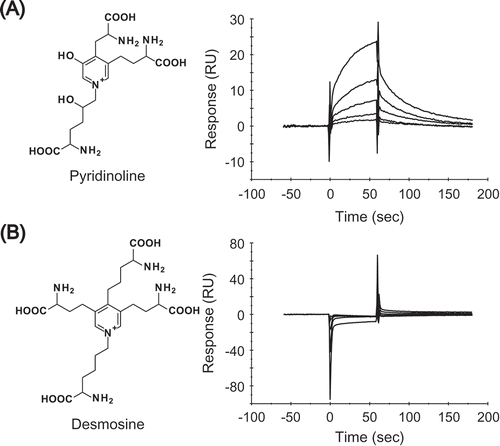
Discussion
We recently reported that RAGE elicits cell toxicity by binding to specific AGE structures as GLAP and GA-pyridine. We have also shown that 3-HP, a common moiety in GLAP and GA-pyridine, is critical for the interaction with RAGE [Citation14]. These findings imply the presence of intrinsic RAGE ligands containing the 3-HP moiety. In addition, other research has shown that the enzymatic post-translational modification of a collagen triple helix by lysyl oxidase results in the formation of 3-HP-containing crosslinks in collagen [Citation24]. Pyr is the most abundant crosslink in a wide variety of tissues [Citation16], but its physiological effects on cells and possible receptors are largely unknown.
In the current study, Pyr was isolated from a commercially available bovine achilles tendon by cation exchange column chromatography and preparative HPLC. Purified Pyr was detected on analytical HPLC monitoring fluorescence (Ex/Em, 295 nm/395 nm) and absorbance at 295 nm (
The AGEs-RAGE axis has been shown to accelerate cellular dysfunction following the generation of reactive oxygen species [Citation26]. We previously revealed that 3-HP derivatives including GLAP and GA-pyridine elicit toxicity in a rat neuronal PC12 cell line through interaction with RAGE [Citation14]. In the current study, Pyr decreased cell viability of PC12 cells in a concentration-dependent manner (
In addition, SPR-based analysis showed that Pyr bound directly to RAGE, but desmosine, a crosslink in elastin, did not interact with RAGE. These data are consistent with an earlier study showing that the 3-hydoxyl group of pyridinium is critical for RAGE ligand activity [Citation14]. The KD value of Pyr to RAGE was determined as 4.38 × 10−5 (M), indicating that Pyr interacts with RAGE at about 10-fold higher affinity than AGEs such as GLAP (KD = 5.21 × 10−4 (M)) and GA-pyridine (KD = 2.47 × 10−4 (M)) [Citation14]. An earlier study revealed that GLAP was detected in streptozotocin-induced diabetic rats but not in normal rats, indicating that GLAP accumulates only under hyperglycemic conditions [Citation27]. The physiological concentration of Pyr in serum has considered to be about 0.003 – 0.019 µM [Citation28,Citation29], whereas the concentration used in the cytotoxicity assays in PC12 cells were 0.05 – 5 µM. As shown in
PRRs, including TLRs and RAGE, evoke inflammatory processes by recognizing DAMPs that are mainly released from the inside of necrotic cells [Citation30]. Also, recent research has revealed that components of the extracellular matrix (ECM), such as hyaluronic acid and fibronectin, act as DAMPs [Citation31–Citation34]. However, the way in which PRRs sense ECM-related molecules has not been fully elucidated, nor have their physiological roles. Despite the fact that collagen is a major protein of ECM, no collagen-derived DAMPs have been reported, even though collagen damage has been shown to be involved in pathologic conditions. For instance, during bacterial invasion, host tissue collagen was degraded by bacterial proteinases, which typically leads to the pathogenesis of multiple disorders [Citation35,Citation36]. In the cartilage of osteoarthritis patients, matrix metalloproteinases expressed in local inflammatory cells promote collagen degradation [Citation37,Citation38]. Collagen degradation has been shown to result in the release of Pyr [Citation16], suggesting that Pyr may function as an ECM-derived DAMP (ECM-DAMP). In the present study, we showed that Pyr elicits effects on PC12 cells via direct interaction with RAGE. Our data strongly support the theory that collagen-derived molecules are recognized by PRRs including RAGE. We also examined the possibility that hydroxyproline, a collagen-specific amino acid, acts as an alternative RAGE ligand, but no interaction was observed between RAGE and hydroxyproline (data not shown). The data indicate that RAGE recognizes Pyr as a specific recognition site in collagen.
The AGEs-RAGE axis has been shown to promote fibrosis and contribute to pathogenesis of diabetic complications by induction of epithelial to mesenchymal transition (EMT) that causes excessive collagen deposition in epithelial cells [Citation39–Citation41]. Notably, Nam and Nagaraj [Citation42] recently reported that AGEs in ECM proteins promote EMT of lens epithelial cells. Although the overall effects of Pyr-RAGE interaction are still unclear, the results of the present study imply that RAGE senses ECM damage by recognizing Pyr as an ECM-DAMP, and thereby contributes to the repair of tissue collagen by inducing EMT. Indeed, we recently identified the EMT-related effects of Pyr on renal epithelial cells (Murakami et al., unpublished data).
In conclusion, our data demonstrate that Pyr is an intrinsic ligand of RAGE. Moreover, the results suggest that RAGE senses the degradation of collagen, which is a major ECM protein. Therefore, these results can help to clarify the mechanisms of multiple disorders associated with collagen degradation and AGEs-related fibrosis.
Author contributions
T.U., F.H. and H.W. designed the research. Y.M., T.F., R.K. and T.H. performed and analyzed the experiments. Y.M., F.H. and H.W. wrote the manuscript.
Acknowledgments
We thank Dr. McTaggart Iain Peter (Meiji University) for helpful comments on the manuscript.
Disclosure statement
No potential conflict of interest was reported by the authors.
References
- Hayase F. Recent development of 3-deoxyosone related Maillard reaction products. Food Sci Technol Res. 2000;6:79–86.
- Neeper M, Schmidt AM, Brett J, et al Cloning and expression of a cell surface receptor for advanced glycosylation end products of proteins. J Biol Chem. 1992;267:14998–15004.
- Ott C, Jacobs K, Haucke E, et al Role of advanced glycation end products in cellular signaling. Redox Biol. 2014;2:411–429.
- Kierdorf K, Fritz G. RAGE regulation and signaling in inflammation and beyond. J Leukoc Biol. 2013;94:55–68.
- Srikanth V, Maczurek A, Phan T, et al Advanced glycation endproducts and their receptor RAGE in Alzheimer’s disease. Neurobiol Aging. 2011;32:763–777.
- Hori O, Brett J, Slattery T, et al The receptor for advanced glycation end products (RAGE) is a cellular binding site for amphoterin. Mediation of neurite outgrowth and co-expression of rage and amphoterin in the developing nervous system. J Biol Chem. 1995;270:25752–25761.
- Hofmann MA, Drury S, Fu C, et al RAGE mediates a novel proinflammatory axis: a central cell surface receptor for S100/calgranulin polypeptides. Cell. 1999;97:889–901.
- Chen GY, Nuñez G. Sterile inflammation: sensing and reacting to damage. Nat Rev Immunol. 2010;10:826–837.
- Takeuchi M, Bucala R, Suzuki T, et al Neurotoxicity of advanced glycation end-products for cultured cortical neurons. J Neuropathol Exp Neurol. 2000;59:1094–1105.
- Sato T, Iwaki M, Shimogaito N, et al TAGE (toxic AGEs) theory in diabetic complications. Curr Mol Med. 2006;6:351–358.
- Takeuchi M, Yamagishi S. Involvement of toxic AGEs (TAGE) in the pathogenesis of diabetic vascular complications and Alzheimer’s disease. J Alzheimers Dis. 2009;16:845–858.
- Usui T, Hayase F. Isolation and identification of the 3-hydroxy-5-hydroxymethyl-pyridinium compound as a novel advanced glycation end product on glyceraldehyde-related Maillard reaction. Biosci Biotechnol Biochem. 2003;67:930–932.
- Nagai R, Hayashi CM, Xia L, et al Identification in human atherosclerotic lesions of GA-pyridine, a novel structure derived from glycolaldehyde-modified proteins. J Biol Chem. 2002;277:48905–48912.
- Murakami Y, Fujino T, Hasegawa T, et al Receptor for advanced glycation end products (RAGE)-mediated cytotoxicity of 3-hydroxypyridinium derivatives. Biosci Biotechnol Biochem. 2018;82:312–319.
- Fujimoto D, Akiba K-Y, Nakamura N. Isolation and characterization of a fluorescent material in bovine achilles tendon collagen. Biochem Biophys Res Commun. 1977;76:1124–1129.
- Saito M, Marumo K. Effects of collagen crosslinking on bone material properties in health and disease. Calcif Tissue Int. 2015;97:242–261.
- Robins SP, Stewart P, Astbury C, et al Measurement of the cross linking compound, pyridinoline, in urine as an index of collagen degradation in joint disease. Ann Rheum Dis. 1986;45:969–973.
- Delmas PD, Schlemmer A, Gineyts E, et al Urinary excretion of pyridinoline crosslinks correlates with bone turnover measured on iliac crest biopsy in patients with vertebral osteoporosis. J Bone Miner Res. 1991;6:639–644.
- Risteli J, Elomaa I, Niemi S, et al Radioimmunoassay for the pyridinoline cross-linked carboxy-terminal telopeptide of type I collagen: a new serum marker of bone collagen degradation. Clin Chem. 1993;39:635–640.
- Robins SP, Duncan A, Wilson N, et al Standardization of pyridinium crosslinks, pyridinoline and deoxypyridinoline, for use as biochemical markers of collagen degradation. Clin Chem. 1996;42:1621–1626.
- Strober W. Trypan blue exclusion test of cell viability. Curr Protoc Immunol. 2001;21:A3B1–A3B2.
- Santilli F, Vazzana N, Bucciarelli LG, et al Soluble forms of RAGE in human diseases: clinical and therapeutical implications. Curr Med Chem. 2009;16:940–952.
- Thomas J, Elsden DF, Partridge SM. Degradation products from elastin: partial structure of two major degradation products from the cross-linkages in elastin. Nature. 1963;200:651–652.
- Siegel RC, Fu JC, Uto N, et al Collagen cross-linking: lysyl oxidase dependent synthesis of pyridinoline in vitro: confirmation that pyridinoline is derived from collagen. Biochem Biophys Res Commun. 1982;108:1546–1550.
- Barber M, Bordoli RS, Elliott GJ, et al The structure(s) of pyridinoline(s). Biochem Biophys Res Commun. 1982;109:1041–1046.
- Daffu G, del Pozo CH, O’Shea KM, et al Radical roles for RAGE in the pathogenesis of oxidative stress in cardiovascular diseases and beyond. Int J Mol Sci. 2013;14:19891–19910.
- Usui T, Shimohira K, Watanabe H, et al Detection and determination of glyceraldehyde-derived pyridinium-type advanced glycation end product in streptozotocin-induced diabetic rats. Biosci Biotechnol Biochem. 2007;71:442–448.
- James I, Crowley C, Perrett D. Assay of pyridinium crosslinks in serum using narrow-bore ion-paired reversed-phase high-performance liquid chromatography. J Chromatogr. 1993;612:41–48.
- Abbiati G, Rigoldi M, Frignani S, et al Determination of pyridinium crosslinks in plasma and serum by high-performance liquid chromatography. J Chromatogr B Biomed Appl. 1994;656:303–310.
- Kaczmarek A, Vandenabeele P, Krysko DV. Necroptosis: the release of damage-associated molecular patterns and its physiological relevance. Immunity. 2013;38:209–223.
- Termeer C, Benedix F, Sleeman J, et al Oligosaccharides of Hyaluronan activate dendritic cells via toll-like receptor 4. J Exp Med. 2002;195:99–111.
- Scheibner KA, Lutz MA, Boodoo S, et al Hyaluronan fragments act as an endogenous danger signal by engaging TLR2. J Immunol. 2006;177:1272–1281.
- Okamura Y, Watari M, Jerud ES, et al The extra domain A of fibronectin activates Toll-like receptor 4. J Biol Chem. 2001;276:10229–10233.
- Hwang HS, Park SJ, Cheon EJ, et al Fibronectin fragment-induced expression of matrix metalloproteinases is mediated by MyD88-dependent TLR-2 signaling pathway in human chondrocytes. Arthritis Res Ther. 2015;17:320.
- Harrington DJ. Bacterial collagenases and collagen-degrading enzymes and their potential role in human disease. Infect Immun. 1996;64:1885–1891.
- Watanabe K. Collagenolytic proteases from bacteria. Appl Microbiol Biotechnol. 2004;63:520–526.
- Tchetverikov I, Lohmander LS, Verzijl N, et al MMP protein and activity levels in synovial fluid from patients with joint injury, inflammatory arthritis, and osteoarthritis. Ann Rheum Dis. 2005;64:694–698.
- Burrage PS, Mix KS, Brinckerhoff CE. Matrix metalloproteinases: role in arthritis. Front Biosci. 2006;11:529–543.
- Oldfield MD, Bach LA, Forbes JM, et al Advanced glycation end products cause epithelial-myofibroblast transdifferentiation via the receptor for advanced glycation end products (RAGE). J Clin Invest. 2001;108:1853–1863.
- Simonson MS. Phenotypic transitions and fibrosis in diabetic nephropathy. Kidney Int. 2007;71:846–854.
- Raghavan CT, Nagaraj RH. AGE-RAGE interaction in the TGFβ2-mediated epithelial to mesenchymal transition of human lens epithelial cells. Glycoconj J. 2016;33:631–643.
- Nam MH, Nagaraj RH. Matrix-bound AGEs enhance TGFβ2-mediated mesenchymal transition of lens epithelial cells via the noncanonical pathway: implications for secondary cataract formation. Biochem J. 2018;475:1427–1440.