ABSTRACT
DDL1 encodes a mitochondrial phospholipase A1 involved in acyl chain remodeling of mitochondrial phospholipids and degradation of cardiolipin in Saccharomyces cerevisiae. The deletion of DDL1 leads to respiratory growth defects. To elucidate the physiological role of DDL1, we screened for genes that, when overexpressed, suppress the respiratory growth defect of the DDL1 deletion mutant. Introduction of COQ8, COQ9, or COQ5, which are involved in coenzyme Q (CoQ) synthesis, using a multicopy vector suppressed the respiratory growth defect of the DDL1 deletion mutant. In contrast, introduction of COQ8 using a multicopy vector did not accelerate the growth of the deletion mutants of TAZ1 or CLD1, which encode an acyltransferase or phospholipase A2, respectively, involved in the remodeling of cardiolipin. These results suggest genetic interactions between the mitochondrial phospholipase A1 gene and the genes involved in CoQ synthesis.
Graphical Abstract
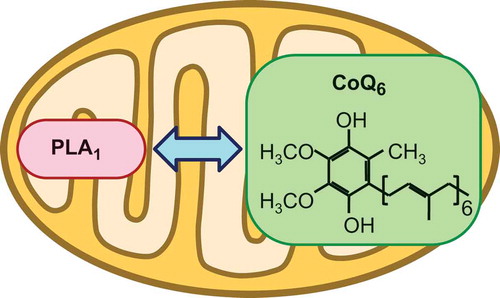
Abbreviations: CoQ: coenzyme Q; CL: cardiolipin; ORF: open reading frame; PA: phosphatidic acid; PC: phosphatidylcholine; PE: phosphatidylethanolamine; PG: phosphatidylglycerol; PLA1: phospholipase A1; iPLA1: intracellular PLA1; PLA2: phospholipase A2
Genetic interaction between DDL1 encoding a mitochondrial phospholipase A1 and genes involved in coenzyme Q synthesis.
Phospholipase A1 (PLA1) is a group of enzymes that hydrolyze glycerophospholipids at the sn-1 position, producing fatty acids and 2-acyl-lysophospholipids. PLA1 enzymes are classified as extracellular PLA1 and intracellular PLA1 (iPLA1) [Citation1]. Mammals have three types of iPLA1 enzymes, PA-PLA1/DDHD1/iPLA1α, p125/Sec23IP/iPLA1β, and KIAA0725p/DDHD2/iPLA1γ; while plants, nematodes, and yeasts have only PA-PLA1 orthologs. In the yeast Saccharomyces cerevisiae, the PA-PLA1 ortholog is encoded by DDL1. Ddl1 has been shown to have phospholipase activity toward phosphatidylethanolamine (PE), phosphatidylglycerol (PG), cardiolipin (CL), phosphatidylcholine (PC), and phosphatidic acid (PA) in vitro [Citation2,Citation3]. In mammals, PA-PLA1 localizes in the cytosol, and its overproduction has been found to induce mitochondrial fragmentation, while its knockdown caused elongation of the mitochondria [Citation4], suggesting that PA-PLA1 regulates mitochondrial morphology. In contrast, Ddl1 has been shown to localize to the matrix of mitochondria [Citation3,Citation5,Citation6] and DDL1 deletion leads to mitochondrial fragmentation [Citation2] and a defect in respiratory growth [Citation7]. Therefore, the role of Ddl1 in the mitochondrial morphology appears to be different from that of mammalian PA-PLA1. In addition, analysis of the phospholipid metabolism in the DDL1 deletion mutant and in the DDL1 overproducing strain has suggested that Ddl1 is involved in the acyl chain remodeling of mitochondrial phospholipids, including CL, and in the degradation of CL [Citation2]. However, the physiological role of Ddl1 remains poorly understood.
Coenzyme Q (CoQ, ubiquinone) is a widely conserved lipophilic compound that plays a critical role in the electron transport chain and oxidation–reduction reactions catalyzed by various enzymes [Citation8–Citation11]. CoQ is composed of quinone and isoprenoid side chain. The length of the isoprenoid side chain varies depending on the organism. While the CoQ of human and Schizosaccharomyces pombe has ten isoprene units (CoQ10), that of S. cerevisiae has six isoprene units (CoQ6). In S. cerevisiae, eleven genes, COQ1–COQ11, have been shown to be involved in CoQ synthesis. The products of these COQ genes localize to mitochondria. It has been shown that deletion mutations in any of the genes COQ1–COQ10 result in respiratory growth defects. Among these, the gene products Coq1–Coq3 and Coq5–Coq7 have been shown to catalyze reactions involved in CoQ synthesis. The roles of Coq4 and Coq8–Coq11 remain elusive. In S. cerevisiae, it has been proposed that Coq2–Coq7 and Coq9 form a large complex, the CoQ synthome [Citation12]. Coq8 has been cast as a putative protein kinase that is involved in the phosphorylation of Coq3, Coq5, and Coq7 [Citation13]. It has been proposed that Coq9 is a deaminase that removes amino groups of CoQ intermediates, imino-demethyl-demethoxy-Q6 or imino-demethoxy-Q6 [Citation14].
To clarify the physiological role of Ddl1, we screened for genes that, when overexpressed, suppress the respiratory growth defect of the DDL1 deletion mutant. Our results suggest genetic interactions between DDL1 and CoQ synthesis genes.
Materials and methods
Strains and media
Yeast strains used in this study are listed in .
Table 1. Strains used in this study.
S. cerevisiae was cultured at 30°C in the minimal medium containing 0.17% yeast nitrogen base (w/o amino acid and ammonium sulfate, Difco), 0.5% ammonium sulfate, and 2% glucose (SD medium), 3% glycerol (SGly medium), 3% glycerol with 0.5% glucose (SGlyD medium), 4.4% Lactate (pH 5.5) (SLac medium), or 1% ethanol (SE medium), or semi-synthetic lactate medium (4.4% lactate, 1.6% NaOH, 0.17% yeast nitrogen base without amino acid and ammonium sulfate, 0.5% ammonium sulfate, 0.3% yeast extract, 0.05% glucose, 0.05% CaCl2⋅2H2O, 0.05% NaCl, 0.06% MgCl2⋅6H2O, 0.1% NH4Cl, 0.1% KH2PO4, pH 5.5).
Plasmids and primers used for the strain construction are listed in Table S1 and Table S2. DDL1 was deleted with URA3 of Kluyveromyces lactis as a marker using the DNA fragment prepared by PCR with pUG72 [Citation15] and primers, DDL1-DEL-U and DDL1-DEL-L. Deletion of DDL1 was confirmed by PCR using primers, DDL1-confirm-U and DDL1-confirm-L. DDL1 was also deleted with ADE2 using the DNA fragment prepared by PCR with the total DNA of the DLY09 strain [Citation16] and primers, EGFP_EcoRI_up_DDL1 and EGFP_KpnI_DDL1. Deletion was confirmed by PCR using primers, DDL1_check and EGFP_KpnI_DDL1.
The strains that express Coq9 tagged with HA epitope was constructed as follows: PCR was performed using primers coq9_F2 and coq9_R1 with pFA6a-3HA-TRP1 [Citation17] as a template. An amplified fragment was introduced into ddl1∆, resulting in W303-1Addl1∆COQ9-HA. A fragment amplified using XhoI_Coq9p_fw and KpnI_down_coq9 from the total DNA of W303-1Addl1∆COQ9-HA was introduced into W303-1A, to obtain W303-1ACOQ9-HA.
Plasmids
Plasmids used in this study are listed in Table S1, and primers used for the plasmid construction are listed in Table S2.
The low copy plasmid harboring DDL1 was constructed as follows: The ORF of DDL1 with its 5ʹ- and 3ʹ-adjacent regions were amplified using primers EGFP_EcoRI_up_DDL1 and EGFP_KpnI_DDL1 with the total DNA of W303-1A. An amplified fragment was digested with EcoRI and KpnI, and cloned into the EcoRI-KpnI sites of YCplac111 [Citation18] to obtain YCplac111-DDL1.
Multicopy plasmid harboring COQ8 was constructed as follows: The ORF of COQ8 with its 5ʹ- and 3ʹ-adjacent regions were amplified using primers EcoRI_up_coq8 and KpnI_down_coq8 with the total DNA of W303-1A. An amplified fragment was digested with EcoRI and KpnI, and cloned into the EcoRI-KpnI sites of YEplac181 [Citation18] to obtain YEp181-COQ8.
Multicopy plasmids harboring MCP2, MCO76, COQ3, and COQ5 were constructed as follows: The ORFs of MCP2, MCO76, COQ3, and COQ5 with their 5ʹ- and 3ʹ-adjacent regions were amplified using primers SacI_up_MCP2 and KpnI_down_MCP2, SacI_up_YPL109C and KpnI_down_YPL109C, SacI_up_coq3 and KpnI_down_coq3, and SacI_up_coq5 and KpnI_down_coq5 with the total DNA of W303-1A. Amplified fragments were digested with SacI and KpnI, and cloned into the SacI-KpnI sites of YEplac181 to obtain YEp181-MCP2, YEp181-MCO76, YEp181-COQ3, and YEp181-COQ5, respectively.
Multicopy plasmid harboring COQ9 was constructed as follows: The ORF of COQ9 with its 5ʹ- and 3ʹ-adjacent regions were amplified using primers EcoRI_up_coq9 and KpnI_down_coq9 with the total DNA of W303-1A. An amplified fragment was digested with EcoRI and KpnI, and cloned into the EcoRI-KpnI sites of YEplac181 to obtain YEp181-COQ9.
Multicopy plasmids harboring COQ7 was constructed as follows: The ORF of COQ7 with its 5ʹ- and 3ʹ-adjacent regions were amplified using primers EcoRI_up_coq7 and SacI_down_coq7 with the total DNA of W303-1A. An amplified fragment was digested with EcoRI and SacI, and cloned into the EcoRI-SacI sites of YEplac181 to obtain YEp181-COQ7.
After PCR amplification, the DNA fragments were checked by sequence analysis.
Preparation of whole cell extract and western blot analysis
Yeast cells were suspended in the breaking buffer (25 mM HEPES-KOH (pH 7.4), 100 mM KCl, 10% (w/v) glycerol, 1 mM DTT) containing 1% (v/v) protease inhibitor cocktail (Sigma), and disrupted by shaking with glass beads 6 times for 30 sec with 1 min cooling intervals at 0°C using Multi-Beads Shocker (YASUI KIKAI). After centrifugation at 1,000 x g for 5 min at 4°C, the supernatant was subjected to western blot analysis as described previously [Citation19].
Analysis of growth
Strains were cultured to logarithmic phase in SD medium, and then spotted onto plates with indicated carbon sources in 10-fold serial dilutions starting with 5 μL of 0.3 or 0.01 OD600 units/mL. Cells were incubated at 30°C.
CoQ extraction and measurement
CoQ from yeasts was extracted as described previously [Citation20]. The yeast cells were cultivated in 55 mL SD supplemented with Ade, His, Trp, and Ura (starting concentration was approx. 1 × 106 cells/mL) for about 24 hours with rotation at 30℃. Cells were harvested from each of 50 mL culture. The CoQ crude extract was analyzed by normal-phase thin layer chromatography (TLC) with authentic CoQ6 or CoQ10 standard. 10 μg of CoQ10 was used for internal standard of CoQ extraction. Normal-phase TLC was conducted on a Kieselgel 60 F254 plate and developed with benzene. The plate was viewed under UV illumination, the CoQ band was collected, and the sample was extracted with hexane/isopropanol (1:1, v/v). Samples were dried and solubilized in ethanol. Purified CoQ was subjected to high-performance liquid chromatography (Shimadzu HPLC Class VP series) equipped with a reverse phase YMC-Pack ODS-A column and detected at a wavelength of 275 nm. Ethanol was used as the mobile phase at a flow rate of 1.0 mL/min.
Results
Screening for multicopy suppressors of the respiratory defect of DDL1 deletion mutant
It was reported that the DDL1 deletion mutant from the yeast knockout collection showed growth defects on non-fermentable carbon sources [Citation7]. Deletion mutants of DDL1 were constructed in W303-1A by deleting DDL1 using URA3 of K. lactis or ADE2 of S. cerevisiae (ddl1∆U and ddl1∆, respectively), and their growth was analyzed. The ddl1∆ strain grew as well as the wild-type strain on glucose, but showed growth defects on non-fermentable carbon sources, such as glycerol, lactate, and ethanol ()). In addition, the ddl1∆U strain also showed growth defects on glycerol (data not shown).
Figure 1. Suppression of the defect in respiratory growth of the ddl1∆ strain by overexpression of COQ8.
(A) The wild-type W303-1A strain (WT) harboring YCplac111 and the ddl1∆ strain harboring YCplac111, YCplac111-DDL1 (YCp-DDL1), YEp13, the clone obtained from the screening (Library clone), YEplac181, or YEp181-COQ8 were cultured in the SD medium to logarithmic phase and spotted onto the SD (Glc) or SGly (Gly) medium in 10-fold serial dilutions. Strains were cultured for 2 days on the SD medium or 3 days on SGly medium. (B) The wild-type W303-1A strain (WT) and the ddl1∆ strain harboring YEplac181 (Vec) or YEp181-COQ8 (COQ8) were cultured in the SD medium to logarithmic phase and spotted onto the SD (Glc), SGly (Gly), SLac (Lac), or SE (EtOH) medium in 10-fold serial dilutions. Strains were cultured for 2 days on the SD medium, 3 days on the SGly medium, 5 days on the SLac medium, or 4 days on the SE medium.
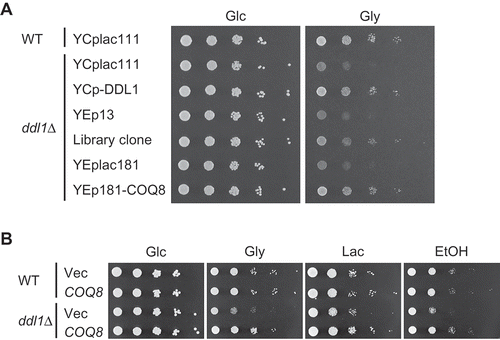
To investigate the physiological role of Ddl1, we screened for genes that, when overexpressed, suppress the growth defect of the ddl1∆U strain on glycerol. A multicopy genomic library was introduced into the ddl1∆U strain, and clones that improved the growth on glycerol were isolated. By screening 157,000 transformants, nine strains that harbor plasmids containing COQ8 and its adjacent chromosomal regions were obtained. As expected, 50 strains harboring plasmids containing DDL1 were also isolated. To confirm that the overexpression of COQ8 suppresses the respiratory growth defect of the DDL1 deletion mutant, a multicopy plasmid containing the open reading frame (ORF) of COQ8, along with its promoter and terminator regions, were constructed and introduced into the ddl1∆ strain. Introduction of the multicopy plasmid containing COQ8 suppressed the growth defect of the ddl1∆ strain on glycerol, lactate, and ethanol (,)). On glucose, the ddl1∆ strain harboring the COQ8-containing multicopy plasmid grew as well as the wild-type strain.
Suppression of the mitochondrial defects of ddl1∆ by COQ genes
Coq8 belongs to the UbiB protein kinase family. S. cerevisiae has two other UbiB protein kinases, Mcp2 and Mco76. Mcp2 is a mitochondrial protein involved in lipid homeostasis and mitochondrial morphology [Citation21]. Mco76 is a mitochondrial protein, but its function is unknown [Citation22]. Introduction of MCP2 and MCO76 into the ddl1∆ strain using the multicopy vector did not improve the growth of the ddl1∆ strain on glycerol ()). This shows that suppression of the respiratory growth defect of the ddl1∆ strain is specific to Coq8 and not a general trait of the UbiB protein kinase family members.
Figure 2. Specific suppression by COQ8 of the defect in respiratory growth of the ddl1∆ strain.
(A) The wild-type W303-1A strain (WT) harboring YEplac181 (Vec) and the ddl1∆ strain harboring YEplac181 (Vec), YEp181-COQ8 (COQ8), YEp181-MCP2 (MCP2) or YEp181-MCO76 (MCO76) were cultured in the SD medium to logarithmic phase and spotted onto the SD (Glc) or SGly (Gly) medium in 10-fold serial dilutions. Strains were cultured for 2 days on the SD medium or 3 days on SGly medium. (B) The wild-type BY4741 strain (WT) harboring YEplac181 (Vec), and the BY4741ddl1∆ strain (ddl1∆), BY4741taz1∆ strain (taz1∆), and BY4741cld1∆ strain (cld1∆) harboring YEplac181 (Vec) and YEp181-COQ8 (COQ8) were spotted onto the SD (Glc) or SGly (Gly) medium and incubated as described in (A).
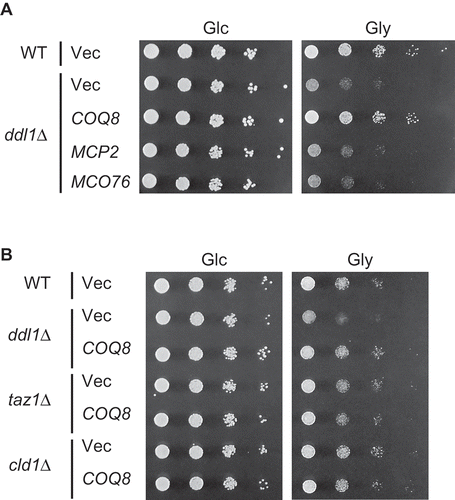
It has been proposed that Ddl1, together with phospholipase A2 (PLA2) Cld1 and acyl transferase Taz1 is involved in acyl chain remodeling of CL [Citation2]. Deletion mutants of CLD1 and TAZ1 did not show defective growth on glycerol, and introduction of a multicopy plasmid containing COQ8 did not improve their growth ()), which implies that suppression of the respiratory growth defect of the ddl1∆ strain by COQ8 is not related to CL remodeling.
In the screening for multicopy suppressor genes of the respiratory growth defect of the ddl1∆ strain, described above, a transformant that has a plasmid containing COQ9 with its adjacent region was isolated. A multicopy plasmid containing COQ9 was then constructed and introduced into the ddl1∆ strain. This restored the respiratory growth defect of the ddl1∆ strain (). It has been suggested that Coq8 is involved in phosphorylation of Coq3, Coq5, and Coq7 [Citation13]. We assessed whether overexpression of COQ3, COQ5, and COQ7 suppresses the respiratory growth defect of the ddl1∆ strain. Introduction of the multicopy plasmid containing COQ5, but not those containing COQ3 or COQ7, partially improved the growth of the ddl1∆ strain on glycerol (). These results highlight genetic interactions between DDL1 and genes involved in CoQ synthesis.
Figure 3. Suppression of the defect in respiratory growth of the ddl1∆ strain by COQ genes.
The wild-type W303-1A strain (WT) harboring YEplac181 and the ddl1∆ strain harboring YEplac181 (Vec), YEp181-COQ3 (COQ3), YEp181-COQ5 (COQ5), YEp181-COQ7 (COQ7), YEp181-COQ8 (COQ8), or YEp181-COQ9 (COQ9) were cultured in the SD medium to logarithmic phase and spotted onto the SD (Glc) or SGly (Gly) medium in 10-fold serial dilutions. Strains were cultured for 2 days on the SD medium or 3 days on the SGly medium.
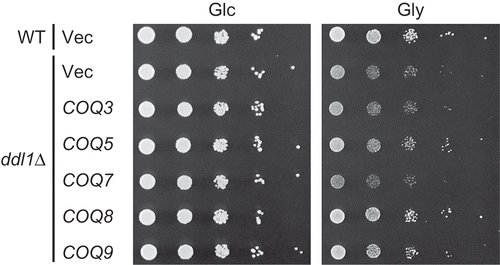
Given that the overexpression of COQ8, COQ9, and COQ5 suppressed the respiratory growth defect of the ddl1∆ strain, we postulated that the growth defect of the ddl1∆ strain was alleviated by an increased CoQ synthesis. To test this hypothesis, the wild-type and ddl1∆ strains, with or without the multicopy plasmid containing COQ8 or COQ9, were cultured in the SD medium and their intracellular CoQ6 levels were determined (). Although statistically significant differences in CoQ6 levels were not observed among the strains, the ddl1∆ strain showed a tendency toward lower level of CoQ6 when compared to the wild-type strain. In addition, introduction of DDL1 using a low-copy plasmid, or COQ8 or COQ9 using the multicopy plasmid, tended to increase the levels of CoQ6 in the ddl1∆ strain. These results raise the possibility that DDL1 is involved in the synthesis of CoQ6.
Figure 4. CoQ6 amount in W303-1A and Δddl1 background strains.
CoQ6 was extracted from the wild-type W303-1A strain harboring YEplac181 (WT/YEp), and the ddl1∆ strain harboring YEplac181 (ddl1∆/YEp), YCplac111-DDL1 (ddl1∆/YCp-DDL1), YEp181-COQ8 (ddl1∆/YEp-COQ8), or YEp181-COQ9 (ddl1∆/YEp-COQ9) and analyzed by HPLC as described in materials and methods. Bars show CoQ6 amounts normalized with cell number (x109 cells). The values are the means and SEM of the results from four independent experiments. Statistical analysis was performed using Student’s unpaired t-test.
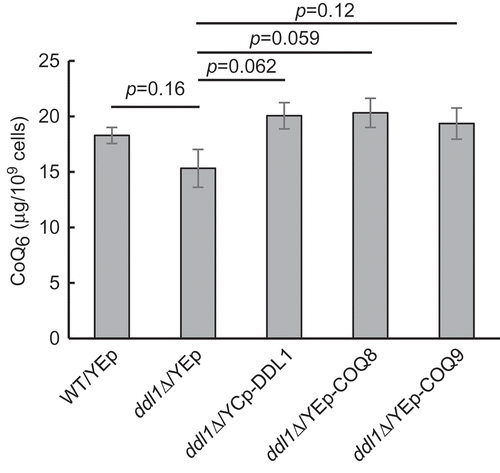
Finally, to investigate the effect of DDL1 deletion and COQ8 overexpression on the level of Coq9, strains that express a fusion gene, which encodes Coq9 tagged with 3xHA epitope (Coq9-HA) under the native COQ9 promoter at the native chromosomal locus, in the wild-type and the ddl1∆ strain background were constructed. The wild-type strain that expressed Coq9-HA instead of Coq9 grew on glycerol, albeit slightly less than its parental strain ()). These results suggest that Coq9-HA is partially functional. The amount of Coq9-HA was determined in the wild-type and ddl1∆ strains with or without COQ8 overexpression ()). Overexpression of COQ8 suppressed the growth defect of the ddl1∆ strain expressing Coq-9-HA, as well as that of the ddl1∆ strain. Deletion of DDL1 did not significantly affect on the amount of Coq9-HA produced. However, overexpression of COQ8 increased the levels of Coq9-HA. These results raise the possibility that suppression of the respiratory growth defect of the ddl1∆ strain by overexpression of COQ8 relies, at least in part, on increasing the amount of Coq9.
Figure 5. Amounts of Coq9-HA in the wild-type and the ddl1∆ strains in the presence or absence of COQ8 overexpression.
(A) Growth of the wild-type and the ddl1∆ strains producing Coq9-HA. The wild-type W303-1A (W303), W303-1ACOQ9-HA (W303-COQ9-HA), ddl1∆, and ddl1∆COQ9-HA strains harboring YEplac181 (Vec) or YEp181-COQ8 (COQ8) were cultured in the SD medium to logarithmic phase and spotted onto the SD (Glc) or SGly (Gly) medium in 10-fold serial dilutions. Strains were cultured for 2 days on the SD medium or 3 days on SGly medium. (B) The W303-1ACOQ9-HA (W303-COQ9-HA) and ddl1∆COQ9-HA strains harboring YEplac181 or YEp181-COQ8 were cultured in the SD medium to logarithmic phase. Whole cell extracts were prepared and subjected to western blot analysis using anti-HA and anti-Pgk1 antibodies.
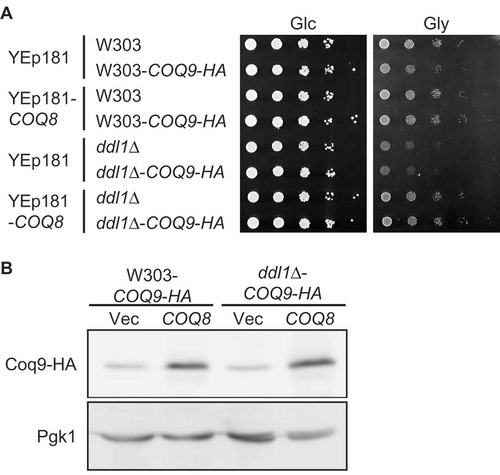
Discussion
In this study, we screened for genes that, when overexpressed, suppress the respiratory growth defect of the ddl1∆ strain. We have found that COQ8 overexpression suppressed the respiratory growth defect of the ddl1∆ strain, and overexpression of COQ9 and COQ5 partially suppressed it ( and ). Although statistically significant differences were not observed, the ddl1∆ strain exhibited a tendency toward lower CoQ6 content than that of the wild-type strain, and the levels of CoQ6 in the ddl1∆ strain tended to increase upon the overexpression of COQ8 and COQ9 (). These results suggest genetic interactions between DDL1 and the genes involved in CoQ formation. Involvement of DDL1 in CoQ synthesis is an attractive model. Under this model, the respiratory defect observed in the ddl1∆ strain could be due to decreased level of CoQ6, which could be recovered to various extents by overexpression of COQ8, COQ9, and COQ5. We determined the CoQ levels in the yeast cells that were cultured in the medium containing glucose, because our wild-type and ddl1∆ strains containing the plasmids did not grow in the liquid SGly medium. It has been reported that the CoQ level increased in the cells cultured in the medium containing glycerol and transcription of COQ4, COQ5, COQ7, and COQ8 was induced by glycerol [Citation23–Citation25]. It is possible that the differences in the CoQ levels among the wild-type and ddl1∆ strains are more significant in the cells cultured on glycerol than in those on glucose. It has been reported that Coq8 is involved in phosphorylation of Coq3, Coq5, and Coq7 [Citation13]; and that overexpression of Coq8 can suppress the respiratory defect of a coq9 mutant [Citation26], and stabilize Coq complexes in various coq mutants [Citation12]. Furthermore, the amount of Coq9-HA increased upon COQ8 overexpression ()). The overexpression of COQ8 probably improved CoQ synthesis by stabilizing the Coq complex through phosphorylation of its components. The crystal structure analysis of human COQ9 revealed that it has a hydrophobic pocket, in which phospholipid species bind [Citation27]. Therefore, it is possible that Ddl1 regulates CoQ synthesis by modulating the binding of Coq9 with phospholipids through its phospholipase activity. It would be of interest to analyze the lipid species that bind to Coq9 in S. cerevisiae. Alternatively, since both COQ9 and COQ5 have been proposed to be involved in the synthesis of demethoxy-Q6 in the CoQ synthetic pathway [Citation14], overexpression of COQ9 and COQ5 might result in an increase in demethoxy-Q6. Therefore, it is plausible that Ddl1 is involved in the synthesis of demethoxy-Q6.
Because the difference in CoQ levels between the wild-type and ddl1∆ strains was not statistically significant, Ddl1 might not be involved in CoQ synthesis. It is possible that the mitochondrial function was impaired in the ddl1∆ strain through alteration in the lipid composition of the mitochondria, which was then improved by increase in CoQ levels upon overexpression of COQ8, COQ9, and COQ5.
PA-PLA1 enzymes are widely conserved in eukaryotes, but their localization and functions appear to vary [Citation1–Citation4]. In Schizosaccharomyces pombe, a PA-PLA1 ortholog was suggested to localize to the mitochondria [Citation28]. It is of interest to assess whether the functions of PA-PLA1 enzymes are conserved in fungi. The findings obtained in this study will contribute to our understanding the physiological role of PLA1 in yeasts.
Acknowledgments
This work was done using the facilities of the Biotechnology Research Center of the University of Tokyo.
Disclosure statement
No potential conflict of interest was reported by the authors.
Supplementary material
Supplementary data can be accessed here
Additional information
Funding
References
- Tani K, Kogure T, Inoue H. The intracellular phospholipase A1 protein family. Biomol Concepts. 2012 Oct;3(5):471–478. PubMed PMID: 25436551; eng.
- Yadav PK, Rajasekharan R. Misregulation of a DDHD domain-containing lipase causes mitochondrial dysfunction in yeast. J Biol Chem. 2016 Aug;291(35):18562–18581. PubMed PMID: 27402848; PubMed Central PMCID: PMCPMC5000100. eng.
- Urafuji K, Arioka M. Yor022c protein is a phospholipase A. Biochem Biophys Res Commun. 2016 Nov;480(3):302–308. PubMed PMID: 27746179; eng.
- Baba T, Kashiwagi Y, Arimitsu N, et al. Phosphatidic acid (PA)-preferring phospholipase A1 regulates mitochondrial dynamics. J Biol Chem. 2014 Apr;289(16):11497–11511. PubMed PMID: 24599962; PubMed Central PMCID: PMCPMC4036285. eng.
- Huh WK, Falvo JV, Gerke LC, et al. Global analysis of protein localization in budding yeast. Nature. 2003 Oct 16;425(6959):686–691. PubMed PMID: 14562095.
- Reinders J, Zahedi RP, Pfanner N, et al. Toward the complete yeast mitochondrial proteome: multidimensional separation techniques for mitochondrial proteomics. J Proteome Res. 2006 Jul;5(7):1543–1554. PubMed PMID: 16823961; eng.
- Steinmetz LM, Scharfe C, Deutschbauer AM, et al. Systematic screen for human disease genes in yeast. Nat Genet. 2002 Aug;31(4):400–404. PubMed PMID: 12134146; eng.
- Kawamukai M. Biosynthesis of coenzyme Q in eukaryotes. Biosci Biotechnol Biochem. 2016;80(1):23–33. PubMed PMID: 26183239; eng.
- Acosta MJ, Vazquez Fonseca L, Desbats MA, et al. Coenzyme Q biosynthesis in health and disease. Biochim Biophys Acta. 2016 Aug;1857(8):1079–1085. PubMed PMID: 27060254; eng.
- Alcázar-Fabra M, Navas P, Brea-Calvo G. Coenzyme Q biosynthesis and its role in the respiratory chain structure. Biochim Biophys Acta. 2016 Aug;1857(8):1073–1078. PubMed PMID: 26970214; eng.
- Stefely JA, Pagliarini DJ. Biochemistry of mitochondrial coenzyme Q biosynthesis. Trends Biochem Sci. 2017 10;42(10):824–843. PubMed PMID: 28927698; PubMed Central PMCID: PMCPMC5731490. eng.
- He CH, Xie LX, Allan CM, et al. Coenzyme Q supplementation or over-expression of the yeast Coq8 putative kinase stabilizes multi-subunit Coq polypeptide complexes in yeast coq null mutants. Biochim Biophys Acta. 2014 Apr;1841(4):630–644. PubMed PMID: 24406904; PubMed Central PMCID: PMCPMC3959571. eng.
- Tauche A, Krause-Buchholz U, Rödel G. Ubiquinone biosynthesis in Saccharomyces cerevisiae: the molecular organization of O-methylase Coq3p depends on Abc1p/Coq8p. FEMS Yeast Res. 2008 Dec;8(8):1263–1275. PubMed PMID: 18801050; eng.
- He CH, Black DS, Nguyen TP, et al. Yeast Coq9 controls deamination of coenzyme Q intermediates that derive from para-aminobenzoic acid. Biochim Biophys Acta. 2015 Sep;1851(9):1227–1239. PubMed PMID: 26008578; PubMed Central PMCID: PMCPMC4516658. eng.
- Gueldener U, Heinisch J, Koehler GJ, et al. A second set of loxP marker cassettes for Cre-mediated multiple gene knockouts in budding yeast. Nucleic Acids Res. 2002 Mar;30(6):e23. PubMed PMID: 11884642; PubMed Central PMCID: PMCPMC101367. eng.
- Deng L, Fukuda R, Kakihara T, et al. Incorporation and remodeling of phosphatidylethanolamine containing short acyl residues in yeast. Biochim Biophys Acta. 2010 Jun;1801(6):635–645. PubMed PMID: PMID: 20176132; S1388-1981(10)00043-0 [pii]; eng.
- Longtine MS, McKenzie A 3rd, Demarini DJ, et al. Additional modules for versatile and economical PCR-based gene deletion and modification in Saccharomyces cerevisiae. Yeast. 1998 Jul;14(10):953–961. PubMed PMID: 9717241; eng.
- Gietz RD, Sugino A. New yeast-Escherichia coli shuttle vectors constructed with in vitro mutagenized yeast genes lacking six-base pair restriction sites. Gene. 1988 Dec 30;74(2):527–534. PubMed PMID: 3073106; eng.
- Kobayashi S, Mizuike A, Horiuchi H, et al. Mitochondrially-targeted bacterial phosphatidylethanolamine methyltransferase sustained phosphatidylcholine synthesis of a Saccharomyces cerevisiae Δpem1 Δpem2 double mutant without exogenous choline supply. Biochim Biophys Acta. 2014 Sep;1841(9):1264–1271. PubMed PMID: 24832487; eng.
- Hayashi K, Ogiyama Y, Yokomi K, et al. Functional conservation of coenzyme Q biosynthetic genes among yeasts, plants, and humans. PLoS One. 2014;9(6):e99038. PubMed PMID: 24911838; PubMed Central PMCID: PMCPMC4049637. eng.
- Tan T, Ozbalci C, Brügger B, et al. Mcp1 and Mcp2, two novel proteins involved in mitochondrial lipid homeostasis. J Cell Sci. 2013 Aug;126(Pt 16):3563–3574. PubMed PMID: 23781023; eng.
- Morgenstern M, Stiller SB, Lübbert P, et al. Definition of a high-confidence mitochondrial proteome at quantitative scale. Cell Rep. 2017 Jun;19(13):2836–2852. PubMed PMID: 28658629; PubMed Central PMCID: PMCPMC5494306. eng.
- Belogrudov GI, Lee PT, Jonassen T, et al. Yeast COQ4 encodes a mitochondrial protein required for coenzyme Q synthesis. Arch Biochem Biophys. 2001 Aug;392(1):48–58. PubMed PMID: 11469793; eng.
- Hagerman RA, Willis RA. The yeast gene COQ5 is differentially regulated by Mig1p, Rtg3p and Hap2p. Biochim Biophys Acta. 2002 Oct;1578(1–3):51–58. PubMed PMID: 12393187; eng.
- Padilla S, Tran UC, Jiménez-Hidalgo M, et al. Hydroxylation of demethoxy-Q6 constitutes a control point in yeast coenzyme Q6 biosynthesis. Cell Mol Life Sci. 2009 Jan;66(1):173–186. PubMed PMID: 19002377; PubMed Central PMCID: PMCPMC3070445. eng.
- Johnson A, Gin P, Marbois BN, et al. COQ9, a new gene required for the biosynthesis of coenzyme Q in Saccharomyces cerevisiae. J Biol Chem. 2005 Sep;280(36):31397–31404. PubMed PMID: 16027161; eng.
- Lohman DC, Forouhar F, Beebe ET, et al. Mitochondrial COQ9 is a lipid-binding protein that associates with COQ7 to enable coenzyme Q biosynthesis. Proc Natl Acad Sci U S A. 2014 Nov;111(44):E4697–E4705. PubMed PMID: 25339443; PubMed Central PMCID: PMCPMC4226113. eng.
- Matsuyama A, Arai R, Yashiroda Y, et al. ORFeome cloning and global analysis of protein localization in the fission yeast Schizosaccharomyces pombe. Nat Biotechnol. 2006 Jul;24(7):841–847. PubMed PMID: 16823372; eng.