ABSTRACT
Rhamnogalacturonan II (RG-II) is a region of pectin macromolecules that is present in plant primary cell walls. RG-II can be solubilized from cell walls as a borate-RG-II complex (B-RG-II), where two RG-II fragments are cross-linked via a borate diester linkage. Here, a rabbit monoclonal antibody against B-RG-II was prepared, which recognized both B-RG-II and RG-II monomers without borate ester-crosslinking. A pectic fragment with unknown structure was also recognized by the antibody, but neither homogalacturonan nor rhamnogalacturonan I was recognized. Immunoelectron microscopic analyses of Arabidopsis root tip cells were performed using this antibody. The signal was detected in developing cell plates and cell walls, which were denser in longitudinal walls than in transverse walls. These results coincide with our previous results obtained in suspension cultured tobacco cells, confirming that RG-II is present in cell plates at an early stage of their assembly.
Abbreviations: B: boron; B-RG-II: borate-RG-II complex; ELISA: enzyme-linked immunosorbent assay; IgG: immunoglobulin G; mBSA: methylated bovine serum albumin; PGA: polygalacturonic acid; PLL: poly-l-lysine; RG-I: rhamnogalacturonan I; RG-II: rhamnogalacturonan II
Graphical Abstract
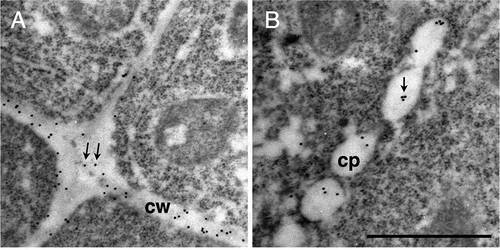
Immunogold labeling of rhamnogalacturonan II in Arabidopsis root cells using rabbit monoclonal antibody. Signals were detected in both cell walls (A) and cell plates (B).
Rhamnogalacturonan II (RG-II) is a pectic polysaccharide fragment that is released from plant cell walls through the action of endo-polygalacturonase [Citation1]. Although RG-II is a relatively small fragment with a size of approximately 5,000 Da, it is structurally very complex, consisting of a (1,4)-linked α-d-oligogalacturonic acid backbone with at least four side chains containing various rare sugars. In the cell wall, it occurs as a substructure of macromolecular pectin and is connected with other substructures including homogalacturonan and rhamnogalacturonan I (RG-I) [Citation1]. RG-II is enzymatically solubilized from the cell wall as a dimer that is covalently cross-linked by a borate diester bond (B-RG-II) [Citation2], indicating that RG-II serves as the site for cross-linking in pectin in muro. Pectins are retained in the cell walls unless the B-RG-II linkage is broken [Citation3]. Insufficient cross-linking of RG-II with borate diester results in structurally abnormal and mechanically weak cell walls [Citation4–Citation6]. These findings demonstrate that the RG-II domain critically contributes to the cell wall architecture. Thus, it is necessary to elucidate where RG-II is located within the cell walls of individual cells and tissues, and whether or not there is a change in its distribution during growth or in response to environmental stimuli. Immunocytochemical analysis using a RG-II-specific antibody is particularly suitable for the purpose.
A recombinant monovalent antibody fragment (Fab) specifically recognizing RG-II was selected from a phage display library of mouse immunoglobulin genes [Citation7]. In immunocytochemistry using this Fab, an alkaline treatment was necessary to detect signals, suggesting that the epitope recognized by the Fab is a structure masked by the esterification of carboxyl groups on uronic acid residues. A rabbit polyclonal anti-RG-II antibody was raised in our laboratory [Citation8] that gave signals in the cell walls without alkaline pretreatment, which has been used in many studies [Citation5,Citation9–Citation13]. Recently, we prepared another lot of rabbit polyclonal anti-RG-II antibody with properties similar to the previous one, and used the antibody to probe for RG-II during the formation of cell plates in suspension-cultured tobacco cells [Citation14]. However, one caveat of polyclonal antibodies is that it is not renewable. In addition, polyclonal antibodies may recognize multiple epitopes within the antigen, possibly making it difficult to interpret the results. Here, we report the production of a rabbit monoclonal anti-RG-II antibody as a new tool to study this polysaccharide, and its use in detecting RG-II in dividing cells in Arabidopsis root tips.
Materials and methods
Plant material
Seeds of Arabidopsis thaliana L. ecotype Columbia-0 (Col-0) were planted on half-strength Murashige and Skoog (MS) medium containing 29 mM sucrose and 0.8% (w/v) gellan gum. At 2 and 4 d after planting, the seedlings were transferred to new plates containing 50 and 100 mM sucrose, respectively, and then used for cryofixation at 24 h after transfer to the 100 mM-sucrose medium.
Preparation of antibody
For preparation of the antigen, B-RG-II was isolated from radish root cell walls as described previously [Citation2] and conjugated with methylated bovine serum albumin (mBSA) as previously described [Citation8]. Immunization of rabbits and the production of monoclonal antibodies were carried out by Abcam (Tokyo, Japan). Three rabbits were immunized with 0.5 mg B-RG-II conjugated with mBSA (B-RG-II-mBSA) and 4 additional injections with 0.25 mg of B-RG-II-mBSA at 3, 5, 7, and 9 weeks after the first injection. Titers of the rabbit sera were assayed with an enzyme-linked immunosorbent assay (ELISA) using a conjugate of B-RG-II and poly-l-lysine (PLL) as the immobilized antigen as described below. The spleen was taken from the rabbit whose serum showed the highest titer with the assay and then used for the cell fusion of lymphocytes and partner cells. Positive clones were screened from the pool of hybridomas by assessing the binding affinity of immunoglobulins secreted in the culture supernatants. One clone secreting immunoglobulin G (IgG) with the highest affinity to B-RG-II was selected and subjected to recombinant antibody production.
To assess the titers of sera during the antibody production, ELISAs were performed using B-RG-II-PLL conjugate as the immobilized antigen. The B-RG-II-PLL conjugate was prepared as previously described [Citation15]. Briefly, B-RG-II was dissolved in sterile distilled water at 1 mg/mL. A 160-µL aliquot of the B-RG-II solution was mixed with 800 µL of 0.01 M NaOH containing 0.001% (w/v) phenolphthalein. After mixing by vortex for 10 s, the pink solution was transferred to a tube containing 0.8 mg cyanuric chloride and mixed by vortex for 10 s while monitoring the solution until it turned colorless. The mixture was then added to 160 µL of 1 mg/mL PLL, mixed by vortex for 10 s, and incubated at 4°C for 2 h. The reaction was diluted by mixing with 1.44 mL of 50 mM sodium phosphate buffer (pH 7.0) and then used to coat the plate.
Competitive ELISA
B-RG-II was covalently linked to the amino-group modified surface of a microtiter plate (NH CovalinkTM; Nunc, Roskilde, Denmark) via carbodiimide coupling, which forms amide linkages between carboxylate groups in RG-II and amino groups on the plate. First, 50 µL of 60 μg/mL B-RG-II was added to each well, followed by 50 µL of 1 mg/mL 1-ethyl-3-(3-dimethylaminopropyl) carbodiimide (Dojindo Laboratories, Kumamoto, Japan). The plate was then incubated at 37°C for 2 h and washed twice with 250 µL PBS (137 mM NaCl, 8 mM Na2HPO4, 1.5 mM KH2PO4, and 2.7 mM KCl) containing 0.05% (w/v) Tween 20 (PBST). The plate was blocked with 250 µL of 0.5% (w/v) ovalbumin (Wako, Osaka, Japan) in PBST at 37°C for 1 h and washed twice with 250 µL PBST. The wells were added with 50 µL PBST containing different concentrations of competitors, then 50 µL of 0.5 ng/mL antibody 42–6 in PBST. The plate was incubated for 1 h at 37°C and washed three times with PBST. Next, 100 µL of goat anti-rabbit IgG antibody conjugated with peroxidase (Nacalai Tesque) diluted 10,000-fold with PBST was added to the wells. After a 1-h incubation at room temperature, the wells were washed three times with PBST, and then signals were detected using an ELISA POD substrate TMB kit (Nacalai Tesque). RG-I was prepared from radish cell wall as described previously [Citation16], except that DEAE-Sepharose FF and HiLoad Superdex columns (GE Healthcare) were used as the media for ion exchange and gel-permeation chromatography, respectively. Polygalacturonic acid (PGA) and esterified pectin, both were prepared from citrus, were obtained from Nacalai Tesque and Sigma-Aldrich, respectively.
Dot-blot analysis
B-RG-II was dissolved in 20 mM Tris-HCl (pH 8.0) at appropriate concentrations, and 1-µL aliquots were spotted onto a nylon membrane (Hybond-N+, GE Healthcare, Piscataway, NJ, USA). The air-dried membrane was blocked with Block Ace (DS Pharma Biomedical, Osaka, Japan) for 30 min. The blot was incubated with anti-RG-II antibody in binding buffer (a 9:1 [v/v] mixture of 10 mM phosphate buffer, pH 7.4, containing 0.05% Tween 20 and Block Ace solution) for 1 h at room temperature. The antibody concentrations used were 1 ng/mL and 2 µg/mL for the 42–6 and polyclonal antibodies, respectively. The blot was washed three times with washing buffer (a 9:1 [v/v] mixture of 10 mM phosphate buffer, pH 7.4, containing 0.1% Tween 20 and Block Ace solution) for 5 min each time.
The blot was then incubated with peroxidase-conjugated anti-rabbit secondary antibody (Nacalai Tesque, Kyoto, Japan), diluted 20,000-fold in binding buffer for 1 h at room temperature. After washing the blot 3 times for 5 min each, the signal was detected using a chemiluminescence substrate kit (Chemi-Lumi One Super, Nacalai Tesque).
High pressure frozen/freeze substitution of arabidopsis root tips
The following stock solutions were prepared at least 2 days before carrying out the high pressure frozen/freeze substitution experiment: stock solution A, 2% (v/v) glutaraldehyde dissolved in acetone (stored at – 20°C); stock solution B, 32% (v/v) 2,2-dimethoxypropane dissolved in acetone (stored at – 20°C). Stock solutions A, B, and acetone were mixed at a ratio of 1:1:2 (v/v) to make the freeze substitution solution.
Roots less than 3 mm in length were cut from seedlings and immersed in 0.2 M sucrose in a petri dish. The roots were transferred onto an aluminum specimen carrier and frozen in a HPM-010 high pressure freezer (Bal-Tec AG, Balzers, Lichtenstein). The frozen samples were immediately transferred into liquid-nitrogen. They were removed from the carrier in liquid nitrogen and transferred to cryovials held in liquid nitrogen.
Two mL of the freeze substitution solution was transferred into 10-mL glass vials, and the vials were transferred to a polystyrene foam container filled with liquid nitrogen. The specimens in liquid nitrogen were poured onto the frozen surface of the freeze substitution solution. The glass vials were stored at – 80°C for 4 d to allow for the substitution to proceed. The vials were then gradually warmed at – 20°C for 2 h, 4°C for 2 h, and room temperature for 2 h. The specimens were washed for 15 min in acetone 4 times, and then dehydrated in 99.5% ethanol for 15 min 3 times.
The dehydrated specimens were gradually infiltrated with 33% LR White resin (Electron Microscopy Sciences, Fort Washington, PA, USA) in ethanol at room temperature overnight, followed by 50% LR White resin in ethanol at room temperature for 12 h, 75% LR White resin in ethanol at room temperature overnight, and 100% LR White resin at room temperature for 12 h. After transferring the specimens to gelatin capsules, the LR White resin was allowed to polymerize at 60°C overnight.
Immunoelectron microscopy
Ultrathin sections (90–100 nm thick) were cut from the embedded blocks by an Ultracut E microtome (Reichert-Jung, Vienna, Austria). The sections were mounted on nickel grids (Nisshin EM Co., Ltd. Tokyo, Japan; 100 mesh). In some experiments, the grids were covered with a supporting film (0.67% [w/v] formvar, 67% [v/v] chloroform, and 33% [v/v] acetone) before mounting the sections. The sections were blocked by soaking in 50 µL blocking buffer containing 1% (v/v) normal goat serum (Dako, Glostrup, Denmark) and 0.1% (w/v) sodium azide in 20 mM Tris-HCl and 154 mM NaCl (pH 8.2) for 30 min at room temperature. The sections were soaked in 50 µL of antibody 42–6 (0.5–0.7 ng/mL) in blocking buffer for 2 d at 4°C. For the control, IgG from an unimmunized rabbit (Sigma-Aldrich) was used at 3.6 ng/mL instead of the antibody. Labeled sections were washed with blocking buffer for 15 min 3 times. The sections were then incubated at 35°C for 2 h with goat anti-rabbit secondary antibody conjugated to 15-nm colloidal gold (British Biocell International, Cardiff, UK) diluted 100 times in blocking buffer. The sections were washed with blocking buffer for 15 min 6 times, and then once with deionized water for 5 min. Washed sections were stained with 2% uranyl acetate for 10 min and washed thoroughly with deionized water. A JEM-1400 transmission electron microscope (JEOL, Tokyo, Japan) operated at 100 kV was used for observations.
Assay methods
Total carbohydrates, 2-keto-3-deoxysugars, and uronic acids were assayed by the phenol-sulfuric acid method [Citation17], the modified thiobarbituric acid method [Citation18], and the m-hydroxybiphenyl method [Citation19], respectively. Identification of neutral sugars were performed by the alditol acetate method as previously described [Citation2], using a Shimadzu GC-14A gas-liquid chromatograph equipped with a SP-2330 capillary column (15 m × 0.25 mm, SUPELCO). Uronic acids were identified using thin-layer chromatography. Polysaccharide samples were hydrolyzed with 2 M trifluoroacetic acid at 120°C for 1 h, evaporated under an air stream, dissolved in a small amount of water, then spotted on a silica gel plate along with standard monosaccharides. The plate was developed using a mixture of acetonitrile and water (85:15, v/v) as the mobile phase, and the sugar was detected on the plate by spraying 0.5% 1-naphthol in 5% (v/v) sulfuric acid followed by heating.
Results and discussion
Production of rabbit monoclonal antibody against B-RG-II
As a relatively small hydrophilic polysaccharide fragment, RG-II is not an efficient immunogen. Hence, we prepared a conjugate of B-RG-II with mBSA as previously described [Citation8] and immunized three rabbits with the conjugate. Lymphocytes taken from a rabbit individual whose serum showed the highest reactivity against B-RG-II were used for hybridoma production. Screening of the hybridoma pool identified at least three clones secreting B-RG-II-reactive immunoglobulins into their culture supernatants, and the one with the highest reactivity, named 42–6, was selected for the recombinant antibody production.
The reactivity of 42–6 with B-RG-II was confirmed by dot-blot analysis. As shown in , the 42–6 antibody recognized B-RG-II with a sensitivity comparable to that of a rabbit polyclonal anti-RG-II antibody [Citation14].
Figure 1. Reactivity of monoclonal antibody with borate-rhamnogalacturonan II complex (B-RG-II).
A positively charged nylon membrane was spotted with 1 µL of 20 mM Tris-HCl (pH 8.0) containing various amounts of B-RG-II in duplicate, and then subjected to chemiluminescent immunodetection with anti-RG-II antibodies. Monoclonal antibody (42–6), the recombinant rabbit monoclonal antibody produced in this study used at 1 ng/mL; polyclonal rabbit antibody, the antibody previously raised and characterized in our laboratory [Citation14] and used at 2 µg/mL; normal rabbit IgG, immunoglobulin G from unimmunized rabbit used at 2 µg/mL.
![Figure 1. Reactivity of monoclonal antibody with borate-rhamnogalacturonan II complex (B-RG-II).A positively charged nylon membrane was spotted with 1 µL of 20 mM Tris-HCl (pH 8.0) containing various amounts of B-RG-II in duplicate, and then subjected to chemiluminescent immunodetection with anti-RG-II antibodies. Monoclonal antibody (42–6), the recombinant rabbit monoclonal antibody produced in this study used at 1 ng/mL; polyclonal rabbit antibody, the antibody previously raised and characterized in our laboratory [Citation14] and used at 2 µg/mL; normal rabbit IgG, immunoglobulin G from unimmunized rabbit used at 2 µg/mL.](/cms/asset/b6864347-f671-4c41-826c-de2f9d3bded7/tbbb_a_1485479_f0001_b.gif)
We next examined the reactivity of the 42–6 antibody with several pectic polysaccharides. Since the retention of polysaccharides onto solid surfaces may be different depending on their structures, here we used a competitive ELISA for the assay. B-RG-II was covalently cross-linked via carbodiimide coupling to the plate modified with amino groups, and the antibody was then allowed to bind to the immobilized B-RG-II in the presence of competitor. Binding of the antibody in solution to the polysaccharide competitor, if any, will decrease the amount of antibody bound to the plate surface, and hence will decrease the ELISA signal. shows a representative result of the assay. Both B-RG-II and RG-II monomer not cross-linked with a borate diester linkage decreased the ELISA signal in a dose-dependent manner. This result indicated that the 42–6 antibody recognized both dimeric RG-II cross-linked with B and RG-II monomers, with slightly higher affinity to the dimer. This selectivity was similar to those observed with the rabbit polyclonal anti-RG-II antibodies previously raised in our laboratory. Neither PGA nor esterified pectin worked as effective competitors. PGA corresponds to polygalacturonic acid with a low degree of methyl esterification in their carboxylic acid groups, whereas more than 85% of carboxylic acid groups are methyl-esterified in the esterified pectin preparation used in this assay. The ELISA signal was slightly decreased in the presence of 1 mg/mL pectin. This may be due to the interaction of the antibody with the RG-II region contained within the pectin macromolecule. However, such a decrease in signal by pectin was not very reproducible; therefore, at present, we cannot exclude the possibility that the change was due to some non-specific interference of antibody binding to the plate caused by the high concentration of the polysaccharide. Another pectic polysaccharide, RG-I, also did not decrease the ELISA signal. These results suggest that the 42–6 antibody specifically recognizes RG-II.
Figure 2. Specificity of rabbit monoclonal antibody. Competitive ELISA to examine the reactivity of the 42–6 antibody against various pectic polysaccharides.
Antibody bound to plate wells was quantified using a peroxidase-conjugated secondary antibody and chemiluminescent reagent kit. Shown are representative results from at least four reproducible, independent experiments. A, comparison of the reactivity toward B-RG-II and RG-II monomer. B, examination of reactivity with various pectic polysaccharides.
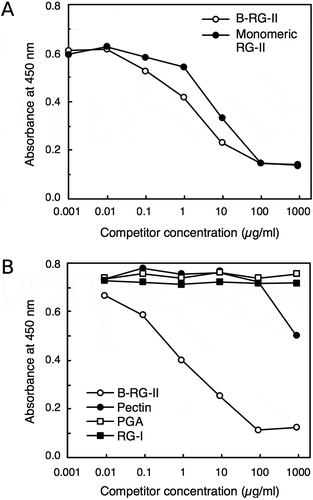
The reactivity of the 42–6 antibody was further examined against various pectic fragments generated by pectinase digestion of cell walls. shows the separation of a pectinase digest of radish cell walls using a DEAE-Sepharose column, through which B-RG-II was purified. Fractions were assayed for total sugars and 2-keto-3-deoxysugars as a diagnostic sugar of RG-II, and the fractions containing major peaks were subjected to a competitive ELISA analysis. We previously reported that separation of pectinase digest of cell walls on DEAE-Sepharose resulted in three peaks that were positive in the 2-keto-3-deoxysugar assay [Citation20]; peaks I, II, and III eluted at approximately 0.05, 0.1, and 0.15 M Cl–, respectively. Peaks II and III contained the RG-II monomer and B-RG-II, respectively. Peak I contained oligosaccharides, but its identity as the 2-keto-3-deoxysugar-containing side chains of RG-II has not been established. Then, from the chromatogram shown in , fractions 62, 72, and 83 were taken as those corresponding to peak I, II, and III, respectively. Fractions 72 and 83, respectively, containing the RG-II monomer and B-RG-II, reduced the ELISA signal as expected (). Fraction 62 did not act as a competitor, which may be because the 2-keto-3-deoxysugar-containing side chain of RG-II, if contained in the fraction, is not the epitope recognized by the 42–6 antibody. Besides these fractions, fractions 17 and 57 without detectable amounts of 2-keto-3-deoxysugars were not reactive with the 42–6 antibody. On the other hand, fraction 91, which did not contain 2-keto-3-deoxysugars, showed activity to reduce the ELISA signal. To examine the possibility that the 42–6 antibody-reactive substance is a part of RG-II devoid of 2-keto-3-deoxysugars, fraction 91 was analyzed for its glycosyl residue composition. As summarized in , the fraction contained rhamnose, xylose, fucose, and uronic acids as the major component monosaccharides. At least a part of the uronic acids was galacturonic acid, as revealed by a thin-layer chromatography analysis (). The 42–6 antibody-reactive substance was unlikely to be an oligosaccharide, because the reactivity was recovered in a high-molecular weight fraction upon fractionation on a desalting column (data not presented). RG-II contains rhamnose, fucose, and galacturonic acid, but not xylose [Citation1]. In addition, if the substance is a partially degraded RG-II lacking several residues or side chains, it should still contain apiose as the residue connecting the backbone and rhamnose- or fucose-containing side chains [Citation1]. However, apiose was not detected in fraction 91. Hence, the 42–6-reactive substance in fraction 91 was not considered to be derived from RG-II.
Table 1. Glycosyl residue composition of polysaccharide in fraction 91.
Figure 3. Examination of antibody reactivity toward pectic fragments generated by pectinase digestion of radish cell walls.
Radish cell walls were digested with pectinase and the digest was applied to a column of DEAE-Sepharose (Cl – form, 16 × 60 mm) equilibrated with column buffer (20 mM Tris-HCl, pH 8.0). After the unbound material was washed with the column buffer, the bound material was eluted using a linear gradient of 0–0.5 M NaCl (0.5 + 0.5 L) in the column buffer. Fractions were assayed for total sugars and 2-keto-3-deoxysugars to generate a chromatogram (A), and fractions containing major peaks (indicated with asterisks) were subjected to competitive ELISA analysis (B).
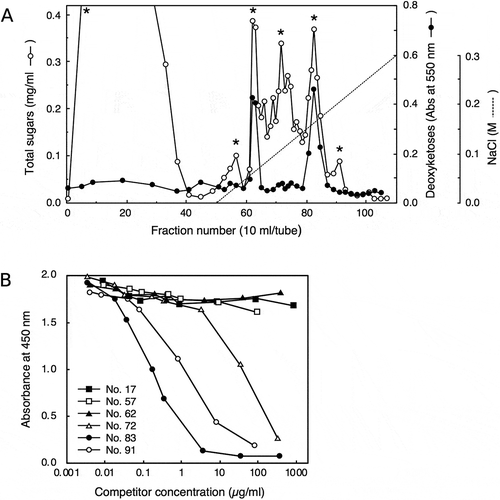
Figure 4. Thin-layer chromatography separation of acid hydrolysate of polysaccharide in fraction 91.
Lanes GalA, GlcA, Fuc, Rha, and Xyl were spotted with authentic d-galacturonic acid, d-glucuronic acid, l-fucose, l-rhamnose, and d-xylose, respectively. Lane #91 was spotted with acid hydrolysate of the polysaccharide contained in fraction 91 after separation on ion exchange column (). White and black arrowheads denote the spot of d-galacturonic acid and an unidentified substance, respectively.
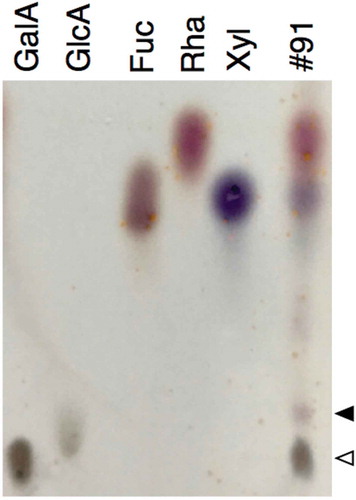
As fraction 91 was eluted immediately after B-RG-II, we cannot exclude the possibility that the B-RG-II preparation was contaminated with the 42–6 antibody-reactive substance in fraction 91. This notion raises the concern that the apparent reactivity with RG-II ( and ) might be due to the substance that peaked at fraction 91 but not to B-RG-II itself. To address the issue, we assessed whether the treatment resulting in the RG-II peak migration also induced the shift in the antibody-reactive fractions. We first performed DEAE-Sepharose column chromatography of the B-RG-II preparation and assessed the 42–6 antibody reactivity of each fraction using competitive ELISA. Peaks around fraction 43 exhibited a positive reaction with the 42–6 antibody as expected (). Next, we treated the B-RG-II preparation with 0.1 M HCl for 10 min at room temperature and separated the sample on the same DEAE-Sepharose column. The acid treatment hydrolyzed borate diester linkages between two RG-II chains, resulting in the generation of RG-II monomers that could be eluted earlier than B-RG-II [Citation20] as shown in . The newly generated peak around fraction 35 was also reactive with the 42–6 antibody (). As the acid treatment used here was mild and unlikely to break glycosidic linkages or other polysaccharide modifications including methyl-esterification or acetylation, contaminating polysaccharides in the preparation, if any, would not change its elution volume as B-RG-II did. Thus, the shift due to the 42–6 antibody reactivity observed here indicates that the 42–6 antibody indeed recognizes RG-II, even if it may not be reactive to this polysaccharide only.
Figure 5. Peak shifts for rhamnogalacturonan II (RG-II) and antibody reactivity.
Borate-RG-II complex without (a) or with (b) acid treatment was separated on a DEAE-Sepharose column (10 × 100 mm), and the fractions were subjected to total sugar analysis for RG-II and competitive ELISA for antibody reactivity. For the antibody reactivity, the decrease in absorbance at 450 nm (∆A450) caused by the addition of each fraction was plotted.
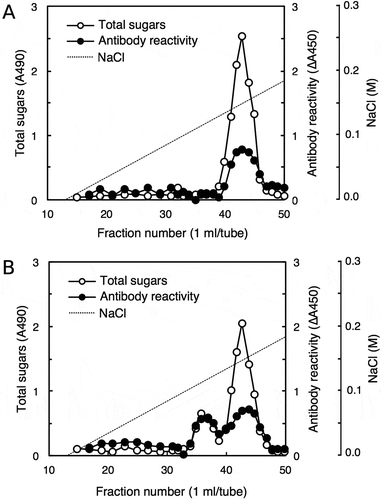
To further increase the usability of the 42–6 antibody, clarifying its epitope structure is mandatory. Therefore, it would be informative to compare the structures of RG-II and the 42–6-reactive polysaccharide in fraction 91 (). To our knowledge, the glycosyl residue composition of fraction 91 () does not coincide with that of any plant cell wall polysaccharides known so far. For example, RG-I is rich in galacturonic acid and rhamnose, but it does not contain xylose as a major constituent [Citation21]. The molecular entity of the substance in question is thus not clear at present, warranting further investigation on its origin and structure.
Immunoelectron microscopy using rabbit monoclonal anti-rg-ii antibody
Having confirmed the recognition of RG-II by the 42–6 antibody, we then proceeded to use this antibody for immunoelectron microscopy to analyze the subcellular localization of RG-II. We previously reported that the rabbit polyclonal anti-RG-II antibody bound to developing cell plates in suspension-cultured tobacco cells, suggesting that the RG-II domain of pectin was already present at an early stage of cell wall assembly [Citation14]. Then, we investigated whether similar results could be obtained with our new rabbit monoclonal antibody, using root tips of Arabidopsis seedlings as the plant material.
Ultrathin sections were excised from Arabidopsis roots that were fixed using a high pressure frozen/freeze substitution technique, probed with the 42–6 antibody, and observed under a transmission electron microscope. Gold-conjugated secondary antibodies were used to visualize the antibodies bound to the section. shows the cells in root tip of Arabidopsis seedlings. Observed in the central part of is a cell undergoing cytokinesis. Treated with the 42–6 antibody, gold particles were found in cell walls as shown in , whereas such a signal was not detectable in sections treated with preimmune serum (). The labeling with rabbit polyclonal anti-RG-II antibody was previously reported to be found preferentially at the membrane-proximal region of the cell wall [Citation8], whereas such a gradient distribution of signals across the wall was not obvious with the 42–6 antibody (). On the other hand, the average densities of labeling found in longitudinal walls were significantly higher (p < 0.01) than that in transverse walls (). The difference suggests the presence of heterogeneity in the cell wall microstructure within a single cell. Alternatively, the density of RG-II in longitudinal walls may be transiently higher while the cells are young and longitudinally short, but it may decrease to a similar level to that in transverse walls as the cells elongate. Further analysis of labeling density in the walls of elongated cells is necessary.
Figure 6. Immunogold labeling of rhamnogalacturonan II in dividing cells in Arabidopsis roots.
(a–c) Section incubated with the 42–6 primary and gold-conjugated secondary antibodies. (d) Section incubated with normal rabbit immunoglobulin and gold-conjugated secondary antibody. (b) and (c) are higher magnifications of squares drawn in the left and middle part in (a), respectively. Arrows in (b) and (c) show representative gold particles. Larger and less defined dots are ribosomes. A line in the upper-right corner in (a), as indicated by the asterisk, is an artificial scratch generated during the section preparation. cp, cell plates; cw, cell walls. Bar = 1 μm.
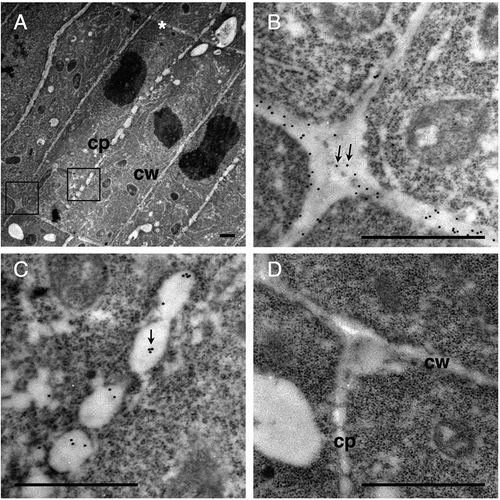
Figure 7. Quantification of the 42–6 antibody immunogold labeling density.
Boxplot representing the number of gold particles per square micrometer after immunogold labeling of rhamnogalacturonan II in longitudinal and transverse cell walls. Gold particles were counted in 20 micrograph images for each direction and divided by the area measured using the ImageJ software [Citation23]. The micrographs used represent 17 independent cells from three seedlings. Box encompasses the 25–75 percentiles with the solid line indicating the median. Whiskers show the 10–90 percentiles.
![Figure 7. Quantification of the 42–6 antibody immunogold labeling density.Boxplot representing the number of gold particles per square micrometer after immunogold labeling of rhamnogalacturonan II in longitudinal and transverse cell walls. Gold particles were counted in 20 micrograph images for each direction and divided by the area measured using the ImageJ software [Citation23]. The micrographs used represent 17 independent cells from three seedlings. Box encompasses the 25–75 percentiles with the solid line indicating the median. Whiskers show the 10–90 percentiles.](/cms/asset/5b5450fc-4aa3-4dee-a484-0b89f49cdd23/tbbb_a_1485479_f0007_b.gif)
The cell in has electron-lucent luminal structures aligned between two daughter nuclei. The structures are considered to be the cell plate at the fenestrated sheet stage, which corresponds to the later phase of cell plate formation [Citation22]. In the sections treated with the 42–6 antibody, gold particles were found in these structures (), whereas such labeling was not observed in the section treated with preimmune serum (). The result indicate that RG-II is present in forming cell plate.
The labeling with the 42–6 antibody was also observed in cell plates at an earlier developmental stage. In , a developing cell plate could be observed in the center of the cell, with a cluster of vesicle-like structures at the edge. The edge was still distant from the walls of parental cells (). Based on these morphologies, the cell plate was considered at the tubular network stage. Gold labeling was found on this cell plate in both the vesicular structure in the edge region and the central luminal structures (). These results together suggest that RG-II appears in the cell plate by the tubular network stage, which corresponds to mid-telophase of cytokinesis [Citation22]. The assumption is consistent with that from our previous study using rabbit polyclonal anti-RG-II antibody and suspension-cultured tobacco cells [Citation14]. Obtaining consistent results with independent antibodies and plant materials further support the presence of RG-II in the developing cell plates of dividing cells, suggesting its role in the organization of the nascent cell wall as the cross-linker of matrix polysaccharides.
Figure 8. Immunogold labeling of rhamnogalacturonan II in root cells at an earlier stage of cell division.
(a) A dividing cell with a developing cell plate, which is presumably at the tubular network stage (white arrowhead). (b) Higher magnifications of the square in (a). Arrows in (b) show representative gold particles. cp, cell plate. Bar = 1 μm.
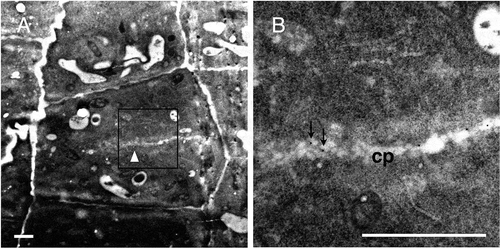
Conclusion
In this study, we describe the preparation of 42–6, a new anti-RG-II rabbit monoclonal antibody. Although the antibody also recognized a polysaccharide with yet unknown structure and its specificity still needs to be established, it can expand the variety of anti-RG-II antibody available for high sensitivity detection or immunocytochemistry. We further used the antibody to demonstrate RG-II in dividing cells in Arabidopsis root tips, then obtained results confirming the presence of RG-II in the forming cell plates.
Author contribution
Y.Z., M.K., and T.M. designed the study. Y.Z., T.A., and M.K. conducted the experiments. Y.Z., M.K., and T.M. wrote the manuscript. T.A. and K.T. contributed to data collection and interpretation, and critically reviewed the manuscript. The final version of the manuscript was approved by all authors.
Acknowledgments
We thank Drs. Takehiro Masumura and Shigeto Morita at Kyoto Prefectural University for the use of the high pressure freezer.
Disclosure statement
No potential conflict of interest was reported by the authors.
Additional information
Funding
References
- O’Neill MA, Ishii T, Albersheim P, et al. Rhamnogalacturonan II: structure and function of a borate cross-linked cell wall pectic polysaccharide. Annu Rev Plant Biol. 2004;55:109–139.
- Kobayashi M, Matoh T, Azuma J. Two chains of rhamnogalacturonan II are cross-linked by borate-diol ester bonds in higher plant cell walls. Plant Physiol. 1996;110:1017–1020.
- Kobayashi M, Nakagawa H, Asaka T, et al. Borate-rhamnogalacturonan II bonding reinforced by Ca2+ retains pectic polysaccharides in higher-plant cell walls. Plant Physiol. 1999;119:199–204.
- Ishii T, Matsunaga T, Hayashi N. Formation of rhamnogalacturonan II-borate dimer in pectin determines cell wall thickness of pumpkin tissue. Plant Physiol. 2001;126:1698–1705.
- Matoh T, Takasaki M, Kobayashi M, et al. Boron nutrition of cultured tobacco BY-2 cells. III. Characterization of the boron-rhamnogalacturonan II complex in cells acclimated to low levels of boron. Plant Cell Physiol. 2000;41:363–366.
- Ryden P, Sugimoto-Shirasu K, Smith AC, et al. Tensile properties of Arabidopsis cell walls depend on both a xyloglucan cross-linked microfibrillar network and rhamnogalacturonan II-borate complexes. Plant Physiol. 2003;132:1033–1040.
- Williams MN, Freshour G, Darvill AG, et al. An antibody Fab selected from a recombinant phage display library detects deesterified pectic polysaccharide rhamnogalacturonan II in plant cells. Plant Cell. 1996;8:673–685.
- Matoh T, Takasaki M, Takabe K, et al. Immunocytochemistry of rhamnogalacturonan II in cell walls of higher plants. Plant Cell Physiol. 1998;39:483–491.
- Baluska F, Hlavacka A, Samaj J, et al. F-actin-dependent endocytosis of cell wall pectins in meristematic root cells. Insights from brefeldin A-induced compartments. Plant Physiol. 2002;130:422–431.
- Iwai H, Hokura A, Oishi M, et al. The gene responsible for borate cross-linking of pectin Rhamnogalacturonan-II is required for plant reproductive tissue development and fertilization. Proc Natl Acad Sci USA. 2006;103:16592–16597.
- Kasprowicz A, Szuba A, Volkmann D, et al. Nitric oxide modulates dynamic actin cytoskeleton and vesicle trafficking in a cell type-specific manner in root apices. J Exp Bot. 2009;60:1605–1617.
- Bárány I, Fadón B, Risueño MC, et al. Cell wall components and pectin esterification levels as markers of proliferation and differentiation events during pollen development and pollen embryogenesis in Capsicum annuum L. J Exp Bot. 2010;61:1159–1175.
- Shi D-C, Wang J, Hu R-B, et al. and calcium are required to maintain the pectin network of the Arabidopsis seed mucilage ultrastructure. Plant Mol Biol. 2017;94:267–280.
- Zhou Y, Awano T, Kobayashi M, et al. Immunocytochemical detection of rhamnogalacturonan II on forming cell plates in cultured tobacco cells. Biosci Biotech Biochem. 2017;81:899–905.
- Gray BM. ELISA methodology for polysaccharide antigens: protein coupling of polysaccharides for adsorption to plastic tubes. J Immunol Methods. 1979;28:187–192.
- Darvill AG, McNeil M, Albersheim P. Structure of plant cell walls: VIII. A new pectic polysaccharide. Plant Physiol. 1978;62:418–422.
- Dubois M, Gilles KA, Hamilton JK, et al. Colorimetric Method for Determination of Sugars and Related Substances. Anal Chem. 1956;28:350–356.
- York WS, Darvill AG, McNeil M, et al. 3-Deoxy-d-manno-2-octulosonic acid (KDO) is a component of rhamnogalacturonan II, a pectic polysaccharide in the primary cell walls of plants. Carbohydr Res. 1985;138:109–126.
- Blumenkrantz N, Asboe-Hansen G. New method for quantitative determination of uronic acids. Anal Biochem. 1973;54:484–489.
- Kobayashi M, Ohno K, Matoh T. Boron nutrition of cultured tobacco BY-2 cells. II. Characterization of the boron-polysaccharide complex. Plant Cell Physiol. 1997;38:676–683.
- Ridley BL. Pectins: structure, biosynthesis, and oligogalacturonide-related signaling. Phytochemistry. 2001;57:929–967.
- Samuels AL, Giddings TH, Staehelin LA. Cytokinesis in tobacco BY-2 and root tip cells: a new model of cell plate formation in higher plants. J Cell Biol. 1995;130:1345–1357.
- Schneider CA, Rasband WS, Eliceiri KW. NIH Image to ImageJ: 25 years of image analysis. Nature Methods. 2012;9:671–675.