ABSTRACT
We overexpressed and purified 3α-hydroxysteroid dehydrogenase from Pseudomonas sp. B-0831 (Ps3αHSD) and its mutants where the active site residues known as the SYK triad, Ser114, Tyr153, and Lys157, were mutated. Ps3αHSD catalyzes the reaction by using a nucleotide cofactor. The NADH binding affinity of K157A mutant was much lower than that of the wild-type, mainly due to loss of a hydrogen bond. The decreased affinity would result in decreased kcat. Compared to the wild-type, the mutants S114A and Y153F showed higher Km and lower kcat values in both oxidation and reduction reactions. Simultaneous mutation of S114A and Y153F resulted in a significant decrease in kcat relative to the single mutant. These results are supported by the notion that Tyr153 is a catalytic base and Ser114 would be a substitute. Loss of hydrogen bonding with NADH upon the Y153F mutation resulted in increased enthalpy change, partially compensated by increased entropy change.
Graphical Abstract
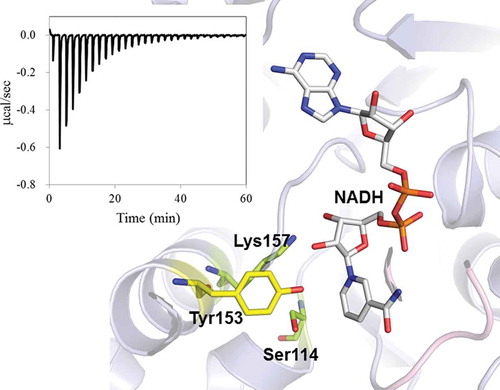
Crystal structure of Ps3aHSD and ITC profile for NADH binding. The effects of mutations on catalysis and cofactor binding are well correlated with the structural information.
3α-Hydroxysteroid dehydrogenase (3αHSD) catalyzes the reversible inter-conversion of hydroxy and oxo groups at position 3 of the steroid nucleus by using either NAD(H) or NADP(H) as a cofactor. Despite similar substrate specificities, eukaryotic and prokaryotic 3αHSDs belong to two different protein superfamilies, the aldo-keto reductase and the short-chain dehydrogenase/reductase (SDR) superfamilies [Citation1,Citation2]. The 3αHSD from Pseudomonas sp. B-0831 (Ps3αHSD) forms a dimer composed of two 26 kDa subunits and can also catalyze the carbonyl reduction of a variety of non-steroidal aldehydes [Citation3–Citation5]. This enzyme has been used as a diagnostics for measuring total bile acids in serum using the enzymatic cycling reaction when coupled with thio-NAD+ and NADH [Citation6]. Common to the SDR family enzymes, Ps3αHSD has the Ser-Tyr-Lys triad (SYK triad) as an active site, and the cofactor-binding motif, GXXXGXG, at the N-terminal region. We previously determined the X-ray crystal structure of Ps3αHSD in the presence of NADH, showing a structural heterodimer composed of apo – and holo-subunits [Citation7,Citation8]. Together with the structural analysis of Ps3αHSD in solution using circular dichroism (CD), we revealed that NADH binding could induce helix formation in the substrate-binding loop, and that there would be negative cooperativity in NADH binding to the two subunits of Ps3αHSD [Citation8]. The results strongly indicate that cofactor binding could regulate the enzymatic function, resulting from the fine-tuned conformational changes.
In order to obtain site-specific information on the structural and functional properties of Ps3αHSD, we first generated an expression system for Ps3αHSD with N-terminal His-tag in Escherichia coli (E. coli). In this study, we analyzed the role of the active site residues in the function and cofactor binding of Ps3αHSD using the mutants, S114A, Y153F, K157A, and S114A/Y153F. NADH binding to the recombinant Ps3αHSD and its mutants was thermodynamically analyzed using isothermal titration calorimetry (ITC). The ITC experiments could determine the accurate binding affinity of biomolecular interactions in solution with thermodynamic parameters such as enthalpy (H) and entropy (S) changes [Citation9,Citation10]. The results could be discussed in the context of the structural information on Ps3αHSD [Citation8], and compared with those of the related enzyme, 3αHSD from Comamonas testosteroni (Ct3αHSD) [Citation11–Citation14].
Materials and methods
Expression and purification of ps3αhsd and its mutants
A DNA fragment encompassing wild-type Ps3αHSD are amplified by PCR, using Ps3αHSD cDNA plasmid as the template and two synthetic primers () to generate a NdeI and a EcoRI site at the 5ʹ – and 3ʹ-end of the amplified fragment, respectively. After digestion with NdeI and EcoRI, the DNA fragments were cloned into pET28a(+) plasmid (Novagen). The mutant plasmids of S114A, Y153F, K157A, and S114A/Y153F were constructed by site-directed mutagenesis using two synthetic mutagenesis primers (). E. coli BL21 (DE3) cells were transformed with the plasmids of wild-type Ps3αHSD and its mutants. Freshly pre-cultivated cells were inoculated into growth medium containing 30 μg mL–1 kanamycin and growth at 37ºC. When the culture reached an A600 of 0.6, isopropyl-1-thio-β-D-galactopyranoside was added to a final concentration of 0.5 mM. The cells were incubated at 22ºC for another 20 hours. Wild-type Ps3αHSD and its mutants were expressed as 6× His fusion proteins. The harvest cells were suspended in 20 mM Tris-HCl (pH 7.5) containing 100 mM NaCl (buffer A) and lysed by sonication at 4ºC. After the cell debris was removed by centrifugation, the supernatant was loaded to a nickel-nitrilotriacetic acid column (Ni-NTA Agarose, QIAGEN). The column was washed using buffer A containing 80 mM imidazole, and the protein was eluted with buffer A containing 400 mM imidazole. The purified proteins were dialyzed against 20 mM Tris-HCl buffer (pH 8.0) containing 100 mM NaCl. The protein concentration was determined at an OD of 280 nm and was calculated using a molar absorption coefficient of 1.4 × 104 M–1 cm–1 for the wild-type, S114A, and K157A [Citation7] and that of 1.2 × 104 M–1 cm–1 for Y153F and S114A/Y153F.
Table 1. The primer sequences for Ps3aHSD using PCR.
Circular dichroism (CD) measurements
Far-UV (200 – 250 nm) CD spectra were measured on a Jasco J-725 or J-820 spectropolarimeter at 20ºC equipped with Peltier-type temperature control system. The spectra were obtained for the protein concentration, 0.1 mg mL–1, in 20 mM Tris-HCl buffer (pH 8.0) containing 100 mM NaCl, using quartz cell with 0.2 cm path-length. CD spectra were obtained using scanning speed of 20 nm min–1, a time response of 1 sec, a bandwidth of 1 nm, and an average over 4 scans.
Catalytic activity measurements
The assays were carried out at 37°C in 0.5 mL solution containing 40 mM Tris-HCl (pH 8.0), in the presence of 1 mM NAD+, 0.025% nitrotetrazolium blue, 0.4% Triton X-100, and 2.5 units mL–1 diaphorase for oxidation reaction, and in the presence of 0.13 mM NADH for reduction reaction [Citation5]. Various concentrations of substrates, cholic acid and dehydrocholic acid, were used for the determination of Km and kcat (Table S1). The oxidation reaction was initiated by adding 20 μL of the enzyme and 25 μL of cholic acid, and terminated by adding 125 μL of 10% SDS after incubation for 5 min. The Km and kcat values for oxidation reaction were determined by measuring the formation of formazan dye at 550 nm, and those for reduction reaction were determined by measuring the decrease of NADH at 340 nm, followed by analyzing using Lineweaver-Burk plot.
ITC measurements
ITC experiments were carried out at 25°C using iTC200 (Malvern Panalytical). All samples were in 20 mM Tris-HCl buffer (pH 8.0) containing 100 mM NaCl. The solution of NADH was titrated into the solution of wild-type Ps3αHSD or its mutants. Each corrected heat was divided by the moles of NADH injected and was analyzed using the Origin software supplied by Malvern Panalytical.
Results
Wild-type Ps3αHSD and its mutants, S114A, Y153F, K157A, and S114A/Y153F, with an N-terminal His-tag, were overexpressed in E. coli, and purified using a Ni-NTA column. Purification yielded proteins with more than 95% purity, as estimated by SDS-PAGE analysis (data not shown). The far-UV CD spectra of the purified proteins indicate that they folded correctly and that their secondary structures were similar (). These spectra were also similar to that of Ps3αHSD without the His-tag [Citation8], indicating that the N-terminal His-tag had little effect on their structure.
Figure 1. Secondary structure analysis. Far-UV CD spectra of wild-type Ps3αHSD and its mutants, S114A, Y153F, K157A, and S114A/Y153F, are superimposed.
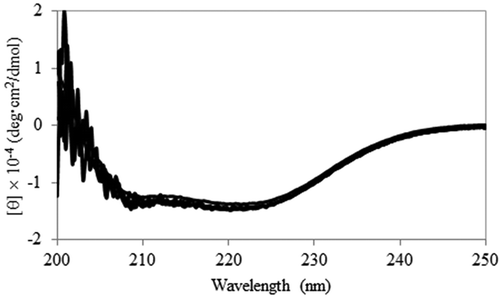
The enzymatic activities of the oxidation or reduction reactions were analyzed using cholic acid and dehydrocholic acid as substrates, respectively, and the results are summarized in . The activity with the His-tag was comparable to that reported previously for Ps3αHSD without the His-tag [Citation5], indicating that the N-terminal His-tag had little effect on their function. The mutations of S114A and Y153F resulted in increased Km and decreased kcat in both oxidation and reduction reactions. Simultaneous mutation of S114A and Y153F resulted in a significant decrease in kcat relative to the single mutant. Mutation of K157A resulted in a decrease in kcat with little change in Km.
Table 2. Steady-state kinetic constants for Ps3αHSD and its mutants with steroids.
Cofactor binding was analyzed using ITC. Because of weak binding affinity [Citation5], the binding thermodynamics of NAD+ were difficult to be determined by the conventional method of NAD+ injection into the protein. shows the ITC profiles of NADH binding to wild-type Ps3αHSD and its mutants. An exothermic heat pulse was observed after each injection of NADH into the wild-type, S114A, Y153F, and S114A/Y153F proteins. Probably because of low affinity with small ΔH, little heat was observed in K157A. The binding thermodynamics of the wild-type, S114A, Y153F, and S114A/Y153F are summarized in . In the case of Y153F, because of its low binding affinity, the binding thermodynamics were determined from the fitting when the binding stoichiometry toward the monomer unit was fixed as 1. The binding stoichiometry of the wild-type, S114A, and S114A/Y153F proteins was determined to be 1.0 ± 0.2 by the fitting procedure. Probably because of the detection limit with ITC of determining the presence or absence of negative cooperativity in NADH binding to the two subunits of Ps3αHSD, the fitting procedure was carried out on the one-site binding model. The Kd value of the wild-type, 15 μM, was comparable to that of Ps3αHSD without the His-tag obtained from fluorescence titration experiments as 7.6 μM [Citation5]. The binding affinity of S114A was about 3-times higher than that of the wild-type, and that of Y153F was about 4-times lower than that of the wild-type, both of which were mainly due to the change in ΔH.
Table 3. Thermodynamic parameters for interactions of NADH with Ps3αHSD and its mutants.
Discussion
The recombinant protein, Ps3αHSD, with an N-terminal His-tag was overexpressed and purified, making it possible to analyze the role of each residue in its structure and function. In this study, we analyzed the contribution of catalytic residues, Ser114, Tyr153, and Lys157, known as the SYK triad to catalysis and NADH binding. Previous studies using Ct3αHSD have shown that the Ser residue corresponding to Ser114 of Ps3αHSD acts to stabilize the substrate and the substrate-binding loop, the Tyr residue corresponding to Tyr153 is a catalytic base, and the Lys residue corresponding to Lys157 acts in forming hydrogen-bonds with the cofactor [Citation12,Citation14]. shows the relative positions of these residues and NADH in the crystal structure of Ps3αHSD [Citation8]. Similar to Ct3αHSD, Tyr153 and Lys157 are hydrogen-bonded to the nicotinamide ribose moiety of NADH. Upon mutation of Y153F, loss of hydrogen bond resulted in increased ΔH, partially compensated by increased ΔS. The ITC results also indicate that the contribution of Lys157 to cofactor binding is greater than that of Tyr153, because the heat generated from NADH binding to the K157A mutant, which is closely correlated with ΔH, was very small (). Little change in Km upon the K157A mutation also indicates that substrate binding is little perturbed by the mutation. Taken together, Lys157 could be critical for cofactor binding, which results in a conformational change in Ps3αHSD to accelerate catalysis [Citation8]. Upon the mutation of Y153F, Ser114 could act as a substitute for the catalytic base, similar to Ct3αHSD [Citation12]. This is supported by the significant loss of kcat upon the simultaneous mutation of S114A/Y153F.
Figure 3. Relative positions of catalytic residues, Ser114, Tyr153, and Lys157, and NADH in the crystal structure of Ps3αHSD [Citation8]. The distances are indicated as the unit of Å. The image was generated using the program PyMol (http://www.pymol.org/).
![Figure 3. Relative positions of catalytic residues, Ser114, Tyr153, and Lys157, and NADH in the crystal structure of Ps3αHSD [Citation8]. The distances are indicated as the unit of Å. The image was generated using the program PyMol (http://www.pymol.org/).](/cms/asset/6cf0d801-d789-4033-b523-4b550c1b4666/tbbb_a_1486175_f0003_oc.jpg)
The kcat values for oxidation reactions by the mutants, S114A, Y153F, K157A, and S114A/Y153F, were decreased by 29-, 37-, 511-, and 1,917-fold, respectively. Compared with the mutation effects on the kcat values of Ct3αHSD [Citation12], the degree of decrease was found to be smaller. The kcat/Km values for oxidation reactions by the wild-type, S114A, Y153F, K157A, and S114A/Y153F were determined to be 4.2 × 105, 1.5 × 103, 1.3 × 103, 4.8 × 102, and 1.6 × 102 M–1 sec–1, respectively. Taken together, the present results showed that the role of catalytic residues in Ps3αHSD is similar to those in Ct3αHSD, but their respective contribution to catalytic efficiency is different. Ps3αHSD can use NADP(H) as well as NAD(H) as a cofactor, which is different from Ct3αHSD. In addition, we found the unique feature that there is a negative cooperativity in the NADH binding to Ps3αHSD [Citation8]. Cofactor binding could regulate the fine-tuned function of Ps3αHSD, resulting from the conformational change. The structure-function relationship of Ps3αHSD in comparison with that of Ct3αHSD will help to understand the precise mechanism of enzyme function.
In this study, we overexpressed and purified recombinant Ps3αHSD with an N-terminal His-tag in E. coli, and evaluated the role of catalytic residues in its catalysis and cofactor binding. NADH binding was analyzed using ITC, and binding thermodynamics including binding affinity were determined. The effects of mutations could be well correlated with the structural information determined previously. Further analysis is now in progress, especially concerning the structural mechanism of conformational change induced upon cofactor binding and its negative cooperativity using site-specific mutants of Ps3αHSD.
Author contributions
MO designed the research, AS prepared samples and carried out CD and catalytic activity measurements, AS and SI carried out ITC, MO wrote the paper.
Supplemental Material
Download PDF (13.1 KB)Acknowledgments
The original cDNA plasmid encoding Ps3αHSD was provided from Dr. Shigeru Ueda of Asahi Kasei Pharma Corporation. The authors thank Mr. Hidekazu Kaminaka of Kyoto Prefectural University for plasmid preparation and Mr. Takahiro Maruno of Osaka University for ITC measurements. The CD experiments were performed in part at Graduate School of Life and Environmental Sciences, Osaka Prefecture University; MO is a visiting researcher.
Disclosure statement
No potential conflict of interest was reported by the authors.
References
- Jez JM, Bennett MJ, Schlegel BP, et al. Jez JM, Bennett MJ, Schlegel BP, Lewis M, Penning TM. Comparative anatomy of the aldo-keto reductase superfamily. Biochem. J.1997;326 (Pt 3):625–636.
- Kallberg Y, Oppermann U, Jörnvall H, et al. Short-chain dehydrogenases/reductases (SDRs). Eur. J. Biochem.2002;269:4409–4417.
- Ueda S, Oda M, Imamura S, et al. Steady-state kinetic properties of 3α-hydroxysteroid dehydrogenase from Pseudomonas sp. B-0831: steroid substrate specificity and nucleotide cofactor dependency. J. Biol. Macromol..2004;4:23–28.
- Ueda S, Oda M, Imamura S, et al. Carbonyl reductase activity of a pluripotent enzyme, 3α-hydroxysteroid dehydrogenase from Pseudomonas sp. B-0831. J. Biol. Macromol.2004;4:29–32.
- Ueda S, Oda M, Imamura S, et al. Transient-phase kinetic studies on the nucleotide binding to 3α-hydroxysteroid dehydrogenase from Pseudomonas sp. B-0831 using fluorescence stopped-flow procedures. Eur. J. Biochem. 2004;271:1774–1780.
- Ueda S, Oda M, Imamura S, et al. Kinetic study of the enzymatic cycling reaction conducted with 3α-hydroxysteroid dehydrogenase in the presence of excessive thio-NAD+ and NADH. Anal. Biochem.2004;332:84–89.
- Kataoka S, Nakamura S, Ohkubo T, et al. Crystallization and preliminary X-ray analysis of the complex of NADH and 3α-hydroxysteroid dehydrogenase from Pseudomonas sp. B-0831. Acta Crystallogr. F. 2006;62:569–571.
- Nakamura S, Oda M, Kataoka S, et al. Apo- and holo-structures of 3α-hydroxysteroid dehydrogenase from Pseudomonas sp. B-0831. Loop-helix transition induced by coenzyme binding. J. Biol. Chem. 2006;281:31876–31884.
- Inaba S, Numoto N, Ogawa S, et al. Crystal structures and thermodynamic analysis reveal distinct mechanisms of CD28 phosphopeptide binding to the Src homology 2 (SH2) domains of three adaptor proteins. J. Biol. Chem.2017;292:1052–1060.
- Oda M, Inaba S, Kamiya N, et al. Structural and thermodynamic characterization of endo-1,3-β-glucanase: insights into the substrate recognition mechanism. BBA - Proteins and Proteomics. 2018;1866:415–425.
- Grimm C, Maser E, Möbus E, et al. The crystal structure of 3α-hydroxysteroid dehydrogenase/carbonyl reductase from Comamonas testosteroni shows a novel oligomerization pattern within the short chain dehydrogenase/reductase family. J. Biol. Chem. 2000;275:41333–41339.
- Hwang CC, Chang YH, Hsu CN, et al. Mechanistic roles of ser-114, tyr-155, and lys-159 in 3α-hydroxysteroid dehydrogenase/carbonyl reductase from comamonas testosteroni. J. Biol. Chem. 2005;280:3522–3528.
- Chang YH, Huang TJ, Chuang LY, et al. Role of S114 in the NADH-induced conformational change and catalysis of 3α-hydroxysteroid dehydrogenase/carbonyl reductase from Comamonas testosteroni. Biochim. Biophys. Acta. 2009;1794:1459–1466.
- Chang YH, Wang CZ, Chiu CC, et al. Contributions of active site residues to cofactor binding and catalysis of 3α-hydroxysteroid dehydrogenase/carbonyl reductase. Biochim. Biophys. Acta. 2010;1804:235–241.