ABSTRACT
The RNA decapping enzyme Dcp2 is a crucial enzyme involved in the process of RNA turnover, which can post-transcriptionally regulate gene expression. Dcp2 has been found to be highly expressed in embryonic, but not adult, kidneys. Here we showed that Dcp2 mRNA was expressed, but Dcp2 proteins were absent, in mouse kidneys after postnatal day 10 (P10). In kidneys of adult Dcp2-IRES-EGFP knock-in mice, Dcp2 was undetectable but EGFP was expressed, indicating that Dcp2 mRNA was not completely silenced in adult kidneys. Using luciferase reporter assays, we found that miR-141-3p/200a-3p directly targeted the 3ʹ UTR of Dcp2 mRNA. Overexpression of miR-141-3p and miR-200a-3p downregulated endogenous Dcp2 protein expression. Furthermore, miR-141-3p and miR-200a-3p expression was low in embryonic kidneys but increased dramatically after P10 and was negatively correlated with Dcp2 protein expression during renal development. These results suggest miR-141-3p/200a-3p may be involved in post-transcriptional repression of Dcp2 expression during renal development.
Abbreviations: IRES: internal ribosome entry site; EGFP: enhanced green fluorescent protein; UTR: untranslated region
GRAPHICAL ABSTRACT
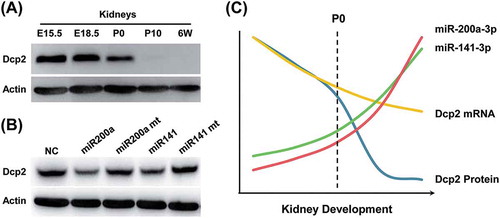
MicroRNA-141-3p/200a-3p target RNA decapping enzyme Dcp2. Their expression is negatively correlated with Dcp2 protein during mouse renal development.
The mammalian kidney is a complex organ in which more than 30 types of cells have been identified. Kidney development is a complicated differentiation process involving a series of interactions between the ureteric bud (UB) and the metanephric mesenchyme (MM). Reciprocal inductive interactions between the UB and the MM play a central role in initiating and maintaining cycles of UB branching [Citation1]. During UB branching, a subtype of MM cells around the UB tip condenses to form the cap mesenchyme, which provides a source of nephron progenitor cells that proliferate and differentiate into glomerular and tubular epithelial cells until postnatal day 3 [Citation2]. During kidney differentiation, gene expression is precisely controlled at specific levels, forming a complex signaling network [Citation3–Citation5].
MicroRNAs (miRNAs) are involved in the post-transcriptional regulation of gene expression during renal development. Deletion of Dicer, which is important for primary miRNA processing, in nephron progenitor cells leads to increased apoptosis and premature termination of nephrogenesis. Knockout of Dicer in the UB epithelium results in the development of renal cysts [Citation6] and inactivation of Dicer1 in kidney stromal cells causes renal hypoplasia [Citation7]. MiRNAs post-transcriptionally regulate gene expression by destabilizing mRNAs and/or repressing translation, especially cap-dependent, but not internal ribosome entry site (IRES)-mediated, translation [Citation8].
The regulation of mRNA degradation is a key step in the control of gene expression. The RNA decapping enzyme Dcp2 is the key component of the RNA decay machinery, which also regulates gene expression at the post-transcriptional level. In higher eukaryotic cells, RNA decay is usually initiated by the removal of the 3ʹ poly A tail by deadenylases. RNA can then be degraded by 5ʹ to 3ʹ and 3ʹ to 5ʹ pathways. In the 5ʹ to 3ʹ degradation pathway, Dcp2 and Nudt16 cleave the 7-monomethyl guanosine (m7G) cap [Citation9,Citation10]. The remaining RNA can then be hydrolyzed by the exoribonuclease Xrn1 [Citation11]. Several proteins have been shown to bind and enhance the decapping activity of Dcp2, including Dcp1a, Edc3 and Edc4 (also known as Hedls) [Citation12,Citation13]. Dcp2 is expressed in embryonic kidney cells but its expression decreases after birth and disappears in adult kidneys [Citation9], suggesting that it may play a role in renal development.
Dcp2 preferentially removes the cap structure of a subset of mRNAs that contain a stem-loop structure in the 5ʹ untranslated region (UTR) [Citation14,Citation15] or a uridine-tract at the 3ʹ end [Citation16,Citation17], whereas mRNAs with an m7G cap followed by an m6Am or m6A are decapped significantly less often [Citation18]. Given that Dcp2 is highly expressed in embryonic kidney cells, these findings suggest that Dcp2 may post-transcriptionally repress the expression of selected differentiation-related genes in the embryonic kidney. Furthermore, the lack of Dcp2 proteins in adult kidneys may be a prerequisite for the expression of specific genes in differentiated cells. However, to date, little is known about the mechanism of Dcp2 silencing in adult kidneys.
In this study, we found that a substantial amount of Dcp2 mRNA was expressed in kidneys of mice after P10. Using Dcp2-IRES-EGFP knock-in mice, we confirmed that Dcp2 mRNA was not completely silenced in adult kidneys. Further investigation showed that miR-141-3p and miR-200a-3p, directly targeted Dcp2 and that their expression was negatively correlated with Dcp2 protein expression during renal development. Therefore, miR-141-3p and miR-200a-3p may post-transcriptionally inhibit Dcp2 expression during the late stages of kidney development.
Materials and methods
Animal experiments
Wild type C57BL/6 mice were purchased from Shanghai SLAC Laboratory Animal Co. Ltd. (Shanghai, China). Developmental stages of embryos were determined based on the presence of a vaginal plug. Dcp2-IRES-EGFP knock-in mice were generated by Beijing Biocytogen Co., Ltd. (Beijing, China) using clustered regularly interspaced short palindromic repeats (CRISPR)/Cas9. Genotypes of mice were determined using polymerase chain reaction (PCR) with the primers mDcp2-1275F, EGFP-F2 and mDcp2-1469R (). All animal experiments were performed according to the guidelines of the Care and Use of Laboratory Animals of the Laboratory Animal Ethical Commission of Fudan University, China and were approved by the Animal Ethical Committee of Zhongshan Hospital, Fudan University, China.
Table 1. Primers used for qPCR and plasmids construction.
Cell culture
HEK293T and NIH3T3 embryonic fibroblast cells were grown in Dulbecco’s modified Eagle’s medium containing 10% fetal bovine serum (Wisent, St-Bruno, QC, Canada) in a humidified atmosphere of 5% CO2 at 37°C.
Western blot
Tissues were homogenized using a Precellys®24 homogenizer (Bertin, Montigny-le-Bretonneux, France) in cold lysis buffer (phosphate-buffered saline containg 0.25% TritonX-100) with proteinase inhibitor cocktail (Biotools, Loganholme, Australia). Lysates were centrifuged at 15,000 × g for 10 min at 4°C and supernatants were transferred to new tubes. Glycerol was added to a final concentration of 10%. NIH3T3 cells were also lysed with lysis buffer. A total of 20 μg cellular protein or 40 μg tissue protein were separated by sodium dodecyl sulfate- 10% polyacrylamide gel electrophoresis and transferred to a nitrocellulose membrane (Millipore, Tullagreen, Ireland). The following primary antibodies were used: rabbit anti-Dcp2 antibody (gift from Megerditch Kiledjian, Rutgers University, USA), rabbit anti-GFP antibody (1:4000; Cat. No. 50,430–2-AP, Proteintech, Rosemont, IL, USA) and mouse anti-β-actin antibody (1:5000; Cat. No. 60,008–1-Ig, Proteintech). After extensive washing, membranes were incubated for 1 h at room temperature with the corresponding horseradish peroxidase-conjugated secondary antibodies (Yeasen, Shanghai, China). Immunoreactive proteins were visualized using ECL reagent (Yeasen) and quantified using ImageJ software (https://imagej.nih.gov/ij/).
RNA isolation, reverse transcription and real-time quantitative PCR
Kidney tissue was homogenized in Tripure Isolation Reagent (Roche, Mannheim, Germany) and total RNA was isolated following the manufacturer’s instructions. For mRNA real-time quantitative PCR (qPCR), 1 μg total RNA was reverse transcribed into cDNA using HiScript II Q RT SuperMix for qPCR (Vazyme Biotech Co., Ltd., Nanjing, China). Then HieffTM qPCR SYBR Green Master Mix (Yeasen) was then used for qPCR with the Dcp2 primers mDcp2-626F and mDcp2-787R (). β-actin and 18S rRNA were used as internal controls with the primers mActb-864F and mActb-1028R for β-actin and mRN18S-843F and mRN18S-1079R for 18S rRNA (). For miRNA qPCR, 2 μg total RNA was transcribed into cDNA using a miRcute Plus miRNA First-Strand cDNA Synthesis Kit (Tiangen, Beijing, China). A miRcute Plus miRNA qPCR Detection Kit (Tiangen) was then used for qPCR with the forward primers miR-141-F and miR-200a-F (). The reverse primer for miRNAs qPCR was provided by the kit. U6 small nuclear RNA (snRNA) was used as the internal control with the primers RNU6-5F and RNU6-99R ().
Construction of luciferase reporter plasmids
The coding region of firefly luciferase was excised from the pGL3-basic vector using HindIII and XbaI and was inserted into a pcDNA3.1 vector digested with the same restriction enzymes. DNA oligos containing KpnI, ClaI and AgeI restriction sites were then inserted into the XbaI site to generate the pcDNA3.1-Luc vector. The 5ʹ half of the 3ʹ UTR of Dcp2 was amplified from mouse genomic DNA using Q5 DNA polymerase (NEB, Ipswich, MA, USA) and was cloned into the pcDNA3.1-Luc vector using a Hieff Clone One Step Cloning Kit (Yeasen) with the primers Dcp2-5ʹ-half-F and Dcp2-5ʹ-half-R () to generate the pcDNA3.1-Luc-Dcp2-5ʹ-half (Luc-5ʹ-half) reporter. The amplified fragment of the 3ʹ half of the 3ʹ UTR of Dcp2 was inserted into the pcDNA3.1-Luc vector digested with KpnI and AgeI with the primers Dcp2-3ʹ-half-F and Dcp2-3ʹ-half-R () to generate the pcDNA3.1-Luc-Dcp2-3ʹ-half (Luc-3ʹ-half) reporter. The sequences of both plasmids were confirmed by sequencing.
MiRNAs transfection and dual luciferase assay
One day before transfection, HEK293T cells were seeded in 12-well plates at a concentration of 2.5 × 104 cells per well. A total of 200 ng luciferase reporter plasmid and 50 ng pRL-SV40 Renilla luciferase reporter were transfected with mimic or mutant miRNAs (Ribobio, Guangzhou, China) at a final concentration of 100 nM using Lipofectamine® 3000 reagent (Invitrogen, Carlsbad, CA, USA). After 48 h, firefly and Renilla luciferase activities were measured using a Dual-Luciferase Reporter System (Promega, Madison, WI, USA) following the manufacturer’s protocol. To test the effect of mimic and mutant miRNAs on endogenous Dcp2 expression, 1.2 × 105 NIH3T3 cells were seeded in 6-well plates one day before transfection. Wild type, mutant and negative control miRNAs were transfected into cells at a final concentration of 100 nM using lipofectamine® 3000 (Invitrogen). 48 h after transfection, the expression of endogenous Dcp2 was measured using Western blot.
Statistical analysis
Statistical analyses were performed using Student’s t-tests in Excel (Microsoft, Redmond, WA, USA). Data are presented as the mean ± standard deviation (SD) of three separate experiments. P values <0.05 were considered statistically significant.
Results
Expression of Dcp2 protein and mRNA in mouse kidney during development
According to our previous study, Dcp2 is expressed in embryonic but not in adult mouse kidney [Citation9]. As shown in ,), during renal development, Dcp2 protein was highly expressed in the kidney at embryonic day (E) 15.5 and E18.5 but its expression declined after birth. The expression of Dcp2 decreased to undetectable levels at postnatal day 10 (P10), when nephrogenesis had stopped and remained undetectable at 6 weeks (,)). To determine if Dcp2 mRNA levels were consistent with Dcp2 protein expression, Dcp2 mRNA levels were measured at different stages of renal development using qPCR. Surprisingly, although Dcp2 mRNA decreased during renal development, a substantial amount of Dcp2 mRNA was still expressed at P10 and 6 weeks. As shown in ), when mRNA was normalized to β-actin, Dcp2 mRNA was expressed at approximately 50% of E15.5 levels at P10 and 6 weeks, when Dcp2 protein was no longer detected (,c)). Because β-actin may be differentially expressed during renal development, 18S rRNA was used as a second internal control to normalize Dcp2 mRNA expression. ) shows that at P10 and 6 weeks, Dcp2 mRNA was expressed at 40% and 30% of E15.5 levels, respectively ()). These data indicate that, although Dcp2 protein was absent in the kidney after nephrogenesis stopped, Dcp2 mRNA was maintained. However, it was unclear whether the Dcp2 mRNA was completely silenced, similar to some maternal mRNAs in oocytes, which are silenced by the formation of RNA-protein complexes [Citation19], or whether Dcp2 proteins were degraded by proteolytic machinery immediately after synthesis.
Figure 1. Dcp2 protein and mRNA expression during renal development in mice. (A) Dcp2 protein expression in kidneys at the indicated developmental stages was determined by Western blot. β-actin was used for normalization. (B) Quantification of Dcp2 protein expression. Dcp2 mRNA levels during renal development were determined by qPCR and normalized to (C) β-actin and (D) 18S rRNA. Results are shown as mean ± SD and all experiments were performed in triplicate. 6W, postnatal week 6.
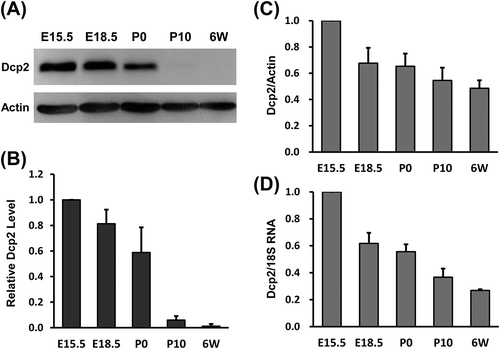
Dcp2 mRNA in adult mice kidneys is not completely silenced
To investigate whether Dcp2 mRNA was completely silenced or not in the adult kidney, Dcp2-IRES-EGFP knock-in mice were generated. An IRES-EGFP fragment was knocked into the 3ʹ UTR of Dcp2 17 nucleotides downstream of the stop codon ()). The EGFP reporter could then be transcribed with Dcp2 and its translation initiated under the control of IRES. If Dcp2 mRNA was completely silenced by RNA-binding proteins with translation-inhibitory functions, the expression of Dcp2 and EGFP would be simultaneously repressed.
Figure 2. Dcp2 mRNA is not silenced in kidneys of adult mice. (A) Schematic representation of the Dcp2-IRES-EGFP knock-in strategy using CRISPR/Cas9. (B) Genotyping of Dcp2-IRES-EGFP mice using primers indicated in (A). (C) Dcp2 and EGFP protein expression in kidneys and testes of 6-week-old mice was determined by Western blot. β-actin was used for normalization. WT, wild type; KI, EGFP knock-in.
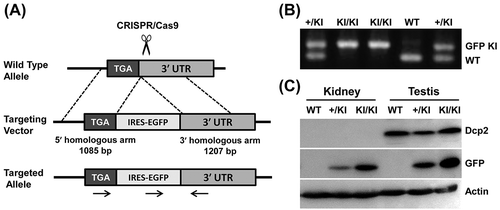
The genotypes of Dcp2-IRES-EGFP knock-in mice were confirmed by PCR ()) and the expression of Dcp2 and EGFP in kidney tissue was analyzed in both heterozygous and homozygous knock-in mice. As shown in ), Dcp2 protein was below the detectable level in kidneys of adult knock-in mice, which is consistent with the result shown in ). However, EGFP was clearly detected in the kidneys of both heterozygous and homozygous knock-in mice ()). The IRES-EGFP fragment knock-in did not affect endogenous Dcp2 protein expression, as Dcp2 protein levels in testes of knock-in mice were not different from those of wild type mice ()). These results indicate that Dcp2 mRNA is not silenced in kidneys of adult mice. One of the regulatory mechanisms of miRNAs is to inhibit cap-dependent, but not IRES-dependent, translation initiation of their target mRNAs, resulting in translational repression without affecting RNA levels. The above data suggest that Dcp2 may be subjected to post-transcriptional regulation by miRNA in the late stages of kidney development.
miR-141-3p and miR-200a-3p directly target the 3ʹ UTR of Dcp2 mRNA
To find potential miRNAs that target Dcp2, the online bioinformatics tools TargetScan 7.1 (http://www.targetscan.org/vert_71/) and miRanda (http://34.236.212.39/microrna/home.do) were used to predict potential miRNA binding sites in the 3ʹ UTR of Dcp2. Bioinformatics analysis showed that many miRNAs are predicted to bind in the 3ʹ UTR of Dcp2. Interestingly, most predicted miRNAs with high probability of conserved targeting (PCT) values [Citation20] were predicted to bind to sites in the 5ʹ half of the 3ʹ UTR of Dcp2. MiR-141-3p and miR-200a-3p were found to have two potential binding sites in the 3ʹ UTR of Dcp2 at nucleotides 209–215, a relative well-conserved region in many mammals, and nucleotides 3753–3759, a less conserved region ()).
Figure 3. Luciferase reporter assays show that miR-141-3p and miR-200a-3p target the 3ʹ UTR of Dcp2. (A) Schematic representation of the highly conserved binding site (top) and the less conserved site (bottom) of miR-141-3p and miR-200a-3p in the Dcp2 3ʹ UTR of different species. Potential sequences base-pairing with miRNA seed regions are shown in grey. Mutations inserted into the seed regions of miRNAs are underlined and shown on the top with miR-200a-3p as representative. (B) Wild type, but not mutant, miR-141-3p and miR-200a-3p inhibit activity of the Luc-5ʹ-half reporter when cotransfected into HEK293T cells. (C) miR-141-3p and miR-200a-3p do not inhibit activity of the Luc-3ʹ-half reporter in dual luciferase assay experiments. Results are shown as mean ± SD and all luciferase experiments were performed in triplicate. P values of Student’s t-tests are indicated by asterisks. * P < 0.05. ** P < 0.01. NC, negative control miRNA.
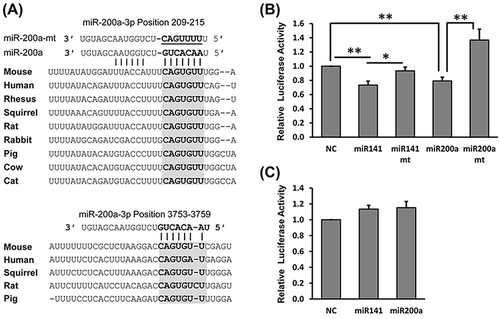
To test whether miR-141-3p and miR-200a-3p can target Dcp2, the 5ʹ (nucleotides 1–3824) and 3ʹ (nucleotides 3692–7214) halves of the 3ʹ UTR of Dcp2 were each cloned downstream of the firefly luciferase gene to generate Luc-5ʹ-half and Luc-3ʹ-half reporters for use in dual luciferase assays. Compared to control miRNA, luciferase activity was significantly lower when miR-141-3p and miR-200a-3p mimics were co-transfected with the Luc-5ʹ-half, but not the Luc-3ʹ-half, reporter (, )). These results indicate that the binding site at nucleotides 3753–3759, which is the only binding site in the Luc-3ʹ-half reporter, is either not targeted by miR-141-3p and miR-200a-3p or cannot function independently. The suppression of the Luc-5ʹ-half reporter by miR-141-3p and miR-200a-3p may be due to the additive effects of the two binding sites or may be effected mainly through the more conserved binding site at nucleotides 209–215 in Luc-5ʹ-half reporter. To further confirm the regulatory function of miR-141-3p and miR-200a-3p on Dcp2, mutations were introduced into the seed regions of miR-141-3p and miR-200a-3p. As shown in ), when mutant miR-141-3p and miR-200a-3p were co-transfected with the Luc-5ʹ-half reporter, their inhibitory effect was abolished ()). For unknown reasons, mutant miR-200a-3p stimulated low luciferase activity of the Luc-5ʹ-half reporter. These results show that Dcp2 can be targeted by miR-141-3p and miR-200a-3p.
Effect of overexpression of miR-141-3p and miR-200a-3p on endogenous Dcp2 protein and mRNA in NIH3T3 cells
To further investigate whether miR-141-3p and miR-200a-3p inhibit endogenous Dcp2 protein expression, both miR-141-3p and miR-200a-3p were transfected into NIH3T3 cells. After 48 h, Dcp2 expression was examined by Western blot. As shown in ), Dcp2 protein expression was significantly downregulated in cells transfected with wild type miR-141-3p and miR-200a-3p compared to cells transfected with negative control miRNA. However, the downregulation of Dcp2 protein expression was abolished when seed regions of miR-141-3p and miR-200a-3p were mutated (,b)). When Dcp2 mRNA levels were measured by qPCR, we found that neither wild type nor mutant miR-141-3p and miR-200a-3p promoted Dcp2 mRNA degradation ()). These results demonstrate that miR-141-3p and miR-200a-3p post-transcriptionally regulate Dcp2 expression by inhibiting Dcp2 protein translation but do not accelerate decay of Dcp2 mRNA.
Figure 4. Overexpression of miR-141-3p and miR-200a-3p downregulates endogenous Dcp2 protein expression but do not affect Dcp2 mRNA levels in NIH3T3 cells. (A) Wild type and mutant miR-141-3p and miR-200a-3p were transfected into NIH3T3 cells. After 48 h, Dcp2 protein levels were measured by Western blot. β-actin was used for normalization. Experiments were performed in triplicate. A representative image is shown in the top panel. (B) Quantification of Dcp2 protein expression. Data are shown as mean ± SD. P values of Student’s t-tests are indicated by asterisks. * P < 0.05. ** P < 0.01. (C) Wild type and mutant miR-141-3p and miR-200a-3p were transfected into NIH3T3 cells and Dcp2 mRNA levels were determined by qPCR with β-actin as internal control. Data are shown as mean ± SD.
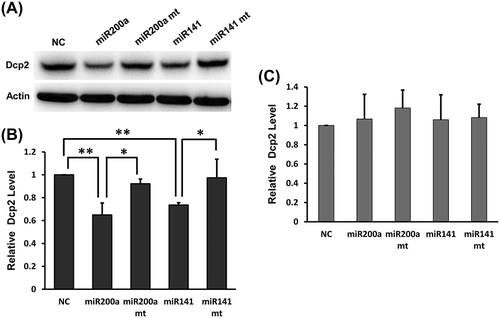
miR-141-3p and miR-200a-3p expression increases dramatically during renal development in mice
To investigate whether miR-141-3p and miR-200a-3p expression is negatively correlated with Dcp2 protein expression during renal development in mice, miR-141-3p and miR-200a-3p levels were examined using qPCR at different stages of kidney development. As shown in , both miR-141-3p and miR-200a-3p were slightly upregulated in kidney tissue from E15.5 to P0. However, their expression dramatically increased after P10. MiR-141-3p expression increased by 3-fold at P10 and 6-fold at 6 weeks, whereas miR-200a-3p expression increased by 10-fold at P10 and 19-fold at 6 weeks (). These expression levels were negatively correlated with those of Dcp2 protein, which was highly expressed in embryonic kidneys but absent in kidneys after P10, although a substantial amount of Dcp2 mRNA was still expressed (). These data suggest that miR-141-3p and miR-200a-3p may post-transcriptionally repress Dcp2 expression during renal development.
Figure 5. MiR-141-3p and miR-200a-3p expression increases significantly during renal development. qPCR was used to measure (A) miR-141-3p and (B) miR-200a-3p levels at different stages of kidney development. The results were normalized to U6 snRNA expression. The expression level at E15.5 was set to 1. Data are shown as mean ± SD and all experiments were performed in triplicate.
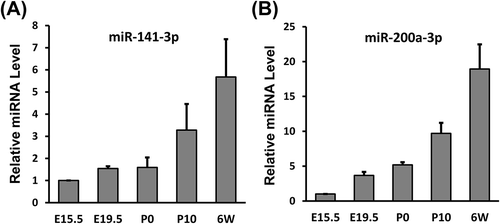
Discussion
Previously, we found that Dcp2 protein is expressed in embryonic, but not adult, kidneys in mice [Citation9]. In this study, we showed that Dcp2 mRNA was still expressed in kidneys after nephrogenesis was complete. MiR-141-3p and miR-200a-3p were confirmed to target Dcp2 mRNA by luciferase reporter assays. Endogenous Dcp2 expression was downregulated when miR-141-3p and miR-200a-3p were overexpressed. Most importantly, both miR-141-3p and miR-200a-3p expression dramatically increased in kidneys of adult mice and their expression was negatively correlated with Dcp2 protein levels. These data indicate that miR-141-3p and miR-200a-3p may post-transcriptionally regulate Dcp2 expression during renal development.
During kidney development, the expression of a series of genes is coordinated to ensure that progenitor cells correctly differentiate into nephrons. This gene expression is precisely controlled by transcriptional, post-transcriptional and epigenetic regulation [Citation6]. RNA degradation machinery also regulates genes expression at the post-transcriptional level. Dcp2, an important component of the RNA degradation machinery, has been found to be involved in many essential processes, such as cell cycle [Citation21], autophagy [Citation22,Citation23], development [Citation24,Citation25], innate immune response [Citation26] and gametogenesis [Citation27,Citation28]. Dcp2 is only expressed in the embryonic kidney and may be involved in post-transcriptional regulation of gene expression during renal development. To our knowledge, the target genes of Dcp2 have not been investigated by deletion of Dcp2 during the early stages of renal development. The fact that Dcp2 mRNA is expressed in kidneys of adult mice when Dcp2 protein is undetectable indicates that Dcp2 is either subjected to post-transcriptional inhibition by miRNAs or is quickly degraded by the proteasome. Bicistronic reporters containing IRES have been widely used to investigate the translational repression mechanism of miRNAs. Using a Dcp2-IRES-EGFP knock-in mouse model, we found that cap-dependent Dcp2 translation in adult kidneys was repressed but IRES-dependent EGFP translation was not, which is consistent with the known regulatory mechanism of some miRNAs. Dcp2 has been found to be targeted by miR-224 in methotrexate-resistant HT29 colon cancer cells [Citation29]. These results support the hypothesis that Dcp2 is likely post-transcriptionally regulated by miRNAs during the late stages of kidney development.
Dcp2 mRNA has a fairly long 3ʹ UTR of 7.2 kb that contains many potential miRNA binding sites. To identify potential miRNAs that bind in this region, we used three criteria. First, miRNA binding sites must be conserved in mammals. Second, miRNA expression must be increased during renal development according to deep sequencing data deposited in the Gene Expression Omnibus (GEO) database. Third, miRNAs must be enriched in adult mouse kidney but not brain, where Dcp2 is highly expressed, according to GEO miRNA sequencing data. Based on our search criteria, five miRNAs were chosen as candidates for luciferase reporter assays. MiR-200b did not inhibit the luciferase reporter activity, whereas miR-148/152 repressed luciferase reporter activity in a Dcp2 3ʹ UTR-independent manner (data not shown). Only miR-141-3p and miR-200a-3p were confirmed to repress Dcp2 expression. Luciferase reporter assays showed that miR-141-3p and miR-200a-3p targeted the 5ʹ, but not 3ʹ, half of the 3ʹ UTR of Dcp2. The 5ʹ half contains two predicted binding sites, whereas the 3ʹ half contains only one predicted binding site. MiR-141-3p and miR-200a-3p effectively inhibited Luc-5ʹ-half reporter activity, possibly due to additive effects of the two binding sites. Another possibility is that miR-141-3p and miR-200a-3p target Dcp2 mainly through the highly conserved site at nucleotides 209–215 in the 3ʹ UTR of Dcp2 but not through the less conserved site at nucleotides 3753–3759. The six additional bases in the 3ʹ half of miR-141-3p and miR-200a-3p that are complementary to the sequence adjacent to the first conserved site in the 3ʹ UTR of Dcp2 may stabilize the interaction. The site at nucleotides 3753–3759 in the Luc-3ʹ-half reporter may function in conjunction with the site at nucleotides 209–215 or may not be targeted by miR-141-3p and miR-200a-3p because its structure may make it inaccessible to miRNAs.
Usually, miRNAs and their targets are exclusively expressed in specific cells. In the present study, we found that miR-141-3p and miR-200a-3p were expressed at low levels in the embryonic kidney but that their expression dramatically increased after P10 (). Furthermore, miR-141-3p and miR-200a-3p expression was negatively correlated with Dcp2 protein expression. Dcp2 proteins were absent in kidneys after P10 despite a substantial amount of Dcp2 mRNAs remaining. Considering that miR-141-3p and miR-200a-3p only inhibit Dcp2 protein translation and do not promote Dcp2 mRNA decay in vivo (), the absence of Dcp2 proteins in kidneys after P10 may be due in part to the post-transcriptional repression of Dcp2 by miR-141-3p and miR-200a-3p. Deletion of miR-141-3p and miR-200a-3p or their target sequences in the 3ʹ UTR of Dcp2 is necessary to confirm that miR-141-3p and miR-200a-3p repress Dcp2 protein expression during renal development. Targeted degradation of Dcp2 protein by the proteasome cannot be excluded, but it will be difficult to specifically inhibit proteasome activity in kidney in vivo to test Dcp2 protein expression.
The embryonic kidney contains many different types of cells, including nephron progenitor cells, tubular cells and interstitial cells. Since it was unknown which cells express Dcp2, we attempted to identify Dcp2-expressing cells in embryonic kidneys using Dcp2-IRES-EGFP knock-in mice. Unfortunately, no EGFP signal could be detected, possibly due to the low translation initiation efficiency of the encephalomyocarditis virus IRES used in this mouse model compared to cap-mediated translation initiation. Anti-EGFP and Dcp2 antibodies were also tested but neither is suitable for immunofluorescence. Other methods could be used to investigate the localization of Dcp2 in embryonic kidneys, such as crossing Dcp2 promoter-controlled Cre-ERT2 recombinase-expressing mice (Dcp2-Cre-ERT2) with Rosa26-CAG-ZsGreen reporter mice and activating the reporter by tamoxifen administration. Similarly, the identity of miR-141-3p and miR-200a-3p-expressing cells is also unknown. Therefore, it is not clear which types of cells are the target of negative regulation of Dcp2 by miR-141-3p and miR-200a-3p. Considering the absence of Dcp2 protein in adult kidneys of mice, miR-141-3p and miR-200a-3p may suppress Dcp2 expression, at least in some types of Dcp2-expressing cells.
It is not clear why Dcp2 is regulated at the post-transcriptional level rather than being transcriptionally silenced in adult kidney. It has been reported that many embryonically expressed proteins involved in renal development are upregulated or re-expressed during recovery of acute kidney injuries. For example, WT1 and Pax2, which are essential for tubule development, have been found to be re-expressed during the tubular epithelial-mesenchymal transition in 5/6 nephrectomized rats [Citation30]. Pax2 is also upregulated in tubular cells during regeneration after renal ischemia reperfusion injury (I/RI) [Citation31]. Six2, a nephron progenitor cell marker, is also significantly upregulated during the early stages of post ischemia [Citation32]. However, it is not known whether Dcp2 is involved in kidney regeneration. As an embryonically-expressed kidney protein, re-expression of Dcp2 after I/RI might promote dedifferentiation and proliferation of resident tubule epithelial cells by degrading differentiation-related genes. Thus, tubule cells can benefit from the post-transcriptional control of Dcp2 by miRNAs such as miR-141-3p and miR-200a-3p, as Dcp2 can be quickly re-expressed through clearance of the inhibitory miRNAs.
It is not clear whether other miRNAs or proteins also participate in the post-transcriptional inhibition of Dcp2 expression during renal development in mice. Additionally, why Dcp2 is subjected to post-transcriptional repression rather than silencing at the transcription level in the adult kidney requires further investigation.
Author contributions
Ming-Nan Zhang performed the experiments and wrote the manuscript. Qun-Ye Tang and Rui-Min Li were responsible for the mouse maintenance. Man-Gen Song designed the experiments and wrote the manuscript.
Competing Interests
The authors declare no competing interests.
Acknowledgments
We thank Yi Zhang and other members of the lab for technical assistance. We also thank Professor Megerditch Kiledjian (Rutgers University, USA) for supplying the anti-Dcp2 antibody.
Disclosure statement
No potential conflict of interest was reported by the authors.
Additional information
Funding
References
- Chai O-H, Song C-H, Park S-K, et al. Molecular regulation of kidney development. Anat Cell Biol. 2013;46:19–31.
- Hartman HA, Lai HL, Patterson LT. Cessation of renal morphogenesis in mice. Dev Biol. 2007;310:379–387.
- Brodbeck S, Englert C. Genetic determination of nephrogenesis: the Pax/Eya/Six gene network. Pediatr Nephrol. 2004;19:249–255.
- Wellik DM, Yallowitz A, Gong N, et al. Hox11 genes interact with Eya1 and Pax2 to activate Six2 and Gdnf expression during metanephric kidney induction. Dev Biol. 2006;295:388.
- Park JS, Ma WX, O’Brien LL, et al Six2 and Wnt regulate self-renewal and commitment of nephron progenitors through shared gene regulatory networks. Dev Cell. 2012;23:637–651.
- Nagalakshmi VK, Ren Q, Pugh MM, et al Dicer regulates the development of nephrogenic and ureteric compartments in the mammalian kidney. Kidney Int. 2011;79:317–330.
- Nakagawa N, Xin C, Roach AM, et al Dicer1 activity in the stromal compartment regulates nephron differentiation and vascular patterning during mammalian kidney organogenesis. Kidney Int. 2015;87:1125–1140.
- Filipowicz W, Bhattacharyya SN, Sonenberg N. Mechanisms of post-transcriptional regulation by microRNAs: are the answers in sight? Nat Rev Genet. 2008;9:102–114.
- Song MG, Li Y, Kiledjian M. Multiple mRNA decapping enzymes in mammalian cells. Molecular Cell. 2010;40:423–432.
- Wang Z, Jiao X, Carr-Schmid A, et al. The hDcp2 protein is a mammalian mRNA decapping enzyme. Proc Natl Acad Sci U S A. 2002;99:12663–12668.
- Li Y, Kiledjian M. Regulation of mRNA decapping. Wiley Interdiscip Rev RNA. 2010;1:253–265.
- Franks TM, Lykke-Andersen J. The control of mRNA decapping and P-Body formation. Molecular Cell. 2008;32:605–615.
- Li Y, Song M, Kiledjian M. Differential utilization of decapping enzymes in mammalian mRNA decay pathways. Rna. 2011;17:419–428.
- Li Y, Song MG, Kiledjian M. Transcript-specific decapping and regulated stability by the human Dcp2 decapping protein. Mol Cell Biol. 2008;28:939–948.
- Li Y, Ho ES, Gunderson SI, et al. Mutational analysis of a Dcp2-binding element reveals general enhancement of decapping by 5 ‘-end stem-loop structures. Nucleic Acids Res. 2009;37:2227–2237.
- Song MG, Kiledjian M. 3‘ Terminal oligo U-tract-mediated stimulation of decapping. Rna-A Publ Rna Soc. 2007;13:2356–2365.
- Mullen TE, Marzluff WF. Degradation of histone mRNA requires oligouridylation followed by decapping and simultaneous degradation of the mRNA both 5ʹ to 3ʹ and 3ʹ to 5ʹ. Genes Dev. 2008;22:50–65.
- Mauer J, Luo X, Blanjoie A, et al Reversible methylation of m(6)A(m) in the 5 ‘ cap controls mRNA stability. Nature. 2017;541:371–375.
- Webster PJ, Liang L, Berg CA, et al. Translational repressor bruno plays multiple roles in development and is widely conserved. Genes Dev. 1997;11:2510–2521.
- Friedman RC, Farh KK-H, Burge CB, et al. Most mammalian mRNAs are conserved targets of microRNAs. Genome Res. 2009;19:92–105.
- Hopkins KC, McLane LM, Maqbool T, et al A genome-wide RNAi screen reveals that mRNA decapping restricts bunyaviral replication by limiting the pools of Dcp2-accessible targets for cap-snatching. Genes Dev. 2013;27:1511–1525.
- Hu GW, McQuiston T, Bernard A, et al A conserved mechanism of TOR-dependent RCK-mediated mRNA degradation regulates autophagy. Nat Cell Biol. 2015;17:930–942.
- Hu GW, McQuiston T, Bernard A, et al TOR-dependent post-transcriptional regulation of autophagy. Autophagy. 2015;11:2390–2392.
- Xu J, Yang JY, Niu QW, et al. Arabidopsis DCP2, DCP1, and VARICOSE form a decapping complex required for postembryonic development. Plant Cell. 2006;18:3386–3398.
- Mishima Y, Tomari Y. Pervasive yet nonuniform contributions of Dcp2 and Cnot7 to maternal mRNA clearance in zebrafish. Genes to Cells. 2017;22:670–678.
- Li Y, Dai JH, Song MG, et al. Dcp2 decapping protein modulates mRNA stability of the critical Interferon Regulatory Factor (IRF) IRF-7. Mol Cell Biol. 2012;32:1164–1172.
- Ma J, Flemr M, Strnad H, et al. Maternally recruited DCP1A and DCP2 contribute to messenger RNA degradation during oocyte maturation and genome activation in mouse. Biol Reprod. 2013;88:12.
- Shapouri F, Saeidi S, de Iongh RU, et al Tob1 is expressed in developing and adult gonads and is associated with the P-body marker, Dcp2. Cell Tissue Res. 2016;364:443–451.
- Mencia N, Selga E, Noe V, et al. Underexpression of miR-224 in methotrexate resistant human colon cancer cells. Biochem Pharmacol. 2011;82:1572–1582.
- Huang B, Pi L, Chen C, et al WT1 and Pax2 re-expression is required for epithelial-mesenchymal transition in 5/6 nephrectomized rats and cultured kidney tubular epithelial cells. Cells Tissues Organs. 2012;195:296–312.
- Maeshima A, Maeshima K, Nojima Y, et al. Involvement of Pax-2 in the action of activin A on tubular cell regeneration. J Am Soc Nephrol. 2002;13:2850–2859.
- Metsuyanim S, Pode-Shakked N, Schmidt-Ott KM, et al Accumulation of malignant renal stem cells is associated with epigenetic changes in normal renal progenitor genes. STEM CELLS. 2008;26:1808–1817.