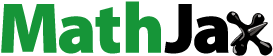
ABSTRACT
Grifola frondosa is an edible mushroom consumed as a health food and/or traditional medicine in Asia. However, the anti-allergic effects of G. frondosa are not yet understood. In this study, we demonstrated the effects of G. frondosa extract (GFE) on IgE-mediated allergic responses, using antigen-stimulated RBL-2H3 cells. Three active compounds: ergosterol, 6β-methoxyergosta-7,22-dien-3β,5α-diol (MEDD), and 6-oxoergosta-7,22-dien-3β-ol (6-OXO) were isolated from GFE and shown to inhibit the antigen-induced release of β-hexosaminidase and histamine. Among the three active components, we focused on ergosterol because of its high content in GFE. Ergosterol inhibited the aggregation of high-affinity IgE receptor (FcεRI), which is the first step in the activation of mast cells and antigen-induced tyrosine phosphorylation. Furthermore, ergosterol suppressed antigen-increased IL-4 and TNF-α mRNA. Taken together, our findings suggest that G. frondosa, including ergosterol and its derivatives as active components, has the potential to be a novel functional food that prevents type I allergies.
Graphical Abstract
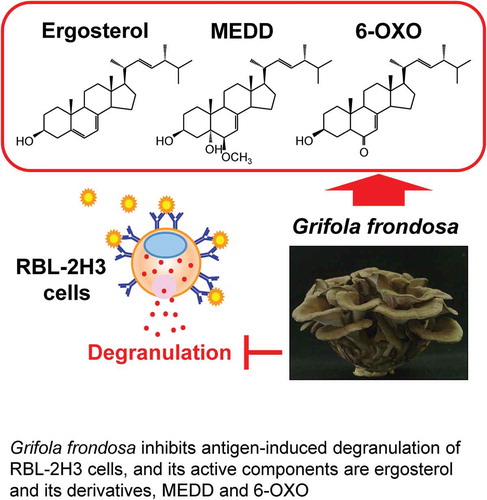
Grifola frondosa (G. frondosa), a basidiomycete fungus belonging to the Polyporaceae family, is a popular edible mushroom in Asia [Citation1], and is consumed as a health food and/or traditional medicine. Previous studies have shown that G. frondosa has pharmacologic effects, such as anti-cancer [Citation2–Citation4], anti-inflammation [Citation5] and anti-diabetes activity [Citation6–Citation8]. These immunomodulatory properties are noteworthy and several polysaccharides and/or proteins of G. frondosa were identified as its active components [Citation1–Citation3,Citation7,Citation9]. Furthermore, it was reported that G. frondosa had anti-allergic activity in mice. The oral administration of G. frondosa suppressed 2,4-dinitro-chlorobenzene-induced atopic dermatitis-like skin lesions in NC/Nga mice [Citation10]. However, the active components and mechanisms of G. frondosa against allergic disease have not been elucidated.
Type I allergy is classified as IgE-mediated immediate hypersensitivity in which mast cells play important roles. Antigen-induced release of inflammatory mediators from mast cells causes the immediate symptoms of IgE-mediated allergic diseases, including allergic rhinitis (hay fever), asthma, food allergy, and anaphylaxis [Citation11–Citation13].
Mast cells express high-affinity IgE receptors (FcεRI) on their surface and the antigen-induced crosslinking of FcεRI-bound IgE initiates downstream signaling pathways, such as the phosphorylation of tyrosine kinase [Citation14] that induces degranulation, arachidonic acid release and cytokine production [Citation15,Citation16]. Inflammatory mediators produced and secreted from mast cells cause inflammatory and allergic disease. Therefore, the prevention of mast cell activation is of great importance for the reduction of allergic symptoms.
RBL-2H3, a rat mast cell line, is a useful cell line for the in vitro screening of anti-allergic agents [Citation17–Citation20]. In the present study, to reveal the anti-allergic activity of G. frondosa, we investigated the inhibitory effects of G. frondosa extract (GFE) on the degranulation of antigen-stimulated RBL-2H3 cells. In addition, we identified the active components of G. frondosa and elucidated the molecular mechanisms of the inhibitory effects on RBL-2H3 cell degranulation.
Materials and methods
Materials
Fruit bodies of G. frondosa were cultivated by the Hokuto Corporation (Nagano, Japan). Dinitrophenyl-human serum albumin (DNP-HSA) was purchased from Sigma-Aldrich (St Louis, MO, USA). Ergosterol and Wakogel C300 were purchased from Wako Pure Chemical Corporation (Osaka, Japan). Tranilast was purchased from Tokyo Chemical Industry Co., Ltd. (Tokyo, Japan) Bond Elut C18 was from Agilent Technologies (Santa Clara, CA, USA). Methyl-β-cyclodextrin (MβCD) was purchased from Sigma-Aldrich. Anti-phosphotyrosine and anti-β-actin antibodies were from BD Bioscience (Sparks, MD, USA) and Santa Cruz Biotechnology (Santa Cruz, CA, USA). Goat anti-mouse IgE antiserum and Alexa Fluor 488-conjugated anti-goat IgG were from Bethyl Laboratories, Inc. (Montgomery, TX, USA), and Abcam (Cambridge, MA, USA), respectively.
Preparation of extracts and isolation of bioactive constituents from G. frondosa
The lyophilisate of G. frondosa (204.5 g) was powdered and extracted with methanol (2 L) at room temperature for 3 days. The extract was evaporated and fractionated by solvent partition between ethyl acetate and water. The ethyl acetate soluble fraction (GFE, 1.23 g) was chromatographed on silica gel (Wakogel C300, 50 g) with an ethyl acetate/hexane mixture (0%, 10%, 20%, 30%, 40%, 50%, 60%, 80%, and 100% ethyl acetate/hexane, 200 mL each) and finally methanol, 200 mL by the stepwise elution to obtain 10 fractions (Fractions 1–10). Fraction 4 (the 30% ethyl acetate in hexane eluate, 642.1mg) was recrystallized from ethyl acetate to give compound 1 (197.6 mg). Fraction 7 (the 60% ethyl acetate in hexane eluate, 29.3 mg) was further purified by column chromatography on silica gel (Wakogel C300, 6 g) with 10% acetone in chloroform, each 1.0 mL of the eluate was collected in a test tube. In fraction no.57–65, compound 2 (7.5 mg) was yielded. Fraction 8 (the 80% ethyl acetate in hexane eluate, 18.6 mg) was further purified by column chromatography on silica gel (Wakogel C300, 1 g) with 1% 2-propanol in chloroform (30 mL) and finally with methanol (1 mL). The eluate with methanol was separated again by ODS (Bond Elut C18, 500 mg) column chromatography using the stepwise elution with 60% acetonitrile in water (15 mL), acetonitrile (15 mL) and methanol (15 mL) to give compound 3 (0.5 mg) in an eluate with methanol. Structures of compounds 1–3 were elucidated by their HREI-MS, 1H and 13C-NMR spectra.
Compound 1. HREI-MS: m/z 396.3408 [M]+ (calcd. for C28H44O: 396.3394). NMR (CDCl3) δH: 0.63 (3H, s), 0.82 (3H, d, J = 7.0 Hz), 0.84 (3H, d, J = 7.5 Hz), 0.92 (3H, d, J = 7.0 Hz), 0.95 (3H, s), 1.04 (3H, d, J = 6.5 Hz), 3.64 (1H, m), 5.18 (1H, dd, J = 7.5, 15.0 Hz), 5.22 (1H, dd, J = 7.0, 15.0 Hz), 5.39 (1H, m), 5.57 (1H, m).
Compound 2. HREI-MS: m/z 426.3506 [M-H2O]+ (calcd. for C29H46O2: 426.3500). NMR (CDCl3) δH: 0.60 (3H, s), 0.83 (3H, d, J = 7.5 Hz), 0.83 (3H, d, J = 7.5 Hz), 0.92 (3H, d, J = 7.0 Hz), 1.00 (3H, s), 1.02 (3H, d, J = 6.5 Hz), 3.40 (3H, s), 3.17 (1H, d, J = 5.0 Hz), 4.08 (1H, m), 5.16 (1H, dd, J = 8.5, 15.5 Hz), 5.23 (1H, dd, J = 7.5, 15.5 Hz), 5.40 (1H, m). NMR (CDCl3) δC: 12.3, 17.6, 18.4, 19.7, 20.0, 21.1, 22.2, 22.9, 28.0, 30.9, 32.8, 33.1, 37.3, 39.4, 39.6, 40.4, 42.8, 43.9, 43.9, 55.0, 56.0, 58.4, 67.9, 76.4, 82.4, 115.0, 132.1, 135.5, 143.7.
Compound 3. HREI-MS: m/z 428.3360 [M]+ (calcd. for C28H44O3: 428.3292). NMR (CDCl3) δH: 0.62 (3H, s), 0.82 (3H, d, J = 7.5 Hz), 0.84 (3H, d, J = 7.5 Hz), 0.92 (3H, d, J = 7.0 Hz), 0.96 (3H, s), 1.03 (3H, d, J = 6.5 Hz), 4.03 (1H, m), 5.18 (1H, dd, J = 8.5, 15.5 Hz), 5.22 (1H, dd, J = 7.5, 15.5 Hz), 5.66 (1H, m). NMR (CDCl3) δC: 12.0, 15.7, 16.9, 18.9, 19.2, 20.4, 21.2, 21.7, 27.1, 29.5, 29.6, 32.3, 35.8, 38.1, 39.6, 39.7, 42.1, 43.2, 44.1, 55.1, 55.3, 66.8, 77.1, 119.0, 131.8, 134.3, 198.0.
Cell culture
RBL-2H3 cells were obtained from the Health Science Research Resources Bank (Osaka, Japan) and were grown in Eagle’s Minimal Essential Medium (Nissui Seiyaku, Tokyo, Japan) supplemented with 10% (v/v) heat-inactivated fetal bovine serum, penicillin G (15 μg/mL), and streptomycin (50 μg/mL). The cells were cultivated in an incubator at 37°C with 5% CO2.
β-hexosaminidase release assay
RBL-2H3 cells were seeded into 24-well plates at a density of 2.5 × 105 cells/well and sensitized with anti-DNP IgE using conditioned medium (10 μL/mL) from a DNP-specific IgE-producing hybridoma (kindly supplied by Dr. Kazutaka Maeyama, Ehime University, Ehime, Japan). After culture for 24 hours in a humidified atmosphere of 5% CO2/95% air at 37°C, the cells were washed with PIPES buffer (25 mM PIPES pH 7.2, 119 mM NaCl, 5 mM KCl, 5.6 mM glucose, 0.4 mM MgCl2, 2 mM CaCl2, and 0.1% BSA) two times and incubated in the 500 μL of same buffer in the presence or absence of samples for 20 minutes at 37°C. The samples were dissolved in dimethyl sulfoxide or ethanol of which the final concentration was maximum 0.5% and 1%, respectively. The cells were then challenged with DNP-HSA (20 ng/mL). Thirty minutes later, the supernatant was collected, and total lysate was prepared by adding 500 μL of 0.1% Triton-X 100 to wells without samples. Ten microliters of the supernatant or total lysate were mixed with an equal volume of substrate solution (1 mM p-nitrophenyl N-acetyl-β-D-glucosaminide in 0.1 M citrate buffer pH 4.5) and incubated for 1 h at 37°C. The reaction was stopped by adding 180 μL of stop buffer (0.1 M Na2CO3/NaHCO3, pH 10.0). The production of p-nitrophenol was determined by measuring the absorbance at 405 nm (OD405 nm) with an iMark™ microplate reader (Bio-Rad Laboratories, Hercules, CA, USA). Background fluorescence of the substrate in PIPES buffer alone was subtracted from all readings. The percentage of β-hexosaminidase release was calculated by using the following equation:
It is confirmed that all samples did not directly affect on β-hexosaminidase activity.
Histamine release assay
RBL-2H3 cells were seeded into 24-well plates at 2.5 × 105 cells/well and sensitized with anti-DNP IgE. After culture for 24 hours, the cells were washed with PIPES buffer two times and then pre-incubated with samples in PIPES buffer. After pre-incubation with test samples for 20 minutes, the cells were stimulated with DNP-HSA (20 ng/mL) for 30 minutes. Released histamine was determined by fluorometric assay based on the method of Shore et al. [Citation21]. Briefly, the conditioned media was collected and mixed with an equal volume of 0.8 N HClO4. The mixture was centrifuged (9,500 × g, 5 minutes), and the supernatant (500 μL) was transferred to a test tube. Subsequently, 5N NaOH (100 μL) and 1% (w/v) o-phthalaldehyde/methanol (50 μL) were added, and the mixture incubated at room temperature for 4 minutes. After stopping the reaction with 3N HCl (100 μL), the fluorescence (Ex 355 nm/Em 450 nm) was measured using an F-2000 Fluorescence Spectrophotometer (Hitachi, Tokyo, Japan).
Quantitative real-time PCR
RBL-2H3 cells were seeded into 24-well plates at 1.5 × 105 cells/well and sensitized with anti-DNP IgE. After culture for 24 hours, the cells were pre-incubated with ergosterol dissolved in ethanol. The final concentration of ethanol did not exceed 1%. After pre-incubation for 20 minutes, the cells were incubated for 2 hours with antigen. Ttotal RNA was extracted with RNAiso plus (Takara, Shiga, Japan) according to the manufacturer’s instructions. cDNA was synthesized from total RNA by reverse transcription using the PrimeScript RT master mix (Takara) according to the manufacturer’s instructions. Real-time PCR was performed using SYBR Premix Ex TaqTM II (Takara Bio, Otsu, Japan) in a Thermal Cycler Dice® Real Time System (TP800, Takara). As an internal control, glyceraldehyde-3-phosphate dehydrogenase (GAPDH) mRNA levels were quantified in parallel with the target genes. The primers used for real-time PCR were (forward) 5′-TCCTTACGGCAACAAGGAAC-3′ and (reverse) 5′-GTGAGTTCAGACCGCTGACA-3′ for rat IL-4 mRNA, (forward) 5′-GCATGATCCGAGATGTGGAA-3′ and (reverse) 5′-ACGAGCGGGAATGAGAAGAG-3′ for rat TNF-α and (forward) 5′-TGCCACTCAGAAGACTGTGG-3′ and (reverse) 5′-GGATGCAGGGATGATGTTCT-3′ for rat GAPDH. Normalization and fold changes were calculated using the ΔΔCt method. Primer specificity was confirmed by a melting curve analysis.
Western blot analysis
RBL-2H3 cells were seeded onto 12-well plates at 3 × 105 cells/well and sensitized with anti-DNP IgE. The cells were cultured for 24 hours, and then used for the following experiment. After incubation for 0–10 minutes with antigen stimulation in the presence or absence of ergosterol, cells were washed twice with ice-cold phosphate-buffered saline (PBS) and lysed in ice-cold lysis buffer (20 mM HEPES pH 7.4, 1% (v/v) Triton X-100, 10% (v/v) glycerol, 50 mM sodium fluoride, 2.5 mM p-nitrophenyl phosphate, 10 μg/mL phenylmethylsulfonyl fluoride, 1 mM Na3VO4, 10 μg/mL leupeptin, 1 mM EDTA). Samples were loaded on an 8% sodium dodecyl sulfate (SDS)-polyacrylamide gel for electrophoresis (PAGE), and transferred to nitrocellulose membranes. The membranes were blocked with Block Ace (DS Pharma Biomedical, Osaka, Japan), and then incubated with the primary antibody (mouse anti-phosphotyrosine antibody (1:500) or goat anti-β-actin antibody (1:500)) overnight at 4°C. Subsequently, the membranes were incubated with the secondary antibody (horseradish-peroxidase-conjugated anti-mouse IgG (Cell Signaling Technology, Beverly, MA, USA) or biotinylated anti-goat IgG (Vector Laboratories, Burlingame, CA, USA)). When a biotinylated antibody was used, the membrane was incubated for a further 30 minutes at room temperature with Vectastain ABC reagent (Vector Laboratories). The proteins were visualized using an enhanced chemiluminescence detection system (ECL; GE Healthcare Bioscience, Buckinghamshire, UK). The position of the target proteins were estimated from the calibration curve prepared from the migration distance of the molecular weight markers.
Immunofluorescence microscopy
RBL-2H3 cells were seeded into 24-well plates containing gelatin-coated cover glasses at 0.75 × 105 cells/well and sensitized with anti-DNP IgE. After culture for 24 hours, the cells cultured on cover glasses were pretreated with or without 50 μM ergosterol or 1 mM methyl beta cyclodextrin (MβCD) in PIPES buffer for 20 minutes. The cells were then challenged with DNP-HSA (50 ng/mL) for 20 minutes. After washing with ice-cold PBS immediately, the cells were fixed with 3.7% formaldehyde in PBS for 20 minutes and blocked with 1% BSA in PBS. The IgE/α-chain of FcεRI complexes on cell surfaces were detected using goat anti-mouse IgE antiserum, and Alexa Fluor 488-conjugated anti-goat IgG. Fluorescence images were acquired using a laser scanning confocal microscope (LSM700; Zeiss, Oberkochen, Germany) with Zen software (Zeiss). The data were quantified by counting the aggregation number of FcεRI positive cells and presented as aggregation positive cells/total cells. The cells were counted in six independent micrographs for each sample.
Statistical analysis
The data were expressed as the means ± standard errors of the means (SEMs). Results were analyzed using one-way ANOVA with Dunnett’s t-test. Differences with P values < 0.05 were considered statistically significant. The results were confirmed with at least three independent sets of experiments.
Results
GFE inhibits β-hexosaminidase release in antigen-stimulated RBL-2H3 cells
To investigate effects of GFE on antigen-induced degranulation, we determined the release of β-hexosaminidase, a known degranulation marker, from antigen-stimulated RBL-2H3 cells. The release of β-hexosaminidase was significantly suppressed by GFE at concentrations of 20 and 50 μg/mL, in a dose-dependent manner (). The inhibition by GFE at 50 μg/mL was similar to that of 50 μM of tranilast, a well-known anti-allergic drug (). The water extract of G. frondosa had no inhibitory effect on degranulation (date not shown).
Figure 1. Effect of GFE on β-hexosaminidase release in antigen-stimulated RBL-2H3 cells.
IgE-sensitized RBL-2H3 cells pretreated with GFE or tranilast (Tra) for 20 minutes were stimulated with DNP-HSA (20 ng/mL) for 30 minutes, and the release of β-hexosaminidase was measured. Each bar represents the mean ± SEM (n = 4). *** p < 0.001 vs. Control (Cont, DNP-HSA alone).
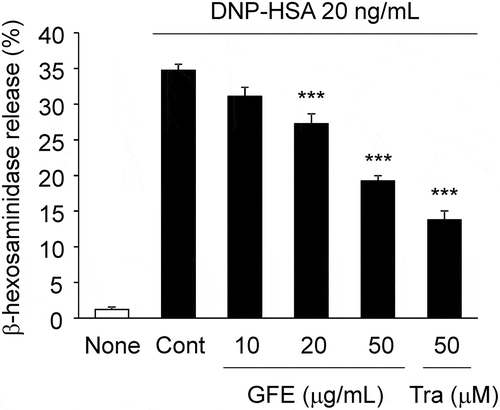
Ergosterol, MEDD, and 6OXO from GFE inhibit β-hexosaminidase and histamine release in antigen-stimulated RBL-2H3 cells
To identify the active compounds of GFE, we fractionated GFE by chromatography repeatedly and isolated compounds 1–3. These were identified as known sterols () ergosterol (1), 6β-methoxyergosta-7, 22-dien-3β, 5α-diol (MEDD, 2), and 6-oxoergosta-7, 22-dien-3β-ol (6-OXO, 3) by spectra data of HREI-MS, 1H-NMR and 13C-NMR, which was in good agreement with respective data previously reported [Citation22–Citation25] (Supplemental table 1–3). Pretreatment with Ergosterol, MEDD, or 6-OXO significantly inhibited antigen-mediated β-hexosaminidase () and histamine release (). These results suggested that ergosterol, MEDD, and 6-OXO inhibit degranulation in allergen-stimulated RBL-2H3 cells.
Figure 2. Structures of active compounds and effect on β-hexosaminidase and histamine release in antigen-stimulated RBL-2H3 cells.
Structures of ergosterol (A), 6β-methoxyergosta-7,22-dien-3β,5α-diol (MEDD) (B), and 6-oxoergosta-7,22-dien-3β-ol (6-OXO) (C). IgE-sensitized RBL-2H3 cells pretreated with active compounds, GFE, or tranilast (Tra) were stimulated with DNP-HSA, and the release of β-hexosaminidase (D) and histamine (E) were measured. Each bar represents the mean ± SEM (n = 3). * p < 0.05, *** p < 0.001 vs. Control (Cont, DNP-HSA alone).
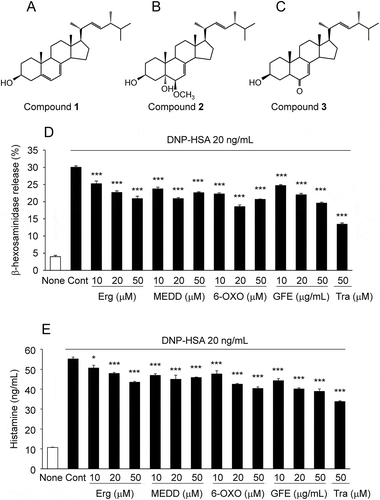
Cholesterol, β-Sitosterol, and Campesterol do not inhibit antigen-induced β-hexosaminidase and histamine release
We examined the effect of different types of sterol, cholesterol, β-sitosterol, and campesterol, on the antigen-induced release of β-hexosaminidase and histamine. Although the antigen-induced release of β-hexosaminidase and histamine were both inhibited by ergosterol consistent with , cholesterol, β-sitosterol, and campesterol had no inhibitory effect (). These results suggested that the inhibitory effect of ergosterol on the degranulation of RBL-2H3 cell is not a common feature among sterols.
Figure 3. Effect of sterols, ergosterol, cholesterol, β-sitosterol, and campesterol on β-hexosaminidase and histamine release in RBL-2H3.
IgE-sensitized RBL-2H3 cells pretreated with 50 μM of sterol or tranilast (Tra) were stimulated with DNP-HSA, and the release of β-hexosaminidase (A) and histamine (B) were measured. Each bar represents the mean ± SEM (n = 3). * p < 0.05, *** p < 0.001 vs. Control (Cont, DNP-HSA alone). Erg, ergosterol; Cho, cholesterol; Sit, β-sitosterol; Cam, campesterol.
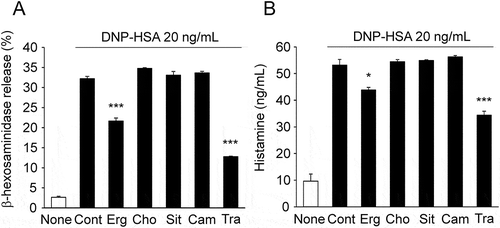
Ergosterol inhibits the antigen-induced production of cytokines from RBL-2H3 cells
We examined the effects of ergosterol on the antigen-induced production of the cytokines, IL-4 and TNF-α, from RBL-2H3 cells. The cells were stimulated with antigen in the presence or absence of ergosterol for 2 hours. Antigen stimulation increased the mRNA levels of IL-4 and TNF-α, and ergosterol at 20 and 50 μM significantly reduced the mRNA levels ().
Figure 4. Effects of ergosterol on antigen-induced increase in IL-4 and TNF-α mRNA expression.
IgE-sensitized RBL-2H3 cells pretreated with ergosterol (Erg) were stimulated with DNP-HSA. Two hours after antigen stimulation, total RNA was extracted and IL-4 (A), TNF-α (B), and GAPDH mRNA expression was measured by quantitative RT-PCR. Values are normalized to those of GAPDH mRNA and the mean value of the non-stimulated cells (None) was set as 1.0. Each bar represents the mean ± SEM (n = 4). * p < 0.05, ** p < 0.01, *** p < 0.001 vs. Control (Cont, DNP-HSA alone).
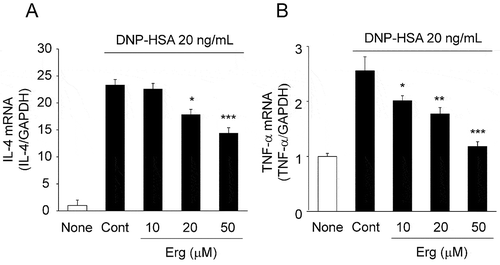
Ergosterol inhibits the antigen-induced aggregation of FcεRI
To investigate the mechanisms by which ergosterol inhibits the degranulation of mast cells, we examined the effect of ergosterol on the antigen-induced aggregation of FcεRI. The distribution of IgE-binding FcεRI on the cell surface was analyzed immunohistochemically. Antigen stimulation of RBL-2H3 cells for 20 minutes induced the aggregation of IgE-binding FcεRI. Pretreatment with 50 μM of ergosterol inhibited the antigen-induced aggregation of FcεRI without affecting the expression of FcεRI. Pretreatment with 1 mM of MβCD, which depletes cholesterol in membranes [Citation26], also inhibited the antigen-induced aggregation of FcεRI ().
Figure 5. Effect of ergosterol on the antigen-induced aggregation of FcεRI.
IgE-sensitized RBL-2H3 cells pretreated with ergosterol (Erg, 50 μM) or methyl beta cyclodextrin (MβCD, 1 mM) were stimulated with DNP-HSA. IgE/α-chain of FcεRI complexes were detected using anti-rat IgE antibody (A). The arrowhead shows the aggregation positive cells (A, lower panels). Data are represented as the number of aggregation positive cells/total cell number (B). Each bar represents the mean ± SEM (n = 6). *** p < 0.001 vs. Cont (Control, DNP-HSA alone).
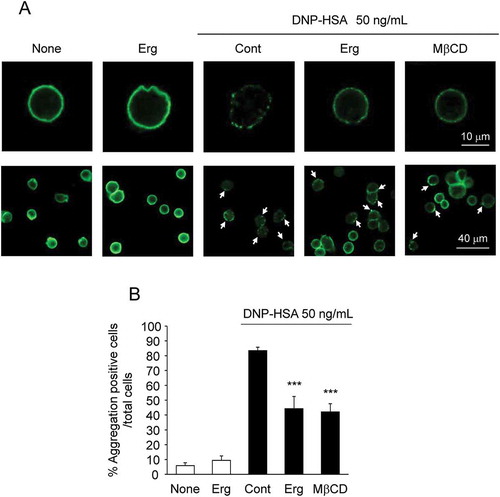
Ergosterol suppresses the antigen-induced tyrosine phosphorylation in RBL-2H3 cells
The aggregation of IgE-binding FcεRI by antigen induced the phosphorylation of tyrosine kinases as the initial step in signaling pathways. Antigen stimulation of RBL-2H3 cells induced the tyrosine phosphorylation of proteins at 72 kDa and 75 kDa with a maximum effect at 5 minutes. The tyrosine phosphorylations of these proteins were suppressed by pretreatment with ergosterol (, Supplemental Figure 1 and Supplemental Figure 2).
Figure 6. Effect of ergosterol on antigen-induced global tyrosine phosphorylation of proteins at 72 kDa and 75 kDa.
IgE-sensitized RBL-2H3 cells pretreated with or without ergosterol (Erg, 50 μM) were stimulated with DNP-HSA (50 ng/mL) for the indicated times. Cell lysates were blotted with anti-phosphotyrosine antibody. The arrowheads on the left side indicated the position of the molecular weight markers and the right side indicated the molecular weight of the target proteins calculated from the calibration curve obtained by the molecular weight markers.Grifola frondosa inhibits antigen-induced degranulation of RBL-2H3 cells, and its active components are ergosterol and its derivatives, MEDD and 6-OXO.
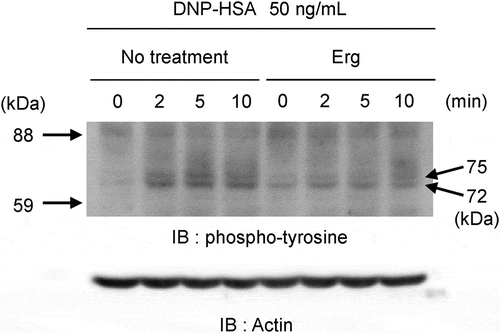
Discussion
In this study, we found that G. frondosa extract (GFE) inhibited degranulation in antigen-stimulated RBL-2H3 cells. Moreover, ergosterol and its derivatives, MEDD and 6-OXO isolated from G. frondosa, were identified as the anti-allergic components. Among the three active components, we focused on ergosterol, because its inhibitory effects were similar to its derivatives and because the ergosterol content, approximately 50 mg per 100 g of fresh fruiting body, was markedly higher than the other active components in G. frondosa. Furthermore, we estimated that ergosterol content is about 50% of GFE, because the majority of fraction 4 (642.1 mg) separated from GFE (1.23 g) was considered to be ergosterol by thin-layer chromatography (data not shown). Accordingly, in our results showed D and E, the effect of 50 μg/mL of GFE equal to 25 μg/mL of ergosterol was approximately the same as the effect of 50 μM (20 μg/mL) of ergosterol. Thus, ergosterol is considered as the main active substance in GFE, which inhibits degranulation on RBL-2H3 cells.
It was previously reported that ergosterol inhibited the degranulation of mucosal-type mast cells [Citation27]. However, the inhibitory mechanism of degranulation has not been clarified. Ergosterol is a characteristic sterol of fungi [Citation28], cholesterol is a sterol derived from animals, and β-sitosterol and campesterol are predominant sterols in plants [Citation29]. Interestingly only ergosterol, but not the other sterols, showed inhibitory effects on antigen-induced degranulation, suggesting that a small difference in the structure of ergosterol compared with the other sterols was required to exert its inhibitory activity.
Ergosterol suppressed the expression of IL-4 and TNF-α mRNA in antigen-stimulated RBL-2H3 cells as well as degranulation. Antigen-induced degranulation of mast cells causes acute allergic responses. In addition, IL-4 and TNF-α produced by mast cells play important roles in the late phase responses of allergic inflammation. IL-4 is a major Th2 cytokine responsible for IgE production and TNF-α is necessary for progression to chronic allergic inflammation [Citation30,Citation31]. Ergosterol significantly reduced IL-4 and TNF-α mRNA levels in antigen-stimulated RBL-2H3 cells, suggesting ergosterol exerts inhibitory actions on both anaphylactic responses and the progression of allergic disorders.
Antigen stimulation induces the aggregation of FcεRI, the first step in the activation of mast cells, which subsequently induces intracellular signal transduction, such as cytosolic Src protein-tyrosine kinases. Aggregated receptors and membrane signaling are localized in lipid raft domains [Citation32]. Cholesterol is abundant in lipid rafts and was reported to play a role in membrane signaling, including the degranulation of mast cells [Citation33,Citation34]. Therefore, ergosterol might modulate the aggregation of FcεRI induced by antigen by replacing cholesterol in lipid rafts, based on their structural similarities. Indeed, we found that the antigen-induced aggregation of FcεRI on RBL-2H3 cells was inhibited by ergosterol. In support of this, the antigen-induced tyrosine phosphorylation of proteins at 72 and 75 kDa, the smaller of which is assumed to be Syk (spleen tyrosine kinase), was suppressed by ergosterol. These results suggest that the inhibitory effect of ergosterol on allergic inflammation is mediated by suppressing the aggregation of FcεRI.
In the present study, we found that G. frondosa has anti-allergic effects and that its active components are ergosterol and its derivatives. Furthermore, we revealed that the inhibitory mechanism of ergosterol on allergic inflammation is mediated by suppressing the aggregation of FcεRI. Although it is known that orally administered ergosterol is absorbed from intestine in rats while many of plant sterols are barely absorbed [Citation35,Citation36], further studies are needed to investigate the kinetics of ergosterol and the clinical effectiveness in an in vivo model for use as functional food. Therefore, these results suggest that G. frondosa, including ergosterol and its derivatives as active compounds, may provide insights into the prevention or treatment of mast cell-dependent allergic diseases.
Author contributions
JK and KM are designed the study and wrote the manuscript. MH and NH contributed to the development of the manuscript. JK, KM and YH carried out experiments and analyzed the data.
Supplemental_figure_2.pdf
Download PDF (131 KB)Supplemental_figure_1.pdf
Download PDF (147 KB)Supplemental_table_3.pdf
Download PDF (38.9 KB)Supplemental_table_2.pdf
Download PDF (36 KB)Supplemental_table_1.pdf
Download PDF (36.3 KB)Disclosure statement
No potential conflict of interest was reported by the authors.
Additional information
Funding
References
- He X, Wang X, Fang J, et al Polysaccharides in Grifola frondosa mushroom and their health promoting properties: A review. Int. J. Biol. Macromol.. 2017;101:910–921.
- Deng G, Lin H, Seidman A, et al A phase I/II trial of a polysaccharide extract from Grifola frondosa (Maitake mushroom) in breast cancer patients: immunological effects. J. Cancer Res. Clin. Oncol.. 2009;135:1215–1221.
- Wesa KM, Cunningham-Rundles S, Klimek VM, et al Maitake mushroom extract in myelodysplastic syndromes (MDS): a phase II study. Cancer Immunol. Immunother.. 2014;64:237–247.
- Masuda Y, Nakayama Y, Tanaka A, et al Antitumor activity of orally administered maitake α-glucan by stimulating antitumor immune response in murine tumor. PLoS One.. 2017;12:1–15.
- Lee JS, Park SY, Thapa D, et al Grifola frondosa water extract alleviates intestinal inflammation by suppressing TNF-alpha production and its signaling. Exp. Mol. Med.. 2010;42:143–154.
- Shen KP, Su CH, Lu TM, et al Effects of Grifola frondosa non-polar bioactive components on high-fat diet fed and streptozotocin-induced hyperglycemic mice. Pharm. Biol.. 2015;53:705–709.
- Xiao C, Wu Q, Xie Y, et al Hypoglycemic effects of Grifola frondosa (Maitake) polysaccharides F2 and F3 through improvement of insulin resistance in diabetic rats. Food Funct.. 2015;6:3567–3575.
- Su CH, Lu TM, Lai MN, et al Inhibitory potential of Grifola frondosa bioactive fractions on α-amylase and α-glucosidase for management of hyperglycemia. Biotechnol. Appl. Biochem.. 2013;60:446–452.
- Tsao YW, Kuan YC, Wang JL, et al Characterization of a Novel Maitake (Grifola frondosa) Protein That Activates Natural Killer and Dendritic Cells and Enhances Antitumor Immunity in Mice. J. Agric. Food Chem.. 2013;61:9828–9838.
- Park HS, Hwang YH, Kim MK, et al Functional polysaccharides from Grifola frondosa aqueous extract inhibit atopic dermatitis-like skin lesions in NC/Nga mice. Biosci. Biotechnol. Biochem.. 2015;79:147–154.
- Burton OT, Oettgen HC. Beyond immediate hypersensitivity: evolving roles for IgE antibodies in immune homeostasis and allergic diseases. Immunol Rev.. 2012;242:128–143.
- Wedemeyer J, Galli SJ. Mast cells and basophils in acquired immunity. Br. Med. Bull.. 2000;56:936–955.
- Galli SJ, Ig TM. E and mast cells in allergic disease. Nat. Med.. 2012;18:693–704.
- Gilfillan AM, Tkaczyk C. Integrated signalling pathways for mast-cell activation. Nat. Rev. Immunol.. 2006;6:218–230.
- Halova I, Draberova L, Draber P. Mast cell chemotaxis - chemoattractants and signaling pathways. Front. Immunol.. 2012;3:119.
- Wernersson S, Pejler G. Mast cell secretory granules: armed for battle. Nat. Rev. Immunol.. 2014;14:478–494.
- Itoh T, Tsukane M, Koike M, et al Inhibitory effects of whisky congeners on IgE-mediated degranulation in rat basophilic leukemia RBL-2H3 cells and passive cutaneous anaphylaxis reaction in mice. J. Agric. Food Chem.. 2010;58:7149–7157.
- Kanda T, Akiyama H, Yanagida A, et al Inhibitory Effects of Apple Polyphenol on Induced Histamine Release from RBL-2H3 Cells and Rat Mast Cells. Bioscience, Biotechnology, and Biochemistry. 1998;62:1284–1289.
- Han S, Sun L, He F, et al Anti-allergic activity of glycyrrhizic acid on IgE-mediated allergic reaction by regulation of allergy-related immune cells. Sci. Rep.. 2017;7:7222.
- Tanaka M, Suzuki M, Takei Y, et al Cirsium maritimum Makino Inhibits the Antigen/Immunoglobulin-E-Mediated Allergic Response In Vitro and In Vivo. J. Agric. Food Chem.. 2017;65:8386–8391.
- Shore PA, Burkhalter A, Jr CVH. A method for the fluorometric assay of histamine in tissues. J Pharmacol Exp Ther.. 1959;127:182–186.
- Kwon HC, Zee SD, Cho SY, et al Cytotoxic ergosterols from Paecilomyces sp. J300. Arch. Pharm. Res.. 2002;25:851–855.
- Kawagishi H, Katsumi R, Sazawa T, et al Cytotoxic steroids from the mushroom Agaricus Blazei. Phytochemistry.. 1988;27:2777–2779.
- Yang SP, Xu J, Yue JM. Sterols from the Fungus Catathelasma imperiale. Chinese J. Chem.. 2003;21:1390–1394.
- Ishizuka T, Yaoita Y, Sterol KM. Constituents from the fruit bodies of Grifola frondosa (FR. S. F. Gray. Chem. Pharm. Bull.. 1997;45:1756–1760.
- Horejsi V. Membrane rafts in immunoreceptor signaling: new doubts, new proofs?. Trends Immunol. 2002;23:562–564.
- Kageyama-Yahara N, Wang P, Wang X, et al The inhibitory effect of ergosterol, a bioactive constituent of a traditional Japanese herbal medicine saireito on the activity of mucosal-type mast cells. Biol. Pharm. Bull.. 2010;33:142–145.
- Chen DQ, An JM, Feng YL, et al Cloud-point extraction combined with liquid chromatography for the determination of ergosterol, a natural product with diuretic activity, in rat plasma, urine, and faeces. J. Anal. Methods Chem.. 2013;2013:479056.
- Valitova JN, Sulkarnayeva AG, Minibayeva FV. Plant sterols: diversity, biosynthesis, and physiological functions. Biochemistry. (Moscow). 2016;81:819–834.
- Hirasawa N, Kagechika H, Shudo K, et al Inhibition by retinoids of antigen-induced IL-4 production in rat mast cell line RBL-2H3. Life Sci. 2001;68:1287–1294.
- Ye J, Piao H, Jiang J, et al Polydatin inhibits mast cell-mediated allergic inflammation by targeting PI3K/Akt, MAPK, NF-κB and Nrf2/HO-1 pathways. Sci. Rep. 2017;7:11895.
- Lingwood D, Simons K. Lipid rafts as a membrane-organizing principle. Science. 2010;327:46–50.
- Derler I, Jardin I, Stathopulos PB, et al Cholesterol modulates Orai1 channel function. Sci Signal. 2016;9:ra10.
- Kovarova M, Wassif CA, Odom S, et al Cholesterol deficiency in a mouse model of Smith-Lemli-Opitz syndrome reveals increased mast cell responsiveness. J. Exp. Med.. 2006;203:1161–1171.
- Zhao YY, Cheng XL, Liu R, et al Pharmacokinetics of ergosterol in rats using rapid resolution liquid chromatography–atmospheric pressure chemical ionization multi-stage tandem mass spectrometry and rapid resolution liquid chromatography/tandem mass spectrometry. J. Chromatogr. B. 2011;879:1945–1953.
- Katan MB, Grundy SM, Jones P, et al Efficacy and Safety of Plant Stanols and Sterols in the Management of Blood Cholesterol Levels. Mayo Clin. Proc. 2003;78:965–978.