ABSTRACT
Chitinase-A from a lycophyte Selaginella doederleinii (SdChiA), having molecular mass of 53 kDa, was purified to homogeneity by column chromatography. The cDNA encoding SdChiA was cloned by rapid amplification of cDNA ends and polymerase chain reaction. It consisted of 1477 nucleotides and its open reading frame encoded a polypeptide of 467 amino acid residues. The deduced amino acid sequence indicated that SdChiA consisted of two N-terminal chitin-binding domains and a C-terminal plant class V chitinase catalytic domain, belonging to the carbohydrate-binding module family 18 (CBM18) and glycoside hydrolase family 18 (GH18), respectively. SdChiA had chitin-binding ability. The time-dependent cleavage pattern of (GlcNAc)4 by SdChiA showed that SdChiA specifically recognizes the β-anomer in the + 2 subsite of the substrate (GlcNAc)4 and cleaves the glycoside bond at the center of the substrate. This is the first report of the occurrence of a family 18 chitinase containing CBM18 chitin-binding domains.
Abbreviations: AtChiC: Arabidopsis thaliana class V chitinase; CBB: Coomassie brilliant blue R250; CBM: carbohydrate binding module family; CrChi-A: Cycas revolute chitinase-A; EaChiA: Equisetum arvense chitinase-A; GH: glycoside hydrolase family, GlxChi-B: gazyumaru latex chitinase-B; GlcNAc: N-acetylglucosamine; HPLC: high performance liquid chromatography; LysM; lysin motif; MtNFH1: Medicago truncatula ecotypes R108-1 chitinase; NCBI: national center for biotechnology information; NF: nodulation factor; NtChiV: Nicotiana tabacum class V chitinase; PCR: polymerase chain reaction; PrChi-A: Pteris ryukyuensis chitinase-A; RACE: rapid amplification of cDNA ends; SDS-PAGE: sodium dodecyl sulfate-polyacrylamide gel electrophoresis; SdChiA: Selaginella doederleinii chitinase-A.
Graphical Abstract
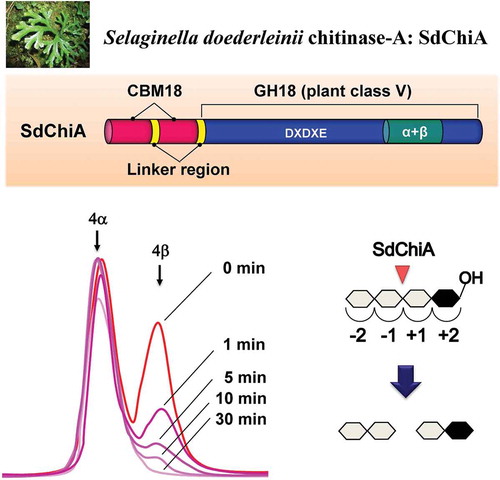
FeF3G6″RhaT catalyzes rutin biosynthesis in buckwheat, which is expressed in rutin-accumulating organs during early developmental stages.
Chitinases (EC 3.2.1.14) catalyze the hydrolysis of chitin, which is a β-1,4-linked homopolymer or oligomer of N-acetylglucosamine (GlcNAc). Many plants possess various chitinases [Citation1,Citation2], but an endogenous substrate for plant chitinases has not yet been found. They may be involved in the interaction between plants and microbes, which produce chitin and chitin-related compounds. It has been reported that one of the physiological roles of these chitinases is to protect plants against fungal pathogens by degrading chitin, a major component of the cell wall of many fungi [Citation3,Citation4]. However, some chitinases do not show any antifungal activity [Citation5,Citation6]. Apart from being induced by infection with fungal pathogens, plant chitinases are also induced by abiotic stress and symbiotic interactions [Citation7–Citation10].
According to the CAZy database (http://www.cazy.org/), plant chitinases belong to the glycoside hydrolase family 18 (GH18) and 19 (GH19), based on the structure of their catalytic domains. Additionally, plant chitinases are divided into five classes [Citation1,Citation11,Citation12], and two subclasses [Citation13,Citation14], based on their amino acid sequences and domain architectures. Class I, II, II-L, and IV chitinases belong to GH19, and class III, IIIb, and V chitinases belong to GH18. Class I chitinases consist of an N-terminal chitin-binding domain and a catalytic domain, whereas class II chitinases have only a catalytic domain homologous to that of class I chitinases. The chitin-binding domain, belonging to the carbohydrate-binding module family 18 (CBM18), has homology to hevein and wheat germ agglutinin [Citation15]. Class IV chitinases share homology with class I chitinases but are smaller due to several deletions [Citation1]. Class II-L chitinases are a subclass of class II chitinases but are smaller due to the deletion of several loops [Citation16]. Class III, IIIb, and V chitinases share the consensus motif DxDxE around the catalytic cleft and the (β/α)8 barrel structure; however, their amino acid sequences only share a 10–15% homology. Class III chitinases are the major GH18 chitinases with three conserved disulfide bonds. A minor subset of these, Class IIIb chitinases, have no disulfide bonds and possess a different chitin oligosaccharide degradation pattern from that of class III chitinases [Citation13,Citation17]. Additionally, distinguishing class IIIb chitinases are two large loops that extrude from the catalytic site, as revealed in the crystal structure of the catalytic domain of the class IIIb chitinase PrChi-A, from Pteris ryukyuensis [Citation18]. Class V chitinases also have a different chitin oligosaccharide degradation pattern, and a long insertion of about 70 amino acids compared to class III and class IIIb chitinases [Citation19,Citation20]. With the exception of two class IIIb chitinases from fern plants with N-terminal CBM50 [lysin motif (LysM)] domain(s) [Citation21,Citation22], plant GH18 chitinases only consist of a catalytic domain.
We consider that this diversity in the structure and roles of plant chitinases has developed over the course of plant evolution. Therefore, we focused on the chitinases in different plants from various evolutionary lineages. In the present study, we extended this work to lycophytes. Vascular plants are classified into two lineages, euphyllophytes and lycophytes. Lycophytes, consisting of three families (clubmosses, quillworts, and spikemosses), are the closest to the common ancestor of vascular plants, and their evolution has been the subject of considerable interest [Citation23,Citation24]. Therefore, we expected to obtain interesting findings about the molecular evolution and diverse physiological roles of plant chitinases by examining them in lycophytes. We began by screening various lycophytes for chitinase activity. Selaginella doederleinii, belonging to the spikemoss lineage, showed a high chitinase activity relative to the other lycophytes assayed. In our study, we describe the successful isolation, characterization, and cDNA cloning of chitinase from S. doederleinii. Sequence analysis showed the novelty of this chitinase, which is designated as S. doederleinii chitinase-A (SdChiA). It consists of two N-terminal CBM18 domains and a catalytic domain of class V chitinase. Our chitin-binding assay clearly showed that SdChiA has a stronger chitin-binding activity than the class I chitinase from Ficus microcarpa (GlxChi-B) and the class V chitinase from Cycas revolute (CrChi-A). It has been reported that the CBM18 chitin-binding domain is present only in plant GH19 chitinases [Citation14]. Therefore, this is the first report of a plant GH18 chitinase containing CBM18 chitin-binding domains.
Materials and methods
Materials
S. doederleinii was collected from the Yona field of the campus of Ryukyu University, Okinawa, Japan. Powdered chitin and N-acetyl-d-glucosamine (GlcNAc) oligomers were purchased from Seikagaku Co. (Tokyo, Japan). The class I chitinase from Ficus microcarpa (GlxChi-B) and class V chitinase from Cycas revoluta (CrChi-A) were prepared as described by Taira et al [Citation6,Citation19]. All other reagents were of analytic grade.
Protein measurement
Protein concentrations were measured by the bicinchoninic acid method [Citation25], using bovine serum albumin as the standard.
Electrophoresis
SDS-PAGE was performed as described [Citation26], using 12.5% acrylamide gel. Proteins on the gel were stained with Coomassie brilliant blue R250 (CBB). Molecular mass was measured in the presence of 2-mercaptoethanol using a PageRuler Unstained Protein Ladder (Thermo Fisher scientific, Japan).
Assay of chitinase activity
Chitinase activity was assayed colorimetrically using glycol chitin. Ten microliters of the sample solution were added to 250 µL of 0.2% (w/v) glycol chitin in 0.1 M glycine-HCl buffer, pH 3.0. After incubation at 37°C for 15 min, the reducing power of the reaction mixture was measured using ferri-ferrocyanide reagent, as described by Imoto and Yagishita [Citation27]. The chitinase activity of each chromatographic fraction was expressed as absorbance of the reaction mixture at 420 nm (∆A420). One unit of enzyme activity was defined as the amount of enzyme releasing one µmol of GlcNAc per min at 37°C.
Purification of chitinase from S. doederleinii
All procedures were performed in a cold room (4°C). Absorbance was measured at 280 nm to monitor proteins, and chitinase activity was measured at pH 3.0 using glycol chitin during chromatographic separation. S. doederleinii (200 g) was homogenized with 1000 mL of deionized water and then left for 4 h at 4°C. After centrifugation, the supernatant was collected as crude chitinase. The crude chitinase was then mixed with an equal volume of 3 M ammonium sulfate solution and blended with 10 mL of Butyl-Toyopearl 650M resin. After overnight incubation at 4°C, the resin containing the adsorbed proteins was collected with a glass filter, and then packed into a column (1.6 × 5 cm). The adsorbed proteins were eluted with 50 mM sodium acetate buffer, pH 5.0. The fractions containing chitinase activity were pooled, loaded onto a Sephadex G-75 column previously washed with 10 mM sodium acetate buffer, pH 5.0, and developed using the same buffer. To concentrate the fractions containing chitinase activity, these fractions were mixed with an equal volume of 3 M ammonium sulfate solution, and then loaded onto the Butyl-Toyopearl 650M column (5 mL). The adsorbed proteins were eluted with 50 mM sodium acetate buffer, pH 5.0. The fractions containing chitinase activity were dialyzed against 10 mM sodium acetate buffer (pH 5.0), and then loaded onto a Mono-Q column equilibrated with the dialysis buffer. The column was washed with the same buffer and the adsorbed proteins were eluted with a linear gradient of NaCl from 0 to 0.3 M in the same buffer. The fractions containing chitinase activity were pooled and mixed with a half volume of 3 M ammonium sulfate in 60 mM sodium acetate buffer. The mixture was loaded onto a Phenyl Superose column (0.5 × 5 cm) equilibrated with 20 mM sodium acetate buffer, pH 5.0. The column was washed with the same buffer, including 1 M ammonium sulfate, and then, the adsorbed proteins were eluted with a linear gradient of ammonium sulfate from 1.0 to 0 M. The purified SdChiA fraction showed a strong band with a molecular mass of 53 kDa, and other minor bands on the SDS-polyacrylamide gel electrophoresis (PAGE) (, lane 1). We judged that these minor bands would not affect the experimental results. Two hundred grams of S. doederleinii yielded 0.3 mg of SdChiA.
Internal amino acid sequence analysis
To obtain internal peptides, in-gel digestion was performed as described [Citation28]. Five micrograms of purified SdChiA were loaded onto an SDS-PAGE well and 0.5 µg of V8 protease was then added to the same well. The SdChiA and protease were electrophoresed to the interface between the stacking and separating gels, and the process was then stopped. After 60 min, the electrophoresis was restarted and completed. The digested products were blotted onto a PVDF membrane and detected with CBB staining. To obtain internal sequences of SdChiA, the peptide bands on the membrane were analyzed using a protein sequencer.
HPLC analysis of the hydrolysis products of chitin oligosaccharide [(glcnac)n] by chitinase
The products of (GlcNAc)n (n = 4, 5, 6) hydrolysis by chitinase were analyzed using the HPLC with TSKgel amide-80 column (Tosoh, 0.46 × 25 cm) using the method of Koga et al [Citation29]. The enzymatic reaction was carried out with 20 pmol of chitinase and 10 nmol of (GlcNAc)n in 100µl of 4 mM glycine-HCl buffer, pH 3.0 for the given time-period at 25°C, and the reaction mixture was immediately cooled in an ice bath. 5 µL of this mixture was then loaded onto the column. To suppress the mutarotation of the reaction products, the enzymatic reaction was conducted at a lower temperature (25°C). The hydrolysis products were eluted with 70% acetonitrile at a flow rate of 0.7 mL/min. The substrate and enzymatic products were detected by ultraviolet absorption at 210 nm.
Cdna cloning
The sequences of all primers are presented in . Total RNA was isolated from S. doederleinii using a RNeasy kit (Qiagen, Valencia, CA, USA). First-strand cDNA synthesis was performed on 5 µg of total RNA using a GeneRacer kit (Invitrogen, Carlsbad, CA, USA) with an oligo(dT) adapter primer. The resulting cDNA was used as a template for polymerase chain reaction (PCR) amplification with degenerate primers. The first PCR was performed with primers P1 (a forward primer designed based on the sequence encoding class V chitinases from Selaginella moellendorffii) and P3 (a reverse primer designed based on an internal peptide sequence of purified SdChiA), and nested PCR with P2 (a forward primer designed on the basis of the sequence encoding class V chitinases from S. moellendorffii) and P4 (a reverse primer designed on the basis of an internal peptide sequence of purified SdChiA). The resulting PCR products were cloned into a pGEM-T vector and sequenced using the ABI Prism 3100 Genetic Analyzer (Applied Biosystems, CA, USA). To obtain the full-length cDNA of SdChiA, both 5′ and 3′ rapid amplification of cDNA ends (RACE) were performed using a GeneRacer kit according to the manufacturer’s instructions. The gene-specific primers P5 (first PCR) and P6 (nested PCR) were used for the 5′ RACE and the gene-specific primers P7 (first PCR) and P8 (nested PCR) for the 3′ RACE. Finally, a cDNA fragment containing the entire coding region of SdChiA cDNA was amplified using the forward primer P9 (designed from the 5′ RACE products from the first PCR), P10 (nested PCR), reverse primer P11 (designed from the 3′ RACE products from the first PCR), and P12 (nested PCR). The sequences of the resulting PCR products were analyzed using the afore-mentioned procedure.
Table 1. Primers for PCR and RACE.
Chitin-binding assay
The chitin-binding ability of chitinase was examined using chitin powder with a 105-µm particle size (Wako, Japan). The chitin powder was washed successively with 1 M NaOH, then neutralized with 1 M HCl, and finally washed with 1 M NaCl before use. Two micrograms of the chitinase were mixed with 10% chitin solution equilibrated with 100 µL of 0.15 M NaCl in 10 mM Tris-HCl, pH 8.0 (TBS buffer). After incubation for 60 min at 4°C, the mixture was centrifuged, and then, the supernatant was collected as the un-adsorbed fractions. Subsequently, the remaining un-adsorbed protein was washed out with the same buffer and the adsorbed proteins were eluted with the sample buffer [0.1 M Tris-HCl (pH 6.8) with 2% SDS, 0.1% BPB, 10% glycerol, 5% 2-mercaptoethanol]. The adsorbed and un-adsorbed proteins were subjected to SDS-PAGE, after which the proteins were detected by CBB staining. For comparison, the class I chitinase GlxChi-B and class V chitinase CrChi-A were also examined using the afore-mentioned procedures.
Results
Properties and internal amino acid sequence of purified S. doederleinii chitinase-a
The enzyme was purified to homogeneity by a series of column chromatography purifications. Using SDS-PAGE in the presence of 2-mercaptoethanol, the molecular mass of purified SdChiA was found to be 53 kDa (, lane 2). The optimum pH and the optimum temperature for SdChiA were pH 3 and 50–60°C, respectively, using glycol chitin as a substrate (), )).
Figure 2. Effect of pH and temperature on the chitinase activity of SdChi-A.
The effect of pH (a) and temperature (b) on activity was examined after incubation at 37°C for 15 min. Methods are described in Materials and Methods.
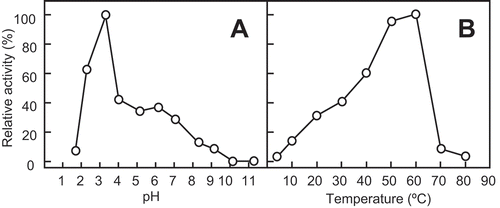
The cleavage patterns of (GlcNAc)n produced by SdChiA and the anomeric forms of the products are presented in . The major hydrolysis products from (GlcNAc)6 were (GlcNAc)4, (GlcNAc)3, and (GlcNAc)2, and those from (GlcNAc)5 were mainly (GlcNAc)3 and (GlcNAc)2. (GlcNAc)4 was mainly hydrolyzed to (GlcNAc)2. The newly created reducing ends of the products were largely β-anomers, indicating that SdChiA is a retaining enzyme. From these cleavage patterns of (GlcNAc)4–6, the subsite structure of SdChiA might be ‒2, ‒1, + 1, + 2, + 3, + 4 or ‒3, ‒2, ‒1, + 1, + 2, + 3 ()). In the case of (GlcNAc)4 hydrolysis by SdChiA, the initial substrate β-(GlcNAc)4 was specifically degraded and β-(GlcNAc)2 was mainly produced. The time-dependent cleavage pattern of this reaction showed that the quantity of (GlcNAc)4 β-anomer clearly decreased and that of (GlcNAc)2 considerably increased with time (). These results indicate that SdChiA specifically recognizes β-anomers in the + 2 subsite of (GlcNAc)4.
Figure 3. HPLC analysis of the hydrolysis products of (GlcNAc)n by SdChiA.
S, Standards (GlcNAc)1–6. (a) hydrolysis products of (GlcNAc)6; (b) hydrolysis products of (GlcNAc)5; (c) hydrolysis products of (GlcNAc)4. I to VI, α and β indicates (GlcNAc)1–6, α- and β- anomer, respectively. Standard (GlcNAc)1–6 and the reaction mixtures were analyzed by HPLC on a TSKgel amide-80 column as described in Materials and Methods. (d) Schematic representation of the substrate binding mode in the subsites. (GlcNAc) are shown as hexagon. The reducing-end sugars are filled in black or gray. The arrows indicate the cleavage site.
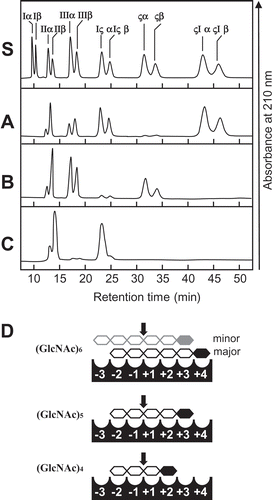
Cloning and sequence analysis of sdchia cdna
The sequences of all primers are presented in . The strategy used for the cloning of SdChiA cDNA is given in ). A 395-bp putative SdChiA PCR product was obtained using degenerate primers based on the internal peptide amino acid sequence (AVYYTNPQPSXMGGA; sequence used for primer design underlined) obtained by V8 protease digestion of purified SdChiA, and a relatively conserved amino acid sequence (THLLYAFV) among plant class V chitinases. Using gene-specific primers based on the sequence of the PCR product, a 577-bp 5′-cDNA fragment and an 812-bp 3′-cDNA fragment were amplified by RACE and sequenced. The resulting full-length SdChiA cDNA consisted of 1477 nucleotides and encoded an open-reading frame of 467 amino acid residues ()). Analysis using the computer program SignalP (http://www.cbs.dtu.dk/services/SignalP/) [Citation30] indicated that the N-terminal sequence consisting of 22 amino acids (Met1 to Ala22) is a putative signal peptide for transportation to the endoplasmic reticulum. The remaining 445 amino acids (Gln23 to Lys467) were considered to constitute the SdChiA mature protein.
Figure 5. Cloning strategy and primary structure of SdChiA.
(a) Cloning strategy of SdChiA cDNA. Three fragments overlapped to obtain the complete nucleotide sequence of SdChiA cDNA. The open box represents the coding region and solid lines represent the 5′- and 3′-noncoding regions. The relative position and direction of each primer are indicated by arrows. The solid lines indicate cDNA fragments amplified by each PCR. SP, signal peptide. (b) Nucleotide sequence of SdChiA cDNA with its deduced amino acid sequence. The putative signal peptide at the N-terminal is indicated by a dotted underline. Internal peptide obtained by V8 protease digestion are underlined. (c) Schematic representation of SdChiA. CBD, chitin-binding domain; GH, glycoside hydrolase.
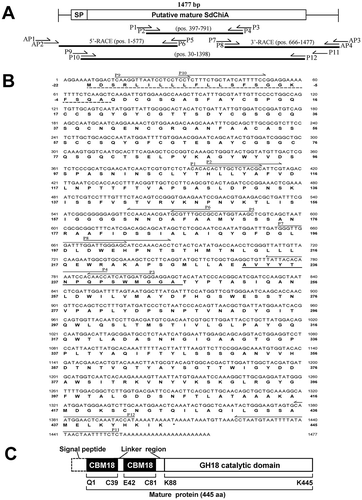
A search of the deduced amino acid sequence of SdChiA against the conserved domains database (CDD) (http://www.ncbi.nlm.nih.gov/Structure/cdd/cdd.shtml) [Citation31] showed that it possessed two CBM18 domains at the N-terminal end and a GH18 domain at the C-terminal end ()). Multiple alignment of CBM18 domains from SdChiA, CBM18 domains from other chitinases, and hevein were performed using the ClustalX software [Citation32], and the results are shown in ). Two CBM18 domains in SdChiA (SdChiA 1st and SdChiA 2nd) are 70% homologous to each other. SdChiA 1st and 2nd have a high level of homology to CBM18 domains from plant class I chitinases (Ananas comosus, 73% and 59% identity, respectively; Secale cereale, 64% and 57%, respectively; Oryza sativa, 61% and 57%, respectively; Hevea brasiliensis, 48% in both cases). Structure-based sequence alignments of the GH18 domain of SdChiA with GH18 chitinases from plants and mammals are shown in ). The GH18 domain of SdChiA had relatively high levels of sequence homology with class V chitinases from C. revoluta (43%), Nicotiana tabacum (41%), and Arabidopsis thaliana (43%), and moderate levels of homology with human chitotriosidase (30%). However, the GH18 domain of SdChiA showed very low sequence homology with plant class III and class IIIb chitinases from Hevamine (17%), Ananas comosus (17%), and Pteris ryukyuensis (14%). In addition, the GH18 domain of SdChiA has a putative extra α/β domain region, which is shared in class V chitinases, but not found in class III and class IIIb chitinases. Thus, SdChiA consists of two N-terminal CBM18 domains and a C-terminal GH18 domain that belong to the group of plant class V chitinases. No other chitinase consisting of this domain combination is known among the plant chitinases.
Figure 6. Alignment of amino acid sequences from domains of SdChiA with those from corresponding regions of several proteins.
(a) Alignment of sequences from chitin-binding domains of SdChiA with those of chitin-binding domain containing proteins. (b) Structure-based sequence alignments of catalytic domain among SdChiA, plant class V chitinases, human chitotriosidase, plant class III chitinases and plant class IIIb chitinase. Identical residues are shown in white and black background; dashes indicate gaps. Zigzag bold lines and arrows indicate α- and β- strands, respectively. The strands shown on the top of sequences are the secondary structures of SdChiA predicted by a computer program (PSIPRED, http://bioinf.cs.ucl.ac.uk/psipred/). The strands at the bottom of the sequences are secondary structures of hevamine. The eight β-strands and α-helixes in the TIM barrel are indicated and labeled. A box indicates a region of the putative extra α/β domain. The catalytic glutamic acid in the DXDXE motif is indicated by a triangle. SdChiA, S. doederleinii chitinase-A; CrChi-A, plant class V chitinase from Cycas revolta (accession no. BAD98525.); NtChiV, plant class V chitinase from Nicotiana tabacum (CAA54374); AtChiC, plant class V chitinase from Arabidopsis thaliana (NP_193716); Chitotriosidase, chitinase from Homo sapiens (AAG10644); Hevamine, plant class III chitinase from Hevea brasiliensis (P23472); PLChi-A, plant class III chitinase from Ananas comosus (BAG38685); PrChi-A, plant class IIIb chitinase from Pteris ryukyuensis (BAE98134).
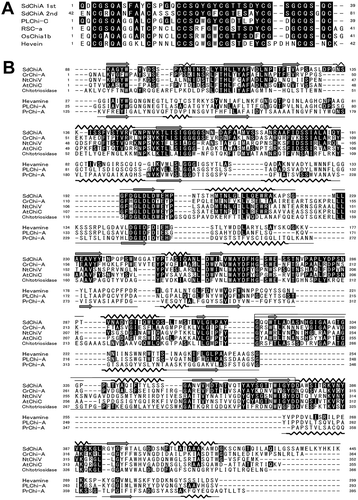
Chitin-binding activity of sdchia
Using chitin equilibrated with 100 µL of TBS buffer, we examined the chitin-binding activities of SdChiA, GlxChi-B, and CrChi-A. GlxChi-B consists of an N-terminal CBM18 chitin-binding domain and a C-terminal GH19 domain with homology to plant class I chitinases. CrChi-A only has a GH18 domain homologous to class V chitinase. These three proteins applied to this experiment are shown in ). After incubation and centrifugation, the adsorbed and un-adsorbed fractions were subjected to SDS-PAGE, and the gel was then stained with CBB ()). SdChiA and a part of GlxChi-B were found in the adsorbed fraction, while CrChi-A was found only in the un-adsorbed fraction. These results indicate that SdChi-A possesses a stronger chitin-binding ability than GlxChi-B or CrChi-A. It seems likely that the two CBM18 domains contribute to the strong chitin-binding ability of SdChiA.
Figure 7. Chitin-binding activity of SdChiA, GlxChi-B and CrChi-A.
(a) SDS-PAGE of three proteins (each 2 µg) applied to the chitin-binding assay. The gel was stained with CBB. Lane M, marker proteins; lane 1, SdChiA (CBM18 × 2+ GH18); lane 2, GlxChi-B (CBM18+ GH19); lane 3, CrChi-A (GH18). (b) The proteins were applied to chitin powders equilibrated with 0.15 M NaCl in 10 mM Tris-HCl. After incubation and centrifugation, the adsorbed and unadsorbed fractions were subjected to SDS-PAGE, and then stained with CBB. Lane M, maker proteins; lane N, the unadsorbed fraction; lane B, the adsorbed fraction.
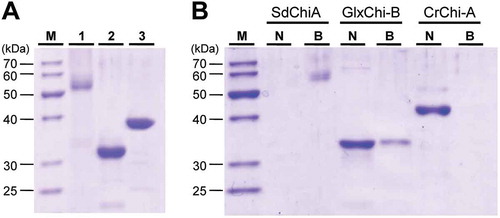
Discussion
In this study, we demonstrate the existence of a new type of chitinase, SdChiA, found in a spikemoss, which is a lycophyte. This new type of chitinase consists of two N-terminal CBM18 domains, and a C-terminal GH18 domain with homology to plant class V chitinase ()). Plant chitinases are divided into five classes and two subclasses; several unclassified minor chitinases have also been reported (), )). Plant class V chitinases consist of only one catalytic domain, according to reports from C. revoluta (CrChi-A), N. tabacum (NtChiV), and A. thaliana (AtChiC) [Citation12,Citation19,Citation33]. Furthermore, plant class III and IIIb chitinases belonging to GH18 also consist of only one catalytic domain, except for PrChi-A from P. ryukyuensis and EaChiA from Equisetum arvense. PrChi-A and EaChiA consist of CBM50 (LysM) domain(s) and a GH18 domain with homology to class IIIb chitinases [Citation21,Citation22] ()). Chitinase from Brassica juncea, BjCHI1, is also unclassified, and consists of two N-terminal CBM18 chitin-binding domains and a GH19 domain [Citation34] ()).
Figure 8. Classification of plant chitinases.
(a) Schematic representation of five classes (I-V) and two subclasses (II-L and IIIb) of chitinases. (b) Schematic representation of unclassified plant chitinases. BjCHI1, Brassica juncea chitinase-1 (AAF02299); PrChi-A, P. ryukyuensis chitinase-A (BAE98134); EaChi-A, E. arvense chitinase-A (BAI22848); SdChiA, S. doederleinii chitinases-A. GH and CBM indicate glycoside hydrolase and carbohydrate binding module, respectively.
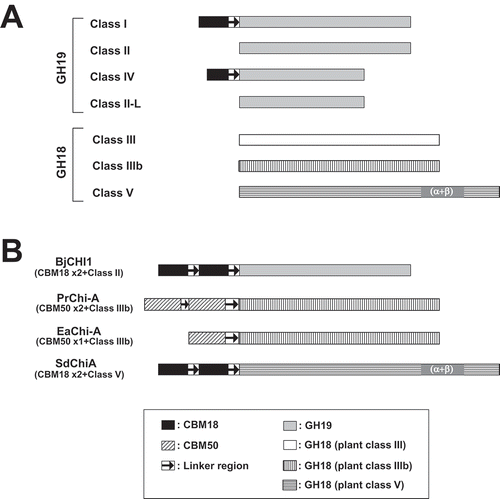
The first seedless vascular plant to have its whole genome sequenced was the lycophyte S. moellendorffii [Citation24]. According to the web database at the national center for biotechnology information (NCBI), there are eight kinds of GH18 chitinase-like proteins in S. moellendorffii, but GH18 chitinases containing CBM domains have not been found. They are also absent from other available plant sequence data. Thus, the present study is the first report of a plant GH18 chitinase with two extra CBM18 domains.
There are many reports that plant GH19 chitinases have antifungal activity, while reports of GH18 chitinases exhibiting antifungal activities are limited [Citation14]. NtChiV (GH18; plant class V) exhibited antifungal activity, but its level of activity is much lower than that of rye seed chitinase-a (CBM18+ GH19; plant class I) [Citation35]. SdChiA is a novel type of plant class V chitinase that possesses two extra CBM18 domains. CrChiA only has a GH18 domain homologous to class V chitinase, while GlxChi-B consists of an N-terminal CBM18 domain and a C-terminal GH19 domain homologous to class I chitinase. GlxChi-B has a strong antifungal activity, but CrChi-A does not [Citation6,Citation19]. Interesting questions for future work are whether and how the two chitin-binding domains of SdChiA influence antifungal activity. Our current research is focused on measuring the antifungal activity of SdChiA.
SdChiA catalyzes the hydrolysis of (GlcNAc)6 into (GlcNAc)4, (GlcNAc)3, and (GlcNAc)2, and the hydrolysis of (GlcNAc)5, mainly into (GlcNAc)3 and (GlcNAc)2 (), )). These cleavage patterns are similar to those produced by other plant class V chitinases, for example, CrChi-A, NtChiV, and AtChiC [Citation19,Citation20,Citation36]. In the case of (GlcNAc)4, SdChiA specifically recognizes β-(GlcNAc)4, and mainly produces β-(GlcNAc)2 (). The produced β-(GlcNAc)2 was slowly converted to α-(GlcNAc)2 by mutarotation with passage of time (). However, the initial substrate α-(GlcNAc)4 did not decrease until 10 minutes after the hydrolysis reaction (,)), and it slightly decreased after 10 minutes ()). From these results, we consider that the reaction rate of β-(GlcNAc)4-specific hydrolysis is more rapid than the conversion rate of α-(GlcNAc)4 to β-(GlcNAc)4 by mutarotation. NtChiV also preferentially hydrolyzes the β-anomer of (GlcNAc)4 and (GlcNAc)5 [Citation36,Citation37]. Based on the crystal structure of the inactive mutant NtChiV complexed with (GlcNAc)4, and anomer analysis of the hydrolysis products by the mutant W190A, it was suggested that the Trp190 of NtChiV in the substrate-binding cleft contributed to the specific recognition of the β-anomer + 2 subsite [Citation37]. Trp271 in SdChiA is corresponding to the residue. However, the tryptophan residue corresponding to Trp190 of NtChiV is also highly conserved in other plant class V chitinases without β-anomer selectivity ()). Therefore, it is unlikely that Trp190 of NtChiV directly recognizes the β-anomer. Umemoto et al [Citation37] suggested that the Trp190 of NtChiV stably bound to the + 2 sugar by face-to-face stacking interaction. We speculate there is another amino acid residue that specifically recognizes β-anomers. Fixing the orientation of + 2 sugar ring via face-to-face stacking interaction with Trp190 of NtChiV may cause its hydroxyl residue on C1 of the sugar to be correctly presented to the amino acid residue which specifically recognizes β-anomers.
The symbiosis-related class V chitinase MtNFH1, from Medicago truncatula ecotype R108-1, lacks chitinase activity but can hydrolyze nodulation factors (NFs), modified chitin oligosaccharides with a fatty acid moiety [Citation38]. According to homology modeling and docking simulation, formation of the substrate-binding cleft differs between MtNFH1 and class V chitinases AtChiC and NtChiV for substrates NF and (GlcNAc)5. We speculate that there is a specific substrate for SdChiA, which may be a derivative of chitin. For example, NFs, which are not only rhizobial, but also mycorrhizal signaling molecules, might be a candidate for substrates. NFs are β-1,4-linked oligomers of GlcNAc having several modifications at the reducing and/or non-reducing end residues [Citation39]. SdChiA may catalyze specific NFs, which attain a stable β-anomeric form by some modification(s).
To address the physiological functions of SdChiA, it is necessary to investigate its synthesis stage and response to various hormones and stresses. To elucidate the mechanism of β-anomer-specific recognition and to search for specific substrates, the structural study of SdChiA is needed.
Author Contribution
TTai conceived and designed research. YK performed experiments. TTai, YK, TTak and KU conducted experiments and contributed to data elaboration. TTai and YK wrote the manuscript. All authors read and approved the manuscript.
Acknowledgments
The authors thank Chihiro Kato and Kentaro Kinjo, University of the Ryukyus, for technical assistance. All authors have provided consent. This research was part of the dissertation submitted by the first author in partial fulfillment of the PhD degree.
Disclosure statement
No potential conflict of interest was reported by the authors.
References
- Collinge DB, Kragh KM, Mikkelsen JD, et al. Plant chitinases. Plant J. 1993;3:31–40.
- Graham LS, Sticklen MB. Plant chitinases. Can J Bot. 1994;72:1057–1083.
- Selitrennikoff CP. Antifungal proteins. Appl Environ Microbiol. 2001;67:2883–2894.
- Theis T, Stahl U. Antifungal proteins: targets, mechanisms, and prospective applications. Cell Mol Life Sci. 2004;61:437–455.
- Taira T, Toma N, Ishihara M. Purification, characterization, and antifungal activity of chitinases from pineapple (Ananas comosus) leaf. Biosci Biotechnol Biochem. 2005;69:189–196.
- Taira T, Ohdomari A, Nakama N, et al. Characterization and antifungal actvity of gazyumaru (Ficus microcarpa) latex chitinases: both the chitin-binding and antifungal activities of class I chitinase are reinforced with increasing ionic strength. Biosci Biotechnol Biochem. 2005;69:811–818.
- De Jong AJ, Cordewener J, Lo Schiavo F, et al. A carrot somatic embryo mutant is rescued by chitinase. Plant Cell. 1992;4:425–433.
- Goormachtig S, Lievens S, Van De Velde W, et al. Srchi13, a novel early nodulin from Sesbania rostrata, is related to acidic class III chitinases. Plant Cell. 1998;10:905–915.
- Yeh S, Moffatt BA, Griffith M, et al. Chitinase genes responsive to cold encode antifreeze proteins in winter cereals. Plant Physiol. 2000;124:1251–1264.
- Kasprzewska A. Plant chitianses: regulation and function. Cell Mol Biol Lett. 2003;8:809–824.
- Shinshi H, Neuhas JM, Ryals J Jr. Meins F. Structure of a tobacco endochitinase gene: evidence that different chitinase genes can arise by transposition of sequences encoding a cysteine-rich domain. Plant Mol Biol. 1990;14:357–368.
- Melchers LS, Apotheker-De Groot M, Van Der Knaap JA, et al. A new class of tobacco chitinases homologous to bacterial exo-chitinases displays antifungal activity. Plant J. 1994;5:469–480.
- Yamagami T, Mine Y, Ishiguro M. Complete amino acid sequence of chitinase-a from bulbs of gladiolus (Gladiolus gandavensis). Biosci Biotechnol Biochem. 1998;62:386–389.
- Taira T. Structures and antifungal activity of plant chitinases. J Appl Glycosci. 2010;57:167–176.
- Broekaert I, Lee HI, Kush A, et al. Woundinduced accumulation of mRNA containing a hevein sequence in laticifers of rubber tree (Hevea brasiliensis). Proc Natl Acad Sci U S A. 1990;87:7633–7637.
- Taira T, Mahoe Y, Kawamoto N, et al. Cloning and characterization of a small family 19 chitinase from moss (Bryum coronatum). Glycobiology. 2011;21:644–654.
- Yamagami T, Ishiguro M. Complete amino acid sequences of chitinase-1 and −2 from bulbs of genus Tulipa. Biosci Biotechnol Biochem. 1998;62:1253–1257.
- Kitaoku Y, Umemoto N, Ohnuma T, et al. A class III chitinase without disulfide bonds from the fern, Pteris ryukyuensis: crystal structure and ligand-binding studies. Planta. 2015;242:895–907.
- Taira T, Hayashi H, Tajiri Y, et al. A plant class V chitinase from a cycad (Cycas revoluta): biochemical characterization, cDNA isolation, and posttranslational modification. Glycobiology. 2009;19:1452–1461.
- Ohnuma T, Numata T, Osawa T, et al. A class V chitinase from Arabidopsis thaliana: gene responses, enzymatic properties, and crystallographic analysis. Planta. 2011;234:123–137.
- Onaga S, Toira T. A new type of plant chitinase containig LysM domains from a fern (Pteris ryukyuensis): roles of LysM domains in chitin binding and antifungal activity. Glycobiology. 2008;18:414–423.
- Inamine S, Onaga S, Ohnuma T, et al. Purification, cDNA cloning, and characterization of LysM-containing plant chitinase from horsetail (Equisetum arvense). Biosci Biotechnol Biochem. 2015;79:1296–1304.
- Kenrick P, Crane P. The origin and early evolution of plants on land. Nature. 1997;389:33–39.
- Banks JA, Nishiyama T, Hasebe M, et al The selaginella genome identifies genetic changes Associated with the evolution of vascular plants. Science. 2011;332:960–963.
- Smith PK, Krohn RI, Hermanson GT, et al. Measurement of protein using bicinchoninic acid. Anal Biochem. 1985;150:76–85.
- Laemmli UK. Cleavage of structural proteins during the assembly of the head of bacteriophage T4. Nature. 1970;227:680–685.
- Imoto T, Yagishita K. A simple activity mesurement of lysozyme. Agric Biol Chem. 1971;35:1154–1156.
- Cleveland D, Fischer S, Kirschner M, et al. Peptide mapping by limited proteolysis in sodium dodecyl sulfate and analysis by gel electrophoresis. J Biol Chem. 1977;252:1102–1106.
- Koga D, Yoshioka T, Arakane Y. HPLC analysis of anomeric formation and cleavage pattern by chitinolytic enzyme. Biosci Biotechnol Biochem. 1998;62:1643–1646.
- Bendtsen JD, Mielsen H, Von Heijin G, et al. Improved prediction of signal peptides: signalP 3.0. J Mol Biol. 2004;340:783–795.
- Marchler-Bauer A, Anderson JB, Cherukuri PF, et al. CDD: a conserved domain database for protein classification. Nucleic Acids Res. 2005;33:D192–D196.
- Larkin MA, Blackshields G, Brown NP, et al. Clustal W and Clustal X version 2.0. Bioinformatics. 2007;23:2947–2948.
- Passarinho PA. and de Vries SC. Arabidopsis chitinases: a genomic survey. Arabidopsis Book. 2002;1:e0023.
- Zhao K-J, Chye M-L. Methyl jasmonate expression of a novel Brassica juncea chitinase with two chitin-binding domains. Planta Mol Biol. 1999;40:1009–1018.
- Ohnuma T, Taira T, Fukamizo T. Antifungal activity of recombinant class V chitinases from Nicotiana tabacum and Arabidopsis thaliana. J Appl Glycosci. 2012;59:47–50.
- Ohnuma T, Numata T, Osawa T, et al. Crystal stracture and mode of action of a class V chitinase from Nicotiana tabacum. Plant Mol Biol. 2011;75:291–304.
- Umemoto N, Ohnuma T, Urpilainen H, et al. Biosci. Role of tryptophan residues in a class V chitinase from Nicotiana Tabacum. Biosci Biotechnol Biochem. 2012;76:778–784.
- Tian Y, Liu W, Cai J, et al. The nodulation factor hydrolase of Medicago truncatula: characterization of an enzyme specifically cleaving rhizobial nodulation signals. Plant Physiol. 2013;163:1179–1190.
- Long SR. Rhizobium symbiosis: nod factors in perspective. Plant Cell. 1996;8:1885–1898.