ABSTRACT
For strain improvement of Aspergillus oryzae, development of the transformation system is essential, wherein dominant selectable markers, including drug-resistant genes, are available. However, A. oryzae generally has a relatively high resistance to many antifungal drugs effective against yeasts and other filamentous fungi. In the course of the study, while investigating azole drug resistance in A. oryzae, we isolated a spontaneous mutant that exhibited high resistance to azole fungicides and found that pleiotropic drug resistance (PDR)-type ATP-binding cassette (ABC) transporter genes were upregulated in the mutant; their overexpression in the wild-type strain increased azole drug resistance. While deletion of the gene designated atrG resulted in increased azole susceptibility, double deletion of atrG and another gene (atrA) resulted in further azole hypersensitivity. Overall, these results indicate that the ABC transporters AtrA and AtrG are involved in azole drug resistance in A. oryzae.
Graphical Abstract
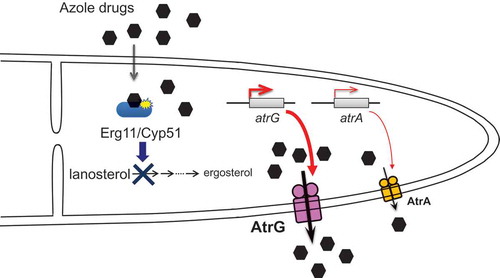
ABC transporters AtrG and AtrA are involved in the azole drug resistance possibly by their drug efflux activities in A. oryzae.
Aspergillus oryzae, a filamentous fungus, is industrially important in the production of some traditional Japanese fermented foods, including sake, soy sauce, and miso (soybean paste) [Citation1]. Furthermore, because it can excrete copious amounts of hydrolytic enzymes, i.e., amylolytic and proteolytic enzymes, it has been used for the industrial production of these hydrolytic enzymes [Citation2,Citation3]; this advantage can promote A. oryzae as a prominently suitable host for production of homologous and heterologous proteins [Citation4,Citation5]. Therefore, strain improvement of A. oryzae is necessary to develop the transformation system wherein multiple dominant selectable markers, including drug-resistant genes, are available. However, A. oryzae has a relatively high resistance to many antifungal drugs, e.g., hygromycin, blasticidin, and oligomycin, that are effective against yeasts and other filamentous fungi [Citation6,Citation7], although A. oryzae is susceptible to some drugs, including azoles and benomyl. Thus, we are interested in the mechanisms underlying such multiple drug resistance in A. oryzae.
While investigating drug resistance in A. oryzae, we identified a colony of the wild-type strain that showed good growth on azole fungicide-containing agar medium. Hence, we isolated this spontaneous mutant and aimed to clarify the reason for this mutant to show such high resistance to azole drugs. Because the target enzyme of azole fungicides is lanosterol 14α-demethylase, an enzyme involved in ergosterol biosynthesis of fungal cells [Citation8–Citation10], it has been most frequently reported that mutations in this enzyme or encoding gene led to azole drug resistance [Citation9,Citation11–Citation14]. An alternative mechanism for azole drug resistance is the upregulation of genes encoding drug efflux pumps, primarily belonging to ATP-binding cassette (ABC) transporters [Citation14–Citation18]. Therefore, we assumed that the spontaneous mutation resulting in azole drug resistance in A. oryzae could be attributed to a mutation in the gene encoding lanosterol 14α-demethylase or upregulation of gene(s) encoding drug efflux pumps. In the present study, we observed that the upregulation of the genes encoding pleiotropic drug resistance (PDR)-type ABC transporters, designated AtrA and AtrG, possibly resulted in an increase in azole drug resistance in the mutant. Indeed, overexpression of the transporters conferred hyper-resistance against azole drugs to the transformants compared with the wild-type strain. Furthermore, we discovered that deletion of these ABC transporter genes could lead to hyper-susceptibility to azole drugs, suggesting that AtrA and AtrG are involved in azole drug efflux and result in azole drug resistance in A. oryzae.
Materials and methods
Strains and media
In this study, A. oryzae RIB40 and RIB203 (stock cultures in National Research Institute of Brewing, Higashi-Hiroshima, Japan) were used as the parental wild-type strains, whereas A. oryzae niaD300 (niaD−) [Citation19] was used as the recipient strain for constructing overexpression strains of the PDR-type ABC transporter genes. For disruption of the ABC transporter genes, A. oryzae ∆ligD::loxP pyrG-deficient strain (∆ligD::loxP, niaD−, sC−, pyrG−) [Citation20] was used as the recipient strain. Further, Escherichia coli DH5α was used for the construction and propagation of plasmid DNAs. Czapek–Dox (CD) medium (containing 0.5% (NH4)2SO4, 0.05% KCl, 0.2% KH2PO4, 0.05% MgSO4, trace amounts of FeSO4, ZnSO4, CuSO4, MnSO4, Na2B4O7, and (NH4)6Mo7O24, and glucose or maltose as the carbon source) was used as a standard minimal medium for fungal cultivation, and YPD medium (containing 0.5% yeast extract, 0.5% polypeptone, and 1% glucose) was used as a complete liquid medium for fungal transformation.
Overexpression and deletion constructs
Overexpression constructs of the ABC transporter genes were generated using the plasmid pNGAG1 [Citation19] harboring the niaD gene as a selectable marker and the glaA gene promoter region for overexpression of the target gene. The ABC transporter genes, atrA, atrG, and atrH, were amplified by polymerase chain reaction (PCR) using the primers shown in Supplementary Table S1, e.g., in brief, the N-terminal 2-kb fragment of atrA comprising the translation initiation codon was PCR-amplified using the primers AoatrAsense + AoatrAanti (Table S1). Then, the resulting fragment was subcloned to the TA cloning vector pCR-TOPO® (Thermo Fisher Scientific, Tokyo, Japan) and digested with SalI and XbaI, whose restriction sites were included in AoatrAsense and AoatrAanti, respectively. Next, the SalI/XbaI-digested DNA fragment was inserted into pNGAG1, from which the uidA gene was excised in advance by digestion with SalI and XbaI, yielding pNGAatrA. Similarly, the plasmids pNGAatrG and pNGAatrH for overexpression of atrG and atrH were generated using the DNA fragments PCR-amplified with the primers AoatrG(TF)sense + AoatrG(TF)anti and AoatrHsense + AoatrHanti, respectively, and the resulting plasmids were digested with NdeI, whose restriction sites are located within the coding regions of the ABC transporter genes, followed by their introduction into the recipient strain A. oryzae niaD300.
To delete the ABC transporter gene atrA, a 1-kb fragment upstream of the start codon of atrA and a 1-kb fragment downstream of the stop codon were PCR-amplified using the primers datrAupFw + datrAupRvpyrG and datrAdwFwpyrG + datrAdwRv, respectively, and using A. oryzae RIB40 genomic DNA as the template. The resulting DNA fragments were used for fusion PCR amplification in combination with the PCR-amplified A. nidulans pyrG gene using the primers AnpyrGFw + AnpyrGRv and A. nidulans FGSC4 genomic DNA as the template. Similarly, the atrG deletion construct was generated by PCR amplification of 1-kb fragments upstream and downstream of atrG with the primers datrGupFw + datrGupRvpyrG and datrGdwFwpyrG + datrGdwRv, respectively, followed by their combination with A. nidulans pyrG fragment by fusion PCR. Moreover, for the atrH deletion, 1-kb fragments upstream and downstream of atrH were PCR-amplified with the primers datrHupFw + datrHupRvpyrG and datrHdwFwpyrG + datrHdwRv, respectively.
Regarding double deletions for the ABC transporter genes, A. nidulans sC, which was PCR-amplified using the primers AnsCFw + AnsCRv and the plasmid pUSC [Citation21] as the template, was used as a selectable marker for transformation of the atrA or atrG deletion mutant strains. The deletion construct of atrA was produced by PCR amplification of 1-kb fragments upstream and downstream of atrA with the primers datrAupFw + datrAupRvsC and datrAdwFwsC + datrAdwRv, respectively, followed by combination with A. nidulans sC fragment by fusion PCR. In addition, the fragments upstream and downstream of atrH were PCR-amplified with the primers datrHupFw + datrHupRvsC and datrHdwFwsC + datrHdwRv, respectively.
Transformation of A. oryzae
Based on a previous report, mycelial protoplasts were prepared with minor modifications and transformation of A. oryzae was performed [Citation22]. Overexpression strains for three ABC transporters were obtained by restriction enzyme-mediated integration (REMI) method [Citation23]. The plasmids were digested with NdeI, whose restriction site was within the coding region and directly introduced into the protoplasts, and with NdeI by polyethylene glycol (PEG)–CaCl2 method.
Northern blot analysis
The wild-type strain and spontaneous resistant mutant were grown in CD medium (2% glucose) for 72 h at 30°C. Then, they were harvested and divided into two parts; each was further grown in CD medium with or without 10 μg/mL miconazole for 8 h, followed by harvesting and disrupting the mycelium for RNA preparation. Northern blots were hybridized with probes for atrA, atrG, and atrH. Digoxigenin (DIG)-labeled DNA fragments generated using the DIG Probe Synthesis Kit (Roche, Indianapolis, IN) with the primer sets for corresponding genes (atrA; AoatrAsense + AoatrAanti, atrG; AoatrGsense + AoatrGanti, atrH; AoatrHsense + AoatrHanti) were used as probes.
Estimation of drug resistance and susceptibility of fungal strains
Approximately 104 conidiospores of the wild-type strain and overexpression strains for ABC transporters were inoculated on CD agar medium containing 2 μg/mL clotrimazole or 20 μg/mL miconazole and cultivated for 14 days at 30°C. To estimate drug susceptibility of the deletion mutant strains for ABC transporters, approximately 104–10 conidiospores were inoculated on CD agar media containing 0.15–0.2 µg/mL clotrimazole or 0.375–0.625 μg/mL miconazole supplemented with methionine and cultivated for 3 days at 30°C.
Results
Isolation and growth characteristics of an azole-resistant mutant of A. oryzae
A. oryzae generally shows high resistance to many antifungal drugs that are effective toward other eukaryotic microorganisms. However, it is sensitive to azole drugs, including clotrimazole, miconazole, and itraconazole, and its growth is inhibited by these drugs at low concentrations (approximately up to 1–5 μg/mL). On testing the growth phenotype of several A. oryzae wild-type strains on drug-containing agar media, we incidentally found a colony of RIB203 that showed vigorous growth on the medium during long-term cultivation. The drug-resistant mutant showed good growth on the agar medium containing 10 μg/mL miconazole, whereas the wild-type strain showed no growth on such a medium (). Because the drug-resistant mutant was obtained by incubating the parental strain on the medium containing azole drugs for an extended period, it would have a spontaneous mutation in the gene(s) involved in azole drug resistance.
Expression analysis of the ABC transporter genes in drug-resistant A. oryzae
Azole drug resistance in the yeast Saccharomyces cerevisiae is reportedly caused by increased ATP-dependent efflux of the drugs outside the cell, mediated by membrane-bound ABC transporter families (PDR5, PDR10, and SNQ2) [Citation24–Citation26], suggesting that ABC transporters are most likely crucially involved in azole drug resistance and that upregulation of their genes may result in the drug resistance in the A. oryzae spontaneous mutant, although alternative azole drug resistance is due to mutations in ergosterol biosynthetic genes, including erg11/cyp51 encoding sterol 14α-demethylase, the target enzyme of azole drugs. Because several genes encoding ABC transporter families are found in the DNA database of the A. oryzae genome sequencing project [Citation27], we first searched the A. oryzae EST database (http://www.nrib.go.jp/ken/EST/db/index.html) [Citation28] to find the candidate ABC transporter genes that were seemingly expressed in the wild-type strain RIB40, and we found four independent EST clones homologous to the genes encoding fungal ABC transporters. These EST clones encode the N-terminal truncated proteins, but the corresponding genes are present in the A. oryzae genome database and were designated atrA (AO090010000219), atrG (AO090011000378), atrH (AO090012000328), and mdrA (AO090009000651). The protein encoded by atrA had a high similarity to Aspergillus nidulans AtrA (AN8928) [Citation16] and that encoded by mdrA was highly homologous to Aspergillus fumigatus Mdr1 (Afu5g06070) [Citation29] and A. nidulans AtrD (AN2300) [Citation30] (). The atrG-encoding protein was highly similar to A. fumigatus Cdr1B/AbcC/AbcB (Afu1g14330) [Citation31–Citation33], whereas the atrH-encoding protein was homologous to A. fumigatus AbcA (Afu2g15130) [Citation33] (). According to the protein structures predicted, AtrA, AtrG, and AtrH belong to PDR-type ABC transporters, whereas MdrA belongs to multidrug resistant (MDR)-type ABC transporters (Supplementary Figure. S1). A recent study suggested that PDR-type ABC transporters and MDR-type ABC transporters are designated ABC-G and ABC-B subfamily proteins, respectively [Citation34].
Figure 2. Phylogenetic trees of the PDR-type (A) and MDR-type (B) ABC transporters from A. oryzae, A. fumigatus, and A. nidulans. The trees were generated using a multiple sequence alignment program ClustalW (http://align.genome.jp/) with default settings. Amino acid sequences of the proteins were obtained from the Aspergillus Genome Database (AspGD; http://www.aspgd.org/).
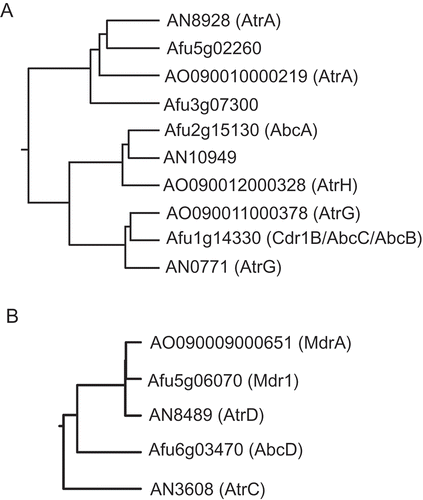
The Northern blot analysis using a respective ABC transporter gene as a probe revealed that the PDR-type ABC transporter genes, artA and atrG, are highly expressed in the drug-resistant mutant, particularly in the presence of the drug (). Another PDR-type ABC transporter gene, atrH, showed no detectable expression in the absence of the drug, but the expression of atrH seemed to be slightly upregulated in the wild-type and mutant strains in the presence of the drug. In contrast, the MDR-type ABC transporter gene, mdrA, is constitutively expressed in the wild-type and mutant strains, irrespective of the presence of the drug (data not shown). Overall, these findings indicate that increased transcription levels of the PDR-type ABC transporter genes, particularly atrA and atrG, are responsible for higher azole drug resistance in the mutant.
Overexpression of the ABC transporter genes in A. oryzae
To confirm the involvement of PDR-type ABC transporter genes in azole drug resistance in A. oryzae, atrA, atrG, and atrH were overexpressed in A. oryzae niaD300, used as the recipient strain under the control of the glucoamylase gene (glaA) promoter. Accordingly, the resident gene promoter was replaced by the maltose-inducible glaA promoter, PglaA, by homologous recombination (Supplementary Figure. S2); this promoter replacement was performed using REMI method as described in the Materials and Methods section, and the resultant transformants were subjected to Southern blot analyses to confirm that the promoter replacement had correctly occurred by recombination at the resident ABC transporter gene on the chromosome and that the transformants had a single copy of the expression construct (data not shown). As shown in , the transformants harboring each overexpression construct displayed better growth on the medium containing azole fungicides compared with the wild-type strain. Overexpression of atrG resulted in slightly poor growth compared with other ABC transporter genes, but the growth difference was not significant, as judged by better conidiation of the atrG overexpression strain, indicating that overexpression of the three ABC transporter genes endowed the fungal strains with high-level azole drug resistance.
Azole susceptibility of deletion mutants for ABC transporters
To evaluate the contribution of the three ABC transporters to azole drug resistance, we generated the deletion mutant strains for atrA, atrG, and atrH and determined their susceptibility to the azole drugs. All the deletion mutants were confirmed by Southern blot analyses (). As shown in ), although the deletion mutant for atrG showed relatively reduced growth on the medium without azole drugs compared with the wild-type and other ABC transporter deletion strains, it displayed significantly poorer growth on azole drug-containing agar media. In contrast, deletion of atrA and atrH had no apparent effect on fungal growth on the azole media. To further examine the contribution of atrA and atrH to azole drug resistance, double deletion mutants, ΔatrAΔatrG, ΔatrGΔatrH, and ΔatrAΔatrH, were generated and tested for their drug susceptibility. As shown in ), additional atrA deletion resulted in an increase in azole drug susceptibility of the atrG deletion mutant, whereas no apparent increase in the drug susceptibility was observed in the ΔatrGΔatrH mutant. Moreover, double deletion of atrA and atrH had no effect on drug susceptibility compared with the wild-type strain (Supplementary Figure. S3). These results suggest that, among the three ABC transporters, AtrG is most responsible for azole drug resistance and AtrA partly contributes to azole drug resistance by complementing the function of AtrG in the wild-type strain. Conversely, AtrH seems to hardly be involved in azole drug resistance in the wild-type strain. In addition to azole drugs, it would be interesting to examine whether the deletion mutants for ABC transporters show hyper-susceptibility against other fungicides, including hygromycin and blasticidin.
Figure 5. Construction of the deletion mutants for the three ABC transporter genes in A. oryzae. (A) Strategies for single gene deletion by homologous recombination and Southern blot analysis of the deletion mutants. Genomic DNAs of the wild-type and deletion mutant strains were digested with the restriction enzyme indicated. Digested DNAs were electrophoresed on a 0.8% agarose gel and transferred onto a nylon membrane. The predicted sizes of the signals resulted by digestion with restriction enzymes are shown in the panel. (B) Strategies for construction of double deletion mutants using the ΔatrG mutant as a host strain. Southern blot analysis was performed as described in (A).
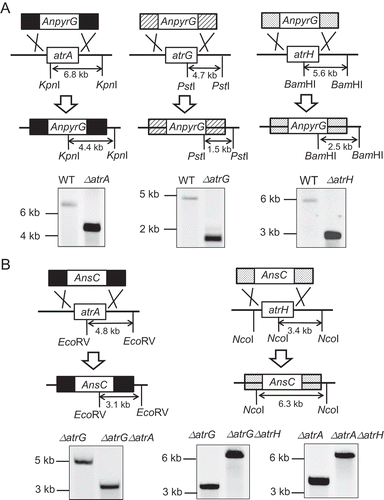
Figure 6. Susceptibility of the deletion mutant strains against the azole fungicides. (A) Growth of the single deletion mutants on the azole-containing medium. Approximately 104–10 conidiospores were inoculated on Czapek–Dox agar medium containing 0.15 µg/mL clotrimazole or 0.375 μg/mL miconazole supplemented with methionine and cultivated for 3 days at 30°C. (B) Growth of the double deletion mutants on the azole-containing medium. The strains were grown under the same culture conditions as described in (A).
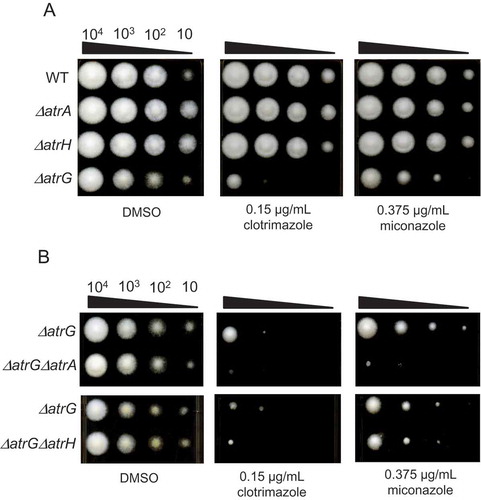
Discussion
We previously established the transformation systems for A. oryzae [Citation21,Citation22]; using these systems, several molecular analyses have been performed on A. oryzae. These transformation systems are commonly based on the complementation of nutrient requirements using strains that harbor corresponding gene mutations. However, owing to the relatively higher resistance in A. oryzae to most antifungal drugs, the drug-resistant genes cannot be made available as the dominant selectable markers for transformation of A. oryzae, with the only exception being the thiamine biosynthetic gene mutant [pyrithiamine resistance gene (ptrA)] that can endow A. oryzae strains with resistance against the thiamine analogue pyrithiamine [Citation7]. Thus, we are interested in determining the reason for such high resistance in A. oryzae against a wide range of antifungal drugs that are generally effective to yeasts and other filamentous fungi.
Notably, we could isolate the spontaneous mutant that showed high resistance to azole drugs. As described in the Results section, the expression of two PDR-type ABC transporter genes, atrA and atrG, was upregulated in the spontaneous resistant mutant without azole drugs and was further enhanced in the presence of azole drugs. Based on observation of the spontaneous mutant, overexpression of atrA and atrG resulted in a higher resistance to azole drugs in the wild-type strain. Furthermore, overexpression of another PDR-type ABC transporter gene, atrH, which was upregulated in the presence of azole drugs, caused hyper-resistance to azole drugs. These results suggest that atrA, atrG, and atrH encode drug efflux pumps, whose overexpression results in azole drug resistance. In the present study, although we investigated the expression of ABC transporter genes, it would be suitable to examine the involvement of ABC transporters in azole efflux at the protein level.
Deletion analysis of these transporter genes showed that atrG was mostly responsible for azole drug resistance in the A. oryzae wild-type strain because its deletion resulted in a hypersensitivity to the drugs. In contrast, deletions of atrA and atrH apparently showed no effect on drug resistance in the wild-type strain, but double deletion of atrA and atrG resulted in a slightly higher azole sensitivity compared with the single atrG deletion mutant, suggesting that AtrA partly contributes to the azole drug resistance observed in the wild-type strain. In contrast to the observation in the overexpression strains for atrA and atrH, deletion analysis showed that atrA and atrH did not seem to be involved in azole drug resistance in the wild-type strain of A. oryzae. This discrepancy might be explained by the transcription levels of these ABC transporter genes, according to the microarray and RNA-seq analyses performed by the National Research Institute of Brewing (https://nribf21.nrib.go.jp/CFGD/). While atrG was expressed highest among the three ABC transporter genes in the wild-type strain, atrA and atrH were expressed at significantly low levels. In reality, the preliminary experiment of quantitative reverse transcription PCR (qRT-PCR) analysis showed that the expression levels of atrA and atrH were approximately 10- and 100-fold lower than that of atrG, respectively (data not shown), suggesting that AtrA and AtrH potentially have an efflux activity against azole fungicides, but they appear to hardly contribute to azole drug resistance owing to significantly low levels of their gene expression in the wild-type strain. Nevertheless, because overexpression of all three ABC transporter genes conferred azole drug resistance on the wild-type strain, it would be useful to examine, by biochemical analysis, whether these ABC transporter genes indeed possess an ability to export the azole fungicides out of the cell. Furthermore, because a number of PDR-type ABC transporter genes are found in the A. oryzae genome, it is possible that there are some ABC transporters, other than AtrH, that potentially possess azole drug efflux activity.
A. oryzae has long been used for the production of traditional Japanese fermented foods; thus, it is regarded as a safe microorganism [Citation1–Citation3], and azole drug resistance in this fungus is unlikely to be relevant from a medical aspect. On the other hand, infections by the human pathogenic fungi A. fumigatus are recently increasing worldwide as the most frequent fungal disease [Citation35,Citation36]. For the treatment of A. fumigatus infections, azole fungicides that inhibit the ergosterol biosynthesis in the fungal cell membrane have been most commonly employed [Citation8,Citation9]. However, during the long-term usage of these drugs for therapy, azole-resistant isolates of A. fumigatus have been documented [Citation37,Citation38]. There are several genetic backgrounds that can confer azole drug resistance on A. fumigatus isolates. In addition to mutations in a gene (erg11/cyp51) involved in ergosterol biosynthesis that have been most frequently reported [Citation9,Citation11–Citation14], another possible mechanism of azole resistance in A. fumigatus is the upregulation of genes encoding ABC transporters. In this context, there are many reports that have described ABC transporters involved in azole drug resistance in A. fumigatus [Citation14–Citation18]. In the present study, the ABC transporter AtrG was shown to be most responsible for azole drug resistance observed in A. oryzae. Consistent with this finding, an ortholog of A. fumigatus, Cdr1B/AbcC/AbcB [Citation31–Citation33], was also reported to be involved in azole resistance because the insertional mutation occurring in the coding region of the cdr1B gene resulted in increased susceptibility to azole drugs [Citation39]. Furthermore, expression levels of cdr1B were upregulated in azole-resistant strains of A. fumigatus, and disruption of cdr1B increased azole susceptibility [Citation32,Citation33]. These observations indicate that AtrG and its orthologs commonly play a pivotal role in azole drug efflux in Aspergillus fungi.
In addition to AtrG, AtrA and AtrH seem to be involved in azole drug efflux, considering the overexpression experiments in the present study. While AtrA orthologs of A. fumigatus are Afu5g02260 and Afu3g07300, which have not yet been reported for their involvement in azole drug resistance, the transcription level of AN8928/atrA, an A. nidulans ortholog, was shown to be upregulated in the presence of an azole drug, imazalil [Citation16]. However, A. nidulans atrA failed to complement the yeast mutant defective in PDR5, recognized as a multidrug transporter gene, suggesting that AtrA does not possess multidrug efflux activity [Citation16], although AtrA possibly only has capability of azole drug efflux. On the other hand, overexpression of Afu2g15130/abcA, an atrH ortholog of A. fumigatus, was reported to confer azole drug tolerance, whereas deletion of Afu2g15130 did not apparently affect the drug resistance in the A. fumigatus wild-type strain [Citation33], consistent with the observations in our study. Overall, the three A. oryzae ABC transporters AtrA, AtrG, and AtrH, could actually possess azole drug efflux activity, and AtrG could play a major role in azole drug resistance in A. oryzae.
Recently, we identified a novel transcription factor, AtrR, which is involved in azole drug resistance in A. fumigatus, A. oryzae, and A. nidulans [Citation40]. Deletion of atrR led to hyper-susceptibility against azole drugs and a significant decrease in virulence in A. fumigatus. Furthermore, deletion of atrR resulted in significant downregulation of the cdr1B gene expression, indicating that AtrR is involved in regulating cdr1B expression in A. fumigatus [Citation40]. In addition, we preliminarily observed that atrR deletion caused a marked decrease in the transcription levels of atrA and atrG in A. oryzae. However, further detailed analyses are now proceeding to clarify the function of the transcription factor AtrR upon the expression of the ABC transporter genes, including atrA and atrG, the results of which will be reported elsewhere.
Author contributions
KG conceived and designed the experiments. DM, KS, AI, and AOT conducted the experiments with assistance from MT. MT, TS, and KG analyzed the data. KG wrote the manuscript.
AtrAG_BBB_Suppl_Figs_revised.pdf
Download PDF (287 KB)ABC_transporter_BBB_suppl._table.pdf
Download PDF (130.1 KB)Acknowledgments
The authors are grateful to Tomoko Shintani and Akio Nagano for their technical assistance.
The authors would like to thank Enago (www.enago.jp) for the English language review.
Disclosure statement
No potential conflict of interest was reported by the authors.
Supplementary material
Supplementary data can be accessed here.
References
- Machida M, Yamada O, Gomi K. Genomics of Aspergillus oryzae: learning from the history of koji mold and exploration of its future. DNA Res. 2008;15:173–183.
- Sakaguchi K, Gomi K, Tagagi M, et al. Fungal enzymes used in oriental food and beverage industries. In: Kinghorn JR, Turner G, editors. Applied Molecular Genetics of Filamentous Fungi. Glasgow: Blackie Academic & Professional; 1992. p. 54–99.
- Hara S, Kitamoto K, Gomi K. New developments in fermented beverages and foods with Aspergillus. In: Bennett JW, Klick M, editors. Biotechnology Series. Vol. 23. Aspergillus: Biology and Industrial Applications. Oxford: Butterworth-Heinemann; 1992. p. 133–153.
- Tanaka M, Gomi K. Stratagies for increasing the production level of heterologous proteins in Aspergillus oryzae. In: Anazawa H, Shimizu S, editors. Microbial Production: from Genome Design to Cell Enginieering. Tokyo: Springer; 2014. p. 149–164.
- Barbesgaard P, Heldt-Hansen HP, Diderichsen B. On the safety of Aspergillus oryzae: a review. Appl Microbiol Biotechnol. 1992;36:569–572.
- Gomi K, Kitamoto K, Kumagai C. Transformation of the industrial strain of Aspergillus oryzae with the homologus amdS gene as a dominant selectable marker. J Ferment Bioeng. 1992;74:389–391.
- Kubodera T, Yamashita N, Nishimura A. Pyrithiamine resistance gene (ptrA) of Aspergillus oryzae: cloning, characterization and application as a dominant selectable marker for transformation. Biosci Biotechnol Biochem. 2000;64:1416–1421.
- Sheehan DJ, Hitchcoch CA, Sibley CM. Current and emerging azole antifungal agents. Clin Microbiol Rev. 1999;12:40–79.
- Mellado E, Diaz-Guerra TM, Cuenca-Estrella M, et al Identification of two different 14-α sterol demethylase-related genes (cyp51A and cyp51B) in Aspergillus fumigatus and other Aspergillus species. J Clin Microbiol. 2001;39:2431–2438.
- Da Silva Ferreira ME, Colombo AL, Paulsen L, et al The ergosterol biosynthesis pathway, transporter genes, and azole resistance in Aspergillus fumigatus. Med Mycol. 2005;43:S133–139.
- Mann PA, Parmegiani RM, Wei SQ, et al Mutations in Aspergillus fumigatus resulting in reduced susceptibility to posaconazole appear to be restricted to a single amino acid in the cytochrome P450 14α-demethylase. Antimicrob Agents Chemother. 2003;47:577–581.
- Mellado E, Garcia-Effron G, Alcazar-Fuoli L, et al A new Aspergillus fumigatus resistance mechanism conferring in vitro cross-resistance to azole antifungals involves a combination of cyp51A alterations. Antimicrob Agents Chemother. 2007;51:1897–1904.
- Snelders E, Van Der Lee HA, Kuijpers J, et al Emergence of azole resistance in Aspergillus fumigatus and spread of a single resistance mechanism. PLoS Med. 2008;5:e219.
- Hagiwara D, Watanabe A, Kamei K, et al Epidemiological and genomic landscape of azole resistance mechanisms in Aspergillus fungi. Front Microbiol. 2016;7:1382.
- Prasad R, Dewergifosse P, Goffeau A, et al Molecular cloning and characterization of a novel gene of Candida albicans, CDR1, conferring multiple resistance to drugs and antifungals. Curr Genet. 1995;27:320–329.
- Del Sorbo G, Andrade AC, Van Nistelrooy JG, et al Multidrug resistance in Aspergillus nidulans involves novel ATP-binding cassette transporters. Mol Gen Genet. 1997;254:417–426.
- Del Sorbo G, Schoonbeek H, De Waard MA. Fungal transporters involved in efflux of natural toxic compounds and fungicides. Fungal Genet Biol. 2000;30:1–15.
- Slaven JW, Anderson MJ, Sanglard D, et al Increased expression of a novel Aspergillus fumigatus ABC transporter gene, atrF, in the presence of itraconazole in an itraconazole resistant clinical isolate. Fungal Genet Biol. 2002;36:199–206.
- Minetoki T, Nunokawa Y, Gomi K, et al Deletion analysis of promoter elements of the Aspergillus oryzae agdA gene encoding α-glucosidase. Curr Genet. 1996;30(5):432–438.
- Tanaka M, Yoshimura M, Ogawa M, et al The C2H2-type transcription factor, FlbC, is involved in the transcriptional regulation of Aspergillus oryzae glucoamylase and protease genes specifically expressed in solid-state culture. Appl Microbiol Biotechnol. 2016;100:5859–5868.
- Yamada O, Lee BR, Gomi K. Transformation system for Aspergillus oryzae with double auxotrophic mutations, niaD and sC. Biosci Biotechnol Biochem. 1997;61:1367–1369.
- Gomi K, Iimura Y, Hara S. Integrative transformation of Aspergillus oryzae with a plasmid containing the Aspergillus nidulans argB gene. Agric Biol Chem. 1987;51:2549–2555.
- Sánchez O, Navarro RE, Aguirre J. Increased transformation frequency and tagging of developmental genes in Aspergillus nidulans by restriction enzyme-mediated integration (REMI). Mol Gen Genet. 1998;258:89–94.
- Balzi E, Wang M, Leterme S, et al PDR5: a novel yeast multidrug resistance transporter controlled by the transcription regulator PDR1. J Biol Chem. 1994;269:2206–2214.
- Wolfger H, Mahé Y, Parle-McDermott A, et al The yeast ATP binding cassette (ABC) protein genes PDR10 and PDR15 are novel targets for the Pdr1 and Pdr3 transcriptional regulators. FEBS Lett. 1997;418:269–274.
- Decottignies A, Lambert L, Catty P, et al Identification and characterization of SNQ2, a new multidrug ATP binding cassette transporter of the yeast plasma membrane. J Biol Chem. 1995;270:18150–18157.
- Machida M, Asai K, Sano M, et al Genome sequencing and analysis of Aspergillus oryzae. Nature. 2005;438:1157–1161.
- Akao T, Sano M, Yamada O, et al Analysis of expressed sequence tags from the fungus Aspergillus oryzae cultured under different conditions. DNA Res. 2007;14:47–57.
- Schrettl M, Kim HS, Eisendle M, et al SreA-mediated iron regulation in Aspergillus fumigatus. Mol Microbiol. 2008;70:27–43.
- Andrade AC, Van Nistelrooy JG, Peery RB, et al The role of ABC transporters from Aspergillus nidulans in protection against cytotoxic agents and in antibiotic production. Mol Gen Genet. 2000;263:966–977.
- Da Silva Ferreira ME, Malavazi I, Savoldi M, et al (2006) Transcriptome analysis of Aspergillus fumigatus exposed to voriconazole. Curr Genet. 2006;50:32–44.
- Fraczek MG, Bromley M, Buied A, et al (2013) The cdr1B efflux transporter is associated with non-cyp51a-mediated itraconazole resistance in Aspergillus fumigatus. J Antimicrob Chemother. 2013;68:1486–1496.
- Paul S, Moye-Rowley WS. Functional analysis of an ATP-binding cassette transporter protein from Aspergillus fumigatus by heterologous expression in Saccharomyces cerevisiae. Fungal Genet Biol. 2013;57:85–91.
- Kovalchuk A, Driessen AJ. Phylogenetic analysis of fungal ABC transporters. BMC Genomics. 2010;11:177.
- Denning DW. Invasive Aspergillosis. Clin Infect Dis. 1998;26:781–803.
- Latgé JP, Calderone R. Host-microbe interactions: fungi invasive human fungal opportunistic infections. Curr Opin Microbiol. 2002;5:355–358.
- Moore CB, Sayers N, Mosquera J, et al Antifungal drug resistance in Aspergillus. J Infect. 2000;41:203–220.
- Dannaoui E, Borel E, Monier MF, et al Acquired itraconazole resistance in Aspergillus fumigatus. J Antimicrob Chemother. 2001;47:333–340.
- Bowyer P, Mosquera J, Anderson M, et al Identification of novel genes conferring altered azole susceptibility in Aspergillus fumigatus. FEMS Microbiol Lett. 2012;332:10–19.
- Hagiwara D, Miura D, Shimizu K, et al A novel Zn2-Cys6 transcription factor AtrR plays a key role in an azole resistance mechanism of Aspergillus fumigatus by coregulating cyp51A and cdr1B expressions. PLoS Pathog. 2017;13:e1006096.