ABSTRACT
The biotransformation of citral, an industrially important monoterpenoid, has been extensively studied using many microbial biocatalysts. However, the metabolic pathways involved in its biotransformation are still unclear, because citral is a mixture of the trans-isomer geranial and the cis-isomer neral. Here, we applied the heterologous expression of geoA, a gene encoding geraniol dehydrogenase that specifically converts geraniol to geranial and nerol to neral, to identify the metabolic pathways involved in the biotransformation of citral. Acinetobacter sp. Tol 5 was employed in order to demonstrate the utility of this methodology. Tol 5 transformed citral to (1R,3R,4R)-1-methyl-4-(1-methylethenyl)-1,3-cyclohexanediol and geranic acid. Biotransformation of citral precursors (geraniol and nerol) by Tol 5 transformant cells expressing geoA revealed that these compounds were transformed specifically from geranial. Our methodology is expected to facilitate a better understanding of the metabolic pathways involved in the biotransformation of substrates that are unstable and include geometric isomers.
Graphical Abstract
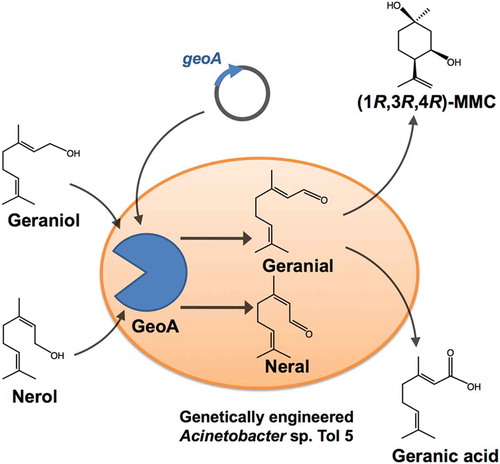
Heterologous expression of geoA was applied to identify the metabolic pathways involved in the biotransformation of citral by Acinetobacter sp. Tol 5.
Terpenoids are naturally occurring compounds that are widely used as industrially important chemicals such as pharmaceuticals, flavors, fragrances, and as large-volume feedstocks for chemical industries [Citation1,Citation2]. According to the number of isoprene units (C5H8), terpenoids are classified into numerous groups including monoterpenoids (two isoprene units), sesquiterpenoids (three isoprene units), and diterpenoids (four isoprene units). Monoterpenoids are the main constituents of essential oils and are used industrially as raw materials for flavor and fragrance compounds [Citation3,Citation4]. Citral, a volatile α,β-unsaturated aldehyde in the monoterpenoid series, is obtained from the essential oil of lemongrass (Cymbopogon citratus) and is composed of geometric isomers (trans-: geranial; cis-: neral). Due to its intense lemon aroma and flavor, citral has been used extensively in the food, cosmetic, and detergent industries since the early 1900s [Citation5]. In addition, derivatives of monoterpenoids including citral have the valuable potential to serve as biologically active compounds or as lead molecules for their development [Citation6].
Biotransformation using whole cells or isolated enzymes as catalysts is one of the ways to produce high-value oxygenated derivatives from monoterpenoids, which makes it possible to realize environmentally safe, energy-saving, and regio- and stereo-selective production under mild conditions [Citation7–Citation11]. To date, the biotransformation of citral by microorganisms including fungi, yeast, and bacteria has been investigated [Citation11–Citation17]. However, previous studies have not determined which biotransformation products are derived from which of the two citral isomers, that is, geranial and neral. This is due to their instability; although each pure isomer can be obtained from chemical synthesis, these are easily isomerized and autoxidized under ambient conditions [Citation18]. Hence, it is difficult to provide a biocatalyst with each isomer as the sole substrate to determine each metabolic pathway involved in the biotransformation of geranial or neral.
Geraniol dehydrogenase (GeoA) from Castellaniella defragrans is an enzyme that specifically converts geraniol to geranial and nerol to neral, respectively ()[Citation19]. We hypothesized that the introduction of geoA into a host strain might be useful for identifying the metabolic pathways involved in the biotransformation of citral, because it can provide geranial or neral as the sole substrate by intracellularly converting their respective precursors, geraniol and nerol. For proof of this concept, a desirable host strain requires four criteria: (1) citral biotransformation activity, (2) tolerance to the cytotoxicity of citral and its derivative products, (3) absence of the original capability for the biotransformation of citral precursors, and (4) established tools for genetic manipulation. Acinetobacter sp. Tol 5, a toluene-degrading Gram-negative bacterium isolated from a biofiltration process [Citation20], seemed to satisfy these criteria. Since strain Tol 5 can metabolize diverse aromatic hydrocarbons and organic solvents [Citation20,Citation21], it was expected to be capable of citral biotransformation and to show high tolerance to the cytotoxicity of citral and its derivatives. A sequence similarity search did not identify orthologs of geoA in the genome of Tol 5. Tools for the genetic manipulation of Tol 5 have already been established [Citation22,Citation23]. Here, we generated and utilized the transformant of Tol 5 into which geoA was introduced in order to demonstrate that the heterologous expression of geoA is useful for identifying the metabolic pathways involved in the biotransformation of citral.
Materials and methods
Substrates, microorganisms, and cell growth
Citral was purchased from Tokyo Chemical Industry Co., Ltd (Tokyo, Japan). Geraniol and nerol were purchased from Wako Pure Chemical Industries, Ltd. (Osaka, Japan). (R)-(–)- and S-(+)-α-methoxy-α-(trifluoromethyl)phenylacetyl chloride were purchased from Sigma-Aldrich (Tokyo, Japan).
Acinetobacter sp. Tol 5 and its transformant were grown on basal salt (BS) medium [Citation21] supplemented with sodium lactate (0.2%, wt/vol) or in Luria-Bertani (LB) medium at 28°C, with shaking. Ampicillin (500 μg/mL) and gentamicin (10 μg/mL) were added to the medium when required. Arabinose was added to a final concentration of 0.5% (wt/vol) for the induction of geoA.
The inhibitory effect of citral on the cell growth of Tol 5 was investigated by using the growth inhibition assay of Mi et al. [Citation24]. To avoid the volatilization of citral into the air, 15-mL conical centrifuge tubes with screw caps were used. Tol 5 cells were precultured in LB medium at 28°C with shaking for 8 h. Twenty microliters of the preculture was inoculated into 2 mL LB medium in a screw-capped test tube. Citral was added from a dimethyl sulfoxide (DMSO) stock solution at the beginning of the exponential growth phase, when the optical density at 660 nm (OD660) reached 0.2. Although a control with the highest applied DMSO concentration was also tested, DMSO had no effect on the cell growth of Tol 5. Samples were harvested during the exponential growth phase every hour and OD660 was measured. The maximum growth rate at a given citral concentration (μmax) was determined at the exponential growth phase immediately after citral addition. The maximum growth rate without citral (μmax0) at the exponential growth phase was also determined. Growth inhibition was evaluated using the ratio of μmax and μmax0 (μmax/μmax0).
Construction of a transformant of Acinetobacter sp. Tol 5 expressing geraniol dehydrogenase
The geoA gene optimized for codon usage of Tol 5 was artificially synthesized (Genewiz, Kawaguchi, Japan) and cloned into the EcoRI-XbaI site of pARP3, an Escherichia coli – Acinetobacter shuttle plasmid [Citation22], generating pGeoA. Genetic manipulation of Tol 5 with pGeoA was performed as described previously [Citation22]. The expression of geoA in the resultant transformant, Tol 5 (pGeoA), was confirmed by SDS-PAGE. Whole cell lysates of the samples were prepared as described previously [Citation21] and loaded on a 10.0% polyacrylamide gel. After electrophoresis, the gel was stained with Coomassie brilliant blue.
Analytical methods
1H- and 13C-NMR spectra were recorded at 500 MHz and 125 MHz, respectively, on a spectrometer (Advance III 500; Bruker Biospin Ltd.). Tetramethylsilane was used as the internal standard (1H-, δ 0.00; 13C-, δ 77.00) for NMR spectra measured in CDCl3. Multiplicities were determined by using the DEPT pulse sequence. Optical rotations were measured with a digital polarimeter (P-1010-GT; JASCO). The infrared (IR) spectra were recorded on a Fourier transform IR spectrometer (FT/IR-4100; JASCO). Thin-layer chromatography (TLC) was performed on precoated plates (Silica gel 60 F254, 0.25 mm; Merck). The mobile phase was hexane/ethyl acetate (EtOAc). Compounds were visualized by spraying the plates with 0.5% vanillin in 96% H2SO4 followed by brief heating. Gas chromatography-mass spectrometry (GC-MS) analysis was performed on a model 7820A gas chromatograph (Agilent Technologies, Wilmington, DE) equipped with a model MSD 5977E mass spectrometer (Agilent Technologies). A fused-silica capillary Rtx-200 column (30 m length, 0.32 mm i.d.; Restex, Bellefonte, PA) was used and helium was supplied through the column as the carrier gas at 10 mL/min. The oven temperature was programmed from 60 to 250°C at an increase rate of 4°C/min. The injection port temperature was maintained at 245°C. The GC column effluent was introduced directly into the ion source via a transfer line at 280°C. The ion source temperature was set at 230°C. The split ratio for the injection and the electron impact ionization voltage were set to 50:1 and 70 eV, respectively.
Time courses of biotransformation and autoxidation
One hundred and fifty microliters of precultured Tol 5 or Tol 5 (pGeoA) cells was inoculated into 15 mL BS medium supplemented with 8 μL toluene in a 50-mL conical centrifuge tube. When the OD660 of the culture reached 1.0, 80 mM citral, geraniol, or nerol dissolved in 20 mM DMSO was added to the culture for their biotransformation. During incubation at 28°C for 14 days with shaking at 115 rpm, 1 mL culture was harvested every other day, saturated with NaCl, and extracted with EtOAc, followed by evaporation of the solvent. The crude extracts were analyzed by TLC, GC, and GC-MS. To isolate a biotransformation product of citral by Tol 5, the crude extract obtained from a culture of the biotransformation for 14 days was subjected to silica gel open-column chromatography (silica gel, 40–66 µm; Wako) with gradient elution (hexane/EtOAc: 9:1 – 1:9). Relative abundances of the substrates and their metabolites were determined on the basis of GC peak area. Citral autoxidation was investigated at the same condition in the absence of microbial cells. The identities of the autoxidation products of citral were confirmed by comparison of their mass spectra to data reported in the literature [Citation25–Citation29], computational searches of a commercial mass spectral library (NIST 14, which includes 276,248 EI spectra for 242,466 compounds), and of authentic samples.
Structural analysis of 1-methyl-4-(1-methylethenyl)-1,3-cyclohexanediol as an isolated biotransformation product
Pale yellow oil: [α]D21.9 − 19.1° (CHCl3, c 0.70); EIMS, m/z (rel. intensity) 170 [M]+ (1), 152 (13), 134 (19), 119 (21), 108 (55), 87 (40), 82 (31), 68 (100), 58 (27), 43 (47); IR (KBr, νmax, cm−1) 3396; 1H NMR (CDCl3, 500 MHz) δ 1.28 (3H, s, H-7), 1.41 (1H, dd, J = 11.6, 2.7 Hz, H-6), 1.43 (1H, m, H-5), 1.50 (1H, dd, J = 13.9, 2.7, Hz, H-2), 1.68 (1H, m, H-5), 1.75 (3H, s, H-9), 1.85 (1H, m, H-6), 1.90 (1H, ddd, J = 12.7, 3.9, 3.9 Hz, H-4), 2.07 (1H, td, J = 13.9, 2.7 Hz, H-2), 3.82 (1H, td, J = 3.7, 2.7 Hz, H-3), 4.98 (2H, m, H-10); 13C NMR (CDCl3, 125 MHz) δ 146.0 (C, C-8), 113.1 (CH2, C-10), 71.4 (C, C-1), 67.3 (CH, C-3), 53.9 (C, C-4), 46.1 (CH2, C-2), 38.1 (CH2, C-6), 31.7 (CH3, C-7), 25.3 (CH2, C-5), 19.3 (CH3, C-9). The assigned compound was confirmed by comparison to the literature [Citation30].
Preparation of esters with (R)-(–) - and (S)-(+)-2-methoxy-2-phenyl-3,3,3-trifluoropropanoic acids
Esters of 1-methyl-4-(1-methylethenyl)-1,3-cyclohexanediol (MMC) with (R)-(–)- and (S)-(+)-2-methoxy-2-phenyl-3,3,3-trifluoropropanoic acids (MTPA esters) were prepared by the method of Ohtani et al. [Citation31], respectively. MMC (0.023 mmol) was dissolved in 2.0 ml dichloromethane and stirred with (R)-(–)- or (S)-(+)-α-methoxy-α-(trifluoromethyl)phenylacetyl chloride (0.041 mmol), trimethylamine (0.090 mmol), and 4-dimethylaminopyridine (0.004 mmol) at room temperature for 30 min under N2. The reaction mixture was concentrated in vacuo, and then (R)-(–)- or (S)-(+)-MTPA esters of MMC was purified by silica gel open-column chromatography.
Results
Tolerance of Acinetobacter sp. Tol 5 to citral
Since citral and some its biotransformation products show antimicrobial activity [Citation2], the tolerance of a host strain to citral is an important factor for its biotransformation. The tolerance of Tol 5 cells to citral was investigated using the growth inhibition assay. Maximum growth rates (μmax) at different concentrations of citral (10–100 mM) and µmax0 (in the absence of citral) were determined. The ratios of µmax and µmax0 were plotted against different concentrations of citral (). The cell growth of Tol 5 was significantly affected at 10 mM citral, distinctly impaired at 90 mM, and completely ceased at 100 mM. Since 6.6 μM – 29 mM citral was subjected to biotransformation in previous studies [Citation11–Citation17]. Tol 5 has sufficient tolerance to be used for citral biotransformation.
Figure 2. Growth inhibition of Acinetobacter sp. Tol 5 by citral.
µmax, maximum growth rate at a given citral concentration; and µmax0, maximum growth rate without citral.The experiments were conducted using three different conical centrifuge tubes with screw caps. Data are expressed as the mean ± standard division (n = 3).
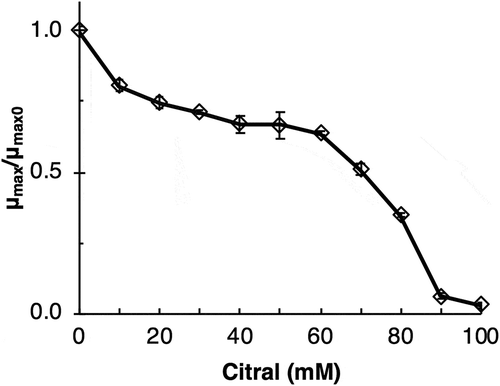
Capability of citral biotransformation in Acinetobacter sp. Tol 5
From the metabolic versatility of Tol 5 [Citation20,Citation21], we expected it would possess the capability for citral biotransformation. Biotransformation was investigated by incubating Tol 5 cells at 28°C with 80 mM citral for 14 days. To distinguish biotransformation products from autoxidation products, citral was also incubated in the same condition except for the absence of Tol 5 cells. The proportion of geranial and neral was 55:45 in the citral used in this study (). Even in the absence of Tol 5 cells, the relative abundance of geranial and neral decreased () and 7 products were detected (), indicating that autoxidation reactions progressed. In the presence of Tol 5 cells, the relative abundance of geranial and neral also decreased () and 8 products were detected (). On the basis of previous reports on the autoxidation and biotransformation of citral [Citation11–Citation17,Citation25–Citation29], 7 autoxidation products were identified by the mass spectral fragmentation pattern and retention time on the GC chromatogram; 3 aliphatic compounds were geranic acid, neric acid, and 3,7-dimethyl-6,7-epoxy-2-octenal, the other compounds possessed cyclic skeleton such as p-mentha-1,5-diene-8-ol, trans-p-menth-2-en-1,8-diol, p-cymen-8-ol, and 8-hydroperoxy-p-cymene. Although 7 of the 8 products in the presence of Tol 5 cells were identified as the same compounds produced by autoxidation in the absence of the cells, one product (cross marker in ) could not be identified. To identify this product, the large-scale biotransformation of citral in the presence of Tol 5 cells was performed, and then this product was isolated from the EtOAc extract by silica gel open-column chromatography. The molecular formula of this product was determined as C10H18O2 from its mass spectral fragmentation pattern. The IR spectrum contained a characteristic absorption band for an alcohol (3396 cm−1) compared with the citral. The 1H and 13C NMR spectra of the isolated product were assigned using the two-dimensional techniques: heteronuclear single-quantum coherence (HSQC), correlation spectroscopy (COSY), and heteronuclear multiple bond correlation (HMBC). The 1H and 13C NMR spectra of the isolated product indicated the existence of two new methylene groups and the disappearance of one methyl group and one quaternary carbon group. The characteristic COSY spectrum indicated the correlation cross-peaks of the three signals at δ3.82–2.07, 1.90, and 1.50 (Figure S1). The characteristic HMBC spectrum showed the key correlation of H-9 (1.75 ppm) to C-4 (53.9 ppm), C-8 (146.0 ppm), and C-10 (113.1 ppm); H-5 (1.43 ppm) to C-3 67.3 ppm), C-4 (53.9 ppm), and C-8 (146.0 ppm) (Figure S1). These data indicated that two hydroxyl groups should be located at C-1 and C-3 of the isolated product. Specific rotation shows the (−)-form (− 19.1°). Finally, the isolated product was determined as 1-methyl-4-(1-methylethenyl)-1,3-cyclohexanediol (MMC), which was reported as a major product from geraniol in chemical synthesis [Citation30], but has not been reported as a biotransformation product to date. The structural evidence was confirmed by comparing it with the spectroscopic data reported in the literature [Citation30]. The absolute configuration of MMC was determined by the modified Mosher’s method [Citation31]. This method only esterified the secondary hydroxyl group in position 3 of MMC. The differences of proton chemical shifts (Δδvalues, δS − δR) between (S)-MTPA ester of MMC and (R)-MTPA ester of MMC indicated that the configuration of C-1, C-3, and C-4 of MMC were R ((1R,3R,4R)-MMC, Figure S2). The products from the autoxidation and biotransformation of citral are summarized in . The present study is the first to demonstrate the biotransformation of citral into (1R,3R,4R)-MMC; however, it was not determined whether this compound was derived from geranial or neral.
Table 1. Production ratios of citral autoxidation and biotransformation by Acinetobacter sp. Tol 5.
Figure 3. Time courses of the autoxidation and biotransformation of citral by Acinetobacter sp. Tol 5.
(A) Consumption of citral during autoxidation. (B) Consumption of citral during biotransformation by Tol 5 cells. (C) Products from citral autoxidation. (D) Products from biotransformation by Tol 5 cells.
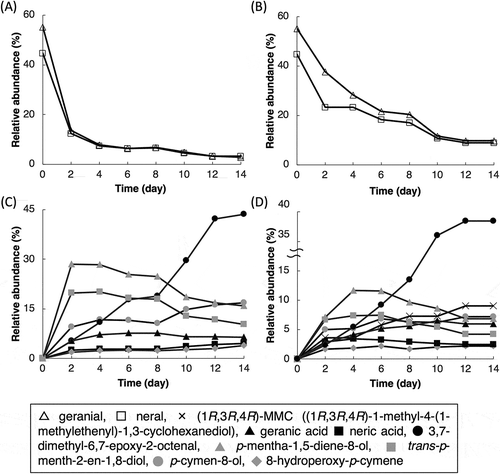
Expression of geoA in Acinetobacter sp. Tol 5
The biotransformation of citral precursors (geraniol and nerol) was investigated to confirm the absence of the capability for the biotransformation by the wild-type cells of Tol 5. Geraniol and nerol were incubated with Tol 5 cells at 28°C for 14 days, but no reaction occurred (Figure S3), indicating that Tol 5 cells are unable to biotransform citral precursors. Although geraniol and nerol were also incubated in the absence of Tol 5 cells, autoxidation did not occur (Figure S4), indicating that geraniol and nerol are more stable in the medium than citral.
Figure 4. Confirmation of GeoA expression in a transformant of Acinetobacter sp. Tol 5.
Whole cell lysate from Tol 5 transformant, Tol 5 (pGeoA), cells was analyzed by SDS-PAGE. An arrowhead indicates a protein induced remarkably by the addition of 0.5% arabinose. M, protein marker (AE-1440, EzStandard; ATTO); (+), Tol 5 (pGeoA) cells grown with 0.5% arabinose; (–), Tol 5 (pGeoA) cells grown without 0.5% arabinose.
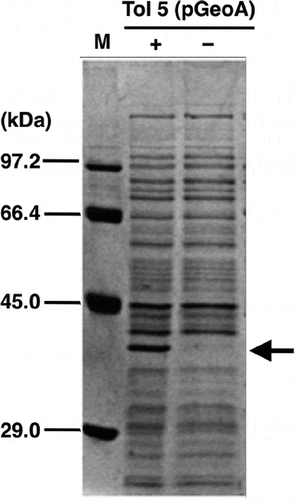
Figure 5. Time courses of the biotransformation of (A) geraniol and (B) nerol by a transformant of Acinetobacter sp. Tol 5 expressing geraniol dehydrogenase.
(1R,3R,4R)-1-methyl-4-(1-methylethenyl)-1,3-cyclohexanediol ((1R,3R,4R)-MMC) is indicated by the cross marker.
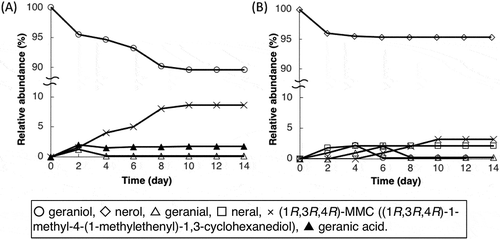
Figure 6. Reaction schemes of citral and its precursors in this study.
(A) Biotransformation of citral precursors (geraniol and nerol) by Acinetobacter sp. Tol 5 and its transformant expressing geraniol dehydrogenase. (B) Biotransformation products of citral by Acinetobacter sp. Tol 5 and autoxidation products of citral in the cultivation condition of Tol 5 cells.
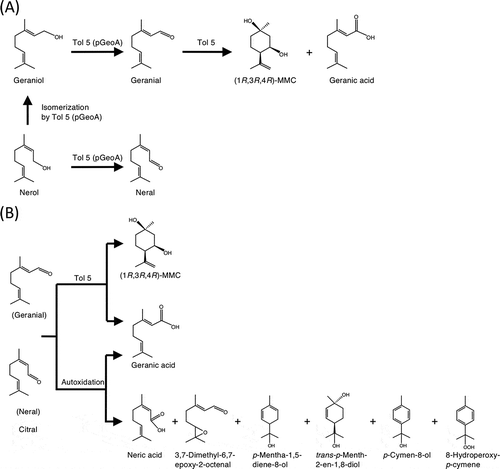
As Tol 5 is tolerant to citral and can transform it but not its precursors (geraniol and nerol), it was considered to be a suitable strain into which geoA could be introduced to identify the metabolic pathways involved in the biotransformation of citral. Therefore, Tol 5 was transformed with the pGeoA plasmid. The resultant transformant, Tol 5 (pGeoA), was confirmed to express geoA by SDS-PAGE (). Arabinose was added for the induction of geoA under the control of an arabinose-inducible promoter. A remarkable band was detected at approximately 38 kDa from Tol 5 (pGeoA) cells cultured in the presence of arabinose. Since the molecular weight of GeoA is 38,272 Da, the expression of geoA in the Tol 5 (pGeoA) cells could be confirmed.
Identification of the pathway for the synthesis of (1R,3R,4R)-1-methyl-4-(1-methylethenyl)-1,3-cyclohexanediol in Tol 5 (pGeoA) cells
To identify the pathway for the synthesis of MMC, the time courses of the biotransformation of geraniol and nerol by Tol 5 (pGeoA) cells were investigated. In the biotransformation of geraniol by Tol 5 (pGeoA) cells, 3 compounds ((1R,3R,4R)-MMC, geranic acid, and geranial) were detected during 14 days of incubation (). The relative abundance of the produced (1R,3R,4R)-MMC gradually increased and finally reached 8.6% at 10 days. The relative abundance of geranic acid and geranial increased to 2.0% and 1.2% in the first two days, but decreased to 1.5% and 0.1% on the fourth day, respectively. After that, the relative abundance of geranic acid finally increased to 1.7%, whereas that of geranial did not change. Since GeoA converts geraniol into geranial, but not into (1R,3R,4R)-MMC and geranic acid, this result indicates that (1R,3R,4R)-MMC and geranic acid were produced from the geranial generated by the oxidation of geraniol by GeoA (). In the biotransformation of nerol by Tol 5 (pGeoA) cells, 4 compounds were identified as neral, geraniol, geranial, and (1R,3R,4R)-MMC (). Unlike the biotransformation of geraniol, the level of geranic acid was below the detection limit of GC-MS. GeoA intracellularly oxidized nerol into neral, but the relative abundance of the produced neral (2.1%) did not change after the fourth day, implying no further reaction. The relative abundance of geraniol and geranial gradually increased to 2.2% and 2.0% until the fourth and sixth days, respectively, and thereafter it decreased to 0.1% and 0.2% in two further days of incubation, respectively. The sequential detection and disappearance of these two chemicals show that geraniol was produced by the isomerization of nerol by Tol 5 (pGeoA) and then oxidized into geranial. In contrast to geraniol and geranial, (1R,3R,4R)-MMC was not detected at the beginning of the incubation period, but it was detected gradually from the fourth day and its relative abundance finally increased to 3.2%. Since the relative abundance of (1R,3R,4R)-MMC increased with the decrease of geranial and geraniol, (1R,3R,4R)-MMC was considered to be produced from geranial oxidized from geraniol by GeoA.
Discussion
In the present study, the biotransformation of citral by Tol 5 cells and the autoxidation of citral in the absence of Tol 5 cells were investigated. The resultant products are listed in . Seven of the 8 biotransformation products detected were also produced by citral autoxidation in the absence of Tol 5 cells. Taking all of the analyses in the present study into account, the biotransformation products of citral by Tol 5 cells were geranic acid and (1R,3R,4R)-MMC, and the other compounds were derived from the autoxidation of citral (). Geranic acid was generated from both the biotransformation and autoxidation of citral. Although geranic acid was detected at a low level as the biotransformation product of geraniol by Tol 5 (pGeoA) cells (), it was below the detection limit as the biotransformation product of nerol by Tol 5 (pGeoA) cells (). Only a very small amount of geraniol was produced from nerol by Tol 5 (pGeoA) cells, resulting in the production of a small amount of geranial. Since the production ratio of (1R,3R,4R)-MMC and geranic acid was considered to be constant (5:1) in the biotransformation of geranial, the amount of geranic acid produced in the biotransformation of nerol was lower than the detection limit of GC-MS. The enzymatic activity involved in the production of (1R,3R,4R)-MMC in Tol 5 cells might be higher than that of geranic acid. Geranial dehydrogenase is known as an enzyme that coverts geranial into geranic acid [Citation19]. Sequence similarity search found 12 homologs of geranial dehydrogenase that show more than 30% amino acid sequence identity in the genome of Tol 5. Probably, some of them are involved in the biotransformation of geranial into geranic acid. On the other hand, no enzyme that converts geranial into (1R,3R,4R)-MMC has been known, because the present study is the first to detect it as the biotransformation product. Further study is needed to identify and characterize these enzymes in Tol 5 cells.
The autoxidation [Citation25–Citation29] and biotransformation of citral, one of the most valuable and widespread monoterpenoids, by bacteria, yeast, and fungi have been studied extensively [Citation11–Citation17]. The autoxidation of citral in the cultivation condition of Tol 5 cells started immediately, and cyclization and chain oxidation reactions occurred (). This autoxidation pathway of citral is similar to that observed in acidic conditions [Citation25–Citation29], but the number of products and the formation rates were different. Probably, such differences were attributed to the temperature and pH of the incubation conditions. In the biotransformation of citral by microorganisms, different products have been reported. For example, sporulated surface cultures of Aspergillus niger strains and Penicillium species mainly produced linalool [Citation12,Citation13], Penicillium digitatum transformed citral into 6-methylhept-5-en-2-one [Citation14]. The major products of A. niger PTCC5011 were citronellol and hydroxy citronellal [Citation15]. Zymomonas mobilis, Citrobacter freundii, Candida rugosa, and Candida parapsilosis produced citronellal or citronellol [Citation16,Citation17]. The present study is the first to demonstrate that (1R,3R,4R)-MMC was transformed from citral. From the results of the biotransformation of citral precursors (geraniol and nerol) by Tol 5 (pGeoA) cells, we finally concluded that (1R,3R,4R)-MMC was specifically transformed from geranial in Tol 5 cells.
Although our results indicated that autoxidation of citral progresses readily, even in the cultivation condition used in the present study, previous studies of citral biotransformation have not paid close attention to it. In the biotransformation of citral precursors by Tol 5 (pGeoA) cells, no autoxidation products of citral were detected (), indicating that the intracellularly produced geranial and neral were not affected by autoxidation. Since it is generally considered that the intracellular environments of microorganisms are reductive, it might be difficult for citral autoxidation to progress inside cells. Therefore, the biotransformation of more stable citral precursors by Tol 5 (pGeoA) cells could clearly distinguish the biotransformation products of citral from the autoxidation products of citral. In the field of microbiology, heterologous expression of an enzyme has been used extensively for the mass production of value-added chemicals. In contrast, we used the heterologous expression of geoA to distinguish biotransformation products from autoxidation products and to identify the metabolic pathways involved in the biotransformation of citral in Tol 5 cells. Since this methodology can be applied for genes encoding other enzymes, it is expected to facilitate a better understanding of the metabolic pathways involved in the biotransformation of substrates that are unstable and include geometric isomers.
Author contribution
AU designed and performed the experiments, analyzed the data, and wrote the manuscript. MI and KH discussed the results and completed the manuscript. All authors have read and approved the final manuscript.
2nd_Revised_Supplementaly_File.pdf
Download PDF (146.8 KB)Acknowledgments
This work was supported by the Advanced Low Carbon Technology Research and Development Program (ALCA) of the Japan Science and Technology Agency (JST) and by the Program for Leading Graduate Schools “Integrative Graduate Education and Research in Green Natural Sciences,” Ministry of Education, Culture, Sports, Science and Technology (MEXT), Japan. The authors have declared that no competing interests exist and they have no conflicts of interest with the contents of this article.
Disclosure statement
No potential conflict of interest was reported by the authors.
Supplementary Material:
Supplemental data for this article can be accessed here.
Additional information
Funding
References
- Joshi S, Chanotiya CS, Agarwal G, et al. Terpenoid compositions, and antioxidant and antimicrobial properties of the rhizome essential oils of different Hedychium species. Chem Biodivers. 2008;5:299–309.
- Vespermann KA, Paulino BN, Barcelos MC, et al. Biotransformation of alpha- and beta-pinene into flavor compounds. Appl Microbiol Biotechnol. 2017;101:1805–1817.
- Chisowa EH, Hall DR, Farman DI. Volatile constituents of the essential oil of Cymbopogon citratus Stapf grown in Zambia. Flavour Frag J. 1998;13:29–30.
- Van Der Werf MJ, De Bont JA, Leak DJ. Opportunities in microbial biotransformation of monoterpenes. Adv Biochem Eng Biotechnol. 1997;55:147–177.
- Surburg H, Panten J. Common fragrance and flavor materials: preparation, properties and uses, 5th completely revised and enlarged edition. Weinheim: Wiley – VCH; 2006.
- Salakhutdinov NF, Volcho KP, Yarovaya OI. Monoterpenes as a renewable source of biologically active compounds. Pure Appl Chem. 2017;89:1105–1117.
- Lye GJ, Dalby PA, Woodley JM. Better biocatalytic processes faster: new tools for the implementation of biocatalysis in organic synthesis. Org Process Res Dev. 2002;6:434–440.
- Doig SD, Simpson H, Alphand V, et al. Characterization of a recombinant Escherichia coli TOP10 [pQR239] whole-cell biocatalyst for stereoselective Baeyer–villiger oxidations. Enzyme Microb Technol. 2003;32:347–355.
- Xu F. Applications of oxidoreductases: recent progress. Ind Biotechnol. 2005;1:38–50.
- Pinheiro L, Marsaioli AJ. Microbial monooxygenases applied to fragrance compounds. J Mol Catal B Enzym. 2007;44:78–86.
- Demyttenaere J. and De Kimpe N, Biotransformation of terpenes by fungi - study of the pathways involved. J Mol Catal B Enzym. 2001;11:265–270.
- Demyttenaere JCR. and De Pooter HL, Biotransformation of citral and nerol by spores of Penicillium digitatum. Flavour Frag J. 1998;13:173–176.
- Demyttenaere JC, Del Carmen Herrera M. and De Kimpe N, Biotransformation of geraniol, nerol and citral by sporulated surface cultures of Aspergillus niger and Penicillium sp. Phytochemistry. 2000;55:363–373.
- Esmaeili A, Tavassoli A. Microbial transformation of citral by Penicillium sp. Acta Biochim Pol. 2010;57:265–268.
- Esmaeili A, Rohany S, Safaiyan S, et al. Microbial transformation of citral by Aspergillus niger-PTCC 5011 and study of the pathways involved. Czech J Food Sci. 2011;29:610–615.
- Müller A, Hauer B, Rosche B. Enzymatic reduction of the α, β-unsaturated carbon bond in citral. J Mol Catal B Enzym. 2006;38:126–130.
- Hall M, Hauer B, Stuermer R, et al. Asymmetric whole-cell bioreduction of an α,β-unsaturated aldehyde (citral): competing prim-alcohol dehydrogenase and C–C lyase activities. Tetrahedron: Asymmetry. 2006;17:3058–3062.
- Cannon RJ, Curto NL, Esposito CM, et al. The discovery of citral-like thiophenes in fried chicken. J Agric Food Chem. 2017;65:5690–5699.
- Lüddeke F, Wulfing A, Timke M, et al. Geraniol and geranial dehydrogenases induced in anaerobic monoterpene degradation by Castellaniella defragrans. Appl Environ Microbiol. 2012;78:2128–2136.
- Hori K, Yamashita S, Ishii S, et al. Isolation, characterization and application to off-gas treatment of toluene-degrading bacteria. J Chem Eng Japan. 2001;34:1120–1126.
- Hori K, Ishikawa M, Yamada M, et al. Production of peritrichate bacterionanofibers and their proteinaceous components by Acinetobacter sp. Tol 5 cells affected by growth substrates. J Biosci Bioeng. 2011;111:31–36.
- Ishikawa M, Nakatani H, Hori K. AtaA, a new member of the trimeric autotransporter adhesins from Acinetobacter sp. Tol 5 mediating high adhesiveness to various abiotic surfaces. PLoS One. 2012;7:e48830.
- Ishikawa M, Hori K. A new simple method for introducing an unmarked mutation into a large gene of non-competent Gram-negative bacteria by FLP/FRT recombination. BMC Microbiol. 2013;13:86–95.
- Mi J, Becher D, Lubuta P, et al. De novo production of the monoterpenoid geranic acid by metabolically engineered Pseudomonas putida. Microb Cell Fact. 2014;13:170–180.
- Kimura K, Nishimura H, Iwata I, et al. Deterioration mechanism of lemon flavor. 2. Formation mechanism of off-odor substances arising from citral. J Agric Food Chem. 1983;31:801–804.
- Schieberle P, Grosch W. Identification of potent flavor compounds formed in an aqueous lemon oil citric-acid emulsion. J Agric Food Chem. 1988;36:797–800.
- Ueno T, Masuda H, Ho CT. Formation mechanism of p-methylacetophenone from citral via a tert-alkoxy radical intermediate. J Agric Food Chem. 2004;52:5677–5684.
- Schieberle P. Garching, and Grosch W, Quantitative analysis of important volatile flavour compounds in fresh and stored lemon oil/citric acid emulsions. Lebensm Wiss Technol. 1988;21:158−162.
- Hagvall L, Baron JM, Borje A, et al. Cytochrome P450-mediated activation of the fragrance compound geraniol forms potent contact allergens. Toxicol Appl Pharmacol. 2008;233:308–313.
- Fkyerat A, Tabacchi R. Enantioselective preparation of 1-hydroxy neoisopulegol and 1-hydroxy neoisomenthol. Tetrahedron: Asymmetry. 1997;8:2231–2236.
- Ohtani I, Kusumi T, Kashman Y, et al. A new aspect of the high-field NMR application of Mosher’s method. The absolute configuration of marine triterpene sipholenol A. J Org Chem. 1991;56:1296–1298.