ABSTRACT
Benzyl isothiocyanate (BITC) is an organosulfur compound derived from cruciferous vegetables and papaya seeds. In this study, we investigated the effect of BITC on the lipid accumulation in 3T3-L1 preadipocytes during adipocyte differentiation. The treatment of BITC during the differentiation-inducing stage significantly ameliorated the lipid accumulation, whereas it had no inhibitory effect during the differentiation-maintaining stage. BITC also significantly suppressed the mRNA expression of the adipocyte-specific markers, such as CCAAT/enhancer-binding protein α (C/EBPα), C/EBPβ, C/EBPδ and peroxisome proliferator-activated receptor γ. BITC significantly inhibited the phosphorylation of extracellular signal-regulated kinase phosphorylation, whereas it enhanced that of AMP-activated protein kinase. Furthermore, BITC significantly suppressed the intracellular 2-deoxyglucose uptake as well as glucose transporter 4 expression. These results suggest that inhibition of the adipocyte differentiation and glucose uptake may mainly contribute to the inhibitory effect of BITC on the lipid accumulation in 3T3-L1 preadipocytes.
Abbreviations: PPARγ: peroxisome proliferator-activated receptor γ; CEBP: CCAAT/enhancer-binding protein; GLUT4: glucose transporter 4; AMPK: AMP-activated protein kinase; ERK1/2: extracellular signal-regulated kinase 1/2; MAPK: a mitogen-activated protein kinase; ITCs: isothiocyanates; BITC: benzyl isothiocyanate; FBS: fetal bovine serum; CS: calf serum; AITC: allyl ITC; IBMX: 3-isobutyl-1-methylxanthine; LDH: lactate dehydrogenase; KRH: Krebs-Ringer-Hepes-bicarbonate; 2-DG: 2-deoxy-d-glucose
Graphical Abstract

The proposed mechanism for inhibition of the lipid accumulation in preadipocytes by BITC.
Adipocytes play an important role in not only maintaining energy homeostasis, but also adipogenesis as a consequence of caloric overabundance [Citation1]. Adipogenesis is a process of the intracellular accumulation of triacyl glycerol by the differentiation, enlargement with lipogenesis and increase in the number of adipocytes [Citation2]. Since adipogenesis mainly contributes to the onset and progression of obesity, the inhibition of adipocyte differentiation, and enhancement of lipolysis are regarded as promising strategies in preventing the development of obesity [Citation3–Citation5].
The differentiation of preadipocytes into adipocytes is regulated by an elaborate transcriptional network such that the coordinated expression of hundreds of proteins is responsible for the maturing adipocytes [Citation6]. Among the transcriptional factors related to the adipocyte differentiation, peroxisome proliferator-activated receptor γ (PPARγ) and CCAAT/enhancer-binding protein α (C/EBPα) are master regulators for the formation of mature adipocytes [Citation7]. Glucose transporter 4 (GLUT4), whose expression is also regulated by C/EBPs, plays an important role in the glucose uptake by adipocytes [Citation8]. AMP-activated protein kinase (AMPK) inactivates acetyl CoA carboxylase and glycerol-3-phosphate acyl transferase-1, both of which play a key role in the lipogenesis of adipocytes [Citation9]. The activation of AMPK also contributes to the inhibition of adipocyte differentiation through down-regulation of C/EBPs and PPARγ [Citation10]. The extracellular signal-regulated kinase 1/2 (ERK1/2), a mitogen-activated protein kinase (MAPK), is another member of the protein kinases that regulate the adipocyte differentiation [Citation11].
Isothiocyanates (ITCs), well-studied electrophilic compounds, are naturally occurring in abundance in cruciferous vegetables including broccoli, watercress, Brussels sprouts, cabbage, Japanese radish and cauliflower [Citation12]. ITCs are regarded to play significant roles in affording the cancer chemopreventive potentials of these vegetables, because they are capable of up-regulating the xenobiotic-detoxifying enzymes, inducing apoptosis, and inhibiting cell cycle progression [Citation12,Citation13]. Benzyl isothiocyanate (BITC), an aromatic ITC derived from papaya [Citation14], has been demonstrated to induce phase 2 drug-metabolizing enzymes [Citation15] and alcohol-metabolizing enzymes [Citation16]. BITC also inhibits cell proliferation in various cell lines, such as T lymphocytes [Citation17], renal proximal tubular cells [Citation18], and colorectal cancer cells [Citation19]. BITC is also able to inhibit inflammatory responses in macrophages [Citation20] and the cytokine expression [Citation21,Citation22]. In addition, BITC has recently been reported to restrain the high-fat diet-induced body weight gain and liver fat accumulation [Citation23]. However, it remains unclear whether or how BITC modulates lipid accumulation in the adipocytes.
In the present study, we assessed BITC as a potential inhibitor of lipid accumulation in preadipocytes using the 3T3-L1 mouse fibroblast cell line, a well-characterized cell culture model for the analysis of adipocyte-specific differentiation [Citation24]. We also investigated the stage-specific effect of BITC on the lipid accumulation and the related signaling molecules, such as transcriptional factors, protein kinases and glucose transporter. The present results suggest that the inhibition of adipocyte differentiation and glucose uptake might mainly contribute to the inhibitory effect of BITC on the lipid accumulation in 3T3-L1 preadipocytes.
Materials and methods
Materials
BITC were purchased from LKT Laboratories, Inc. (St. Paul, MN, USA). Antibodies against phosphorylated-ERK, ERK, phosphorylated-AMPK, AMPK, and GAPDH were purchased from Cell Signaling Technology, Inc. (Beverly, MA, USA). Horseradish peroxidase-linked anti-rabbit IgG was purchased from Santa Cruz Biotechnology (Santa Cruz, CA, USA). Fetal bovine serum (FBS) and calf serum (CS) were purchased from Nichirei Corporation (Tokyo, Japan). Bio-Rad Protein Assay was purchased from Bio-Rad Laboratories (Hercules, CA, USA). Oil Red O solution was purchased from Cayman Chemical (Ann Arbor, MI, USA). Allyl ITC (AITC) and Compound C were purchased from Sigma Aldrich (St. Louis, MO, USA). All other chemicals including Chemi-Lumi One Super, dexamethasone, and 3-isobutyl-1-methylxanthine (IBMX) were purchased from Nacalai Tesque Inc. (Kyoto, Japan).
Cell culture and treatments
Mouse fibroblast cell line 3T3-L1 cells (ATCC® CL‐173™) were cultured in DMEM (Dulbecco’s modified Eagle’s medium, high glucose) supplemented with 10% calf serum and 1% penicillin/streptomycin at 37°C in a humidified chamber of 95% air and 5% CO2. After the cells were grown to the contact inhibition stage, differentiation was induced using the differentiation-inducing medium (DMEM supplemented 10% FBS, 0.5 mM IBMX, 1 μM dexamethasone, and 10 μg/mL insulin) for 3 days in the same medium. The cells were then cultured in the differentiation-maintaining medium (DMEM supplemented 10% FBS and 10 μg/mL insulin) for 3 days. Thereafter, the cells were cultured in DMEM supplemented 10% FBS for another 2 days. To investigate whether BITC is capable of inhibiting the intercellular lipid accumulation, the cells were treated with BITC or DMSO alone as a vehicle control at the time of the medium change into the differentiation-inducing medium, differentiation-maintaining medium or both media. To investigate the molecular mechanism by which BITC inhibits the adipocyte differentiation, the cells were treated with BITC or DMSO alone at the time of the medium change into the differentiation-inducing medium.
Ldh-release assay
Lactate dehydrogenase (LDH)-release assay was carried out for the quantitative determination of cytotoxicity. 3T3-L1 cells were seeded in 96-well plates at a density of 2 × 104 cells/well in culture medium. After incubation, the cells were treated with BITC for 3 days. LDH activity was measured by using an LDH-Cytotoxicity Test Wako, in accordance with the manufacturer’s instructions. The absorbance was measured at 560 nm. Total LDH release (100%) was obtained by the treatment of 0.1% Tween 20.
Oil red o staining
The matured differentiated 3T3-L1 cells in 12-well plates were washed twice with ice-cold PBS and fixed with 10% formalin for 10 min at room temperature. After removing formalin, the fixed cells were treated with 60% isopropanol for 1 min. The cells were stained with a 60% Oil Red O solution for 15 min at room temperature. After removing the Oil Red O solution, the cells were washed with 60% isopropanol and PBS. The oil droplets present in the stained cells were then dissolved in isopropanol, and absorbance of the dissolved solution was measured at 490 nm using a microplate spectrophotometer.
RT-PCR analysis
The post-confluent 3T3-L1 cells were treated with BITC in the differentiation-inducing medium for 3 days. The total RNA was then extracted using Trizol reagent according to the manufacture’s manual. The total RNA was reverse transcribed to cDNA using ReverTra Ace. PCR amplification was then performed with Taq polymerase. Primers used in PCR amplification are as follows: PPARγ, 5ʹ-TATggAgTTCATgCTTgTgA-3ʹ and 5ʹ-CgggAAggACTTTATgTATg-3ʹ (316bp); C/EBPα, 5ʹ-gAAggAACTTgAAgCACAAT-3ʹ and 5ʹ – gACACAgAgACCAgATACAA-3ʹ (111bp); C/EBPβ, 5ʹ-CAAgCTgAgCgACgAgTACA-3ʹ and 5ʹ-CAgCTgCTCCACCTTCTTCT-3ʹ (137bp); C/EBPδ, 5ʹ-gATCTgCACggCCTgTTgTA-3ʹ and 5ʹ-CTCCACTgCCCACCTgTCA-3ʹ (148bp); GLUT4, 5ʹ-TCCTTCTATTTgCCgTCCTC-3ʹ and 5ʹ-TgTTTTgCCCCTCAgTCATT-3ʹ (bp); and β-Actin 5ʹ-CTCACCCACACTgTgCCCATCTA-3ʹ and 5ʹ-gCAATgCCAgggTACATggTggT-3ʹ (454bp). The cycles and annealing temperatures used in PCR amplification are as follows: PPARγ, 22 cycles, 60°C; C/EBPα, 25 cycles, 59°C; C/EBPβ, 30 cycles, 64°C; C/EBPδ, 25 cycles, 67°C; GLUT4, 30 cycles, 64°C; and β-Actin 16 cycles, 65°C. The PCR products were separated on an agarose gel (2–3%), stained with ethidium bromide, and visualized under UV light. The relative densities of the bands were measured using an Image J Software Program (National Institutes of Health, Bethesda, MD, USA).
Western blot analysis
The post-confluent 3T3-L1 cells were treated with BITC in the differentiation-inducing medium for 2 or 3 days. The whole cell lysates were then prepared in lysis buffer (20 mM Tri-HCl pH 7.5, 150 mM NaCl, 1 mM EDTA, 1 mM EGTA, 2.5 mM NaH2PO4, 10 mM NaF, 2 mM Na3VO4, 1 mM phenylmethylsulfonyl fluoride, 1% sodium dodecyl sulfate, 1% sodium deoxycholate and 1% Triton X-100) containing protease inhibitor cocktail and left on ice for 20 min. After sonication, the lysates were centrifuged, and the supernatant was used as the whole cell lysates. The protein concentration in the supernatant was determined by the Bio-Rad protein assay. Equal quantities of protein were subjected to SDS-PAGE and transferred to Immobilon-P membranes. The membranes were blocked, then incubated with the primary antibody overnight at 4°C followed by an appropriate secondary antibody. The secondary antibody binding was visualized using a Chemi-Lumi One Super (Nacalai Tesque). Densitometric analysis of the bands was carried out using the Image J Software Program.
Glucose uptake assay
The glucose uptake assay was performed using an enzyme-dependent fluorometric assay as previously reported [Citation25]. After the treatment with BITC in the differentiation-inducing medium for 3 days, the cells were washed twice with Krebs-Ringer-Hepes-bicarbonate (KRH) buffer containing 0.1% BSA. The cells were incubated with KRH buffer containing 0.1% BSA and 2 mM 2-deoxy-d-glucose (2-DG) for 20 min in a 5% CO2 incubator. The cells were then washed twice with KRH buffer containing 0.1% BSA, added 0.1 M NaOH to each well and mixed using a plate mixer. The plate was heated to 75°C for 1 h using a laboratory heating oven. After heating, the lysate in the wells were neutralized by adding 0.1 M HCl. followed by the addition of 50 μL of 200 mM TEA buffer (pH 8.1). The cell lysate (10 μL) was transferred to a 96‐well plate for fluorescence measurement and incubated for 45 min with 100 μL of assay cocktail, containing 50 mM KCl, 0.1 mM NADP+, 20 U/mL of glucose-6-phosphate dehydrogenase, 0.2 units/mL of diaphorase, 5 μM resazurin sodium salt and 50 mM TEA buffer (pH 8.1). The resulting fluorescence was measured using a fluorescence microplate reader (λex 530 nm, λem 590 nm). Quantification of 2-DG-6-phosphate was carried out by comparing the fluorescence intensity from the experimental samples to its standard curve.
Statistical analysis
All values were expressed as means ± SD. Statistical significance was analyzed by Student’s t-test or one-way ANOVA followed by Tukey’s HSD using XLSTAT software version 2014.3.04 (Addinsoft, Paris, France).
Results
The treatment of BITC during the differentiation-inducing stage decreased lipid accumulation
The tested concentration of BITC was determined to be at most 5 μM, because the cytotoxic effect of BITC toward 3T3-L1 was observed at more than 10 μM in an LDH-release assay (). To investigate the modulating effect of the BITC treatment on the lipid accumulation in 3T3-L1 preadipocytes, the cells were incubated in the differentiation-inducing medium for 3 days (early stage), then in the differentiation-maintaining medium for additional 3 days (late stage). The cells were treated with or without BITC for 6 days (both stages). Thereafter, the cells were cultured in DMEM containing 10% FBS for 2 days for maturing adipocytes. The intracellular lipid accumulation was measured by Oil Red O staining. As shown in (,)), lipid accumulation was significantly inhibited by the BITC treatment all through both stages. We next examined whether the treatment of BITC during the early stage or late stage modulates the lipid accumulation. As shown in (,d)), the treatment of BITC during the early stage significantly inhibited the lipid accumulation, whereas that during the later stage showed a tendency to enhance the lipid accumulation. These results suggested that its treatment during the early stage plays an important role in modulation of the lipid accumulation in the differentiating preadipocytes. In addition, another ITC compound, AITC, showed a weaker effect on the lipid accumulation in the differentiation-inducing (early) stage (e)) compared to that of BITC ().
Figure 1. Cytotoxic effect of BITC in 3T3-L1 preadipocytes.
3T3-L1 preadipocytes were incubated in induction medium with 0.2% Tween-20 as the positive control (PC) or indicated concentrations of BITC for 3 days. The cytotoxicity was determined by an LDH-release assay. All values were expressed as means ± SD of three separate experiments (*p < 0.05, **p < 0.01 compared to negative control).
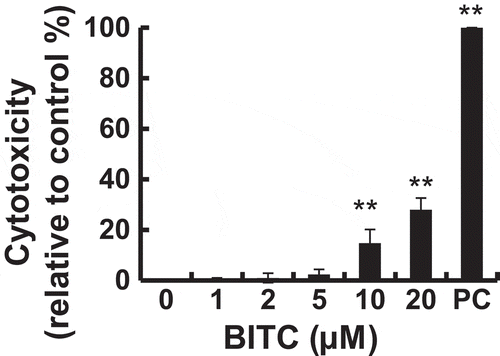
Figure 2. Modulating effects of BITC on lipid accumulation in 3T3-L1 adipocytes.
(A and B) The confluent 3T3-L1 adipocytes were incubated with the differentiation-inducing media for 3 days, then incubated in the differentiation-maintaining media for another 3 days. BITC was treated with the cells all through both stages for 6 days. Thereafter, the cells were cultured in the completed media for 2 days. (C) The confluent 3T3-L1 adipocytes were incubated with the differentiation-inducing media with or without BITC for 3 days (early stage treatment), then incubated in the differentiation-maintaining media for 3 days and in the completed media for 2 days. (D) The confluent 3T3-L1 adipocytes were incubated with the differentiation-inducing media for 3 days, then incubated in the differentiation-maintaining media with or without BITC (late stage treatment) for 3 days and in the completed media for 2 days. (E) The confluent 3T3-L1 adipocytes were incubated with the differentiation-inducing media with or without AITC for 3 days (early stage treatment), then incubated in the differentiation-maintaining media for 3 days and in the completed media for 2 days. The lipid accumulation level was determined by Oil Red O staining after an 8-day incubation. (A) Representative phase-contrast micrographs of the 3T3-L1 adipocytes treated with BITC (0, 1, 2 and 5 μM) for 6 days. (B-E) absorbance of the dissolved solution was measured at 490 nm using a microplate spectrophotometer. All values were expressed as means ± SD of three separate experiments (*p < 0.05, **p < 0.01 compared to negative control).
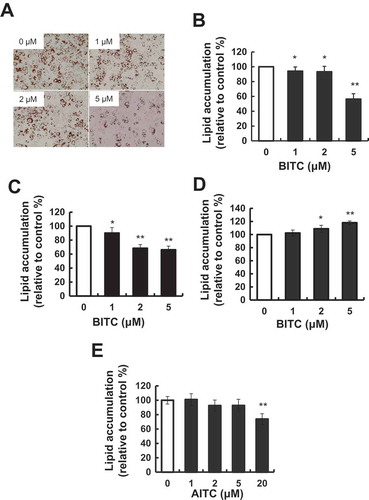
BITC inhibited the gene expressions of the adipogenic transcriptional factors
To determine whether BITC modulates the master markers of the adipocyte-specific differentiation, the expression levels of PPARγ and C/EBPα were determined by RT-PCR using the 3T3-L1 cells incubated in the differentiation-inducing medium with or without BITC for 3 days. The expression level of PPARγ was significantly decreased by 2 and 5 μM BITC to 87.0% and 46.1% of the control level, respectively (,)). The C/EBPα expression level was significantly decreased at the concentrations from 1 μM BITC and higher (,)). We next checked the effect of BITC on the early differentiation markers such as C/EBPβ and C/EBPδ. The expression level of C/EBPβ was significantly decreased by 5 μM BITC to 65.3% of the control level (,)). The C/EBPδ level was significantly decreased at the concentrations from 1 μM BITC and higher (,)). These results suggested that BITC could negatively regulate the gene expression of the adipogenic transcriptional factors in 3T3-L1 preadipocytes during the differentiation-inducing stage.
Figure 3. Suppression of the gene expression of adipogenic transcriptional factors by BITC.
The confluent 3T3-L1 adipocytes were incubated with the differentiation-inducing media with or without BITC for 3 days (early stage treatment), then the gene expression level was determined by RT-PCR. Quantitative data for (A) PPARγ, (B) C/EBPα, (C) C/EBPβ, and (D) C/EBPδ, and representative blots (E). All values were expressed as means ± SD of three separate experiments (*p < 0.05, **p < 0.01 compared to negative control).
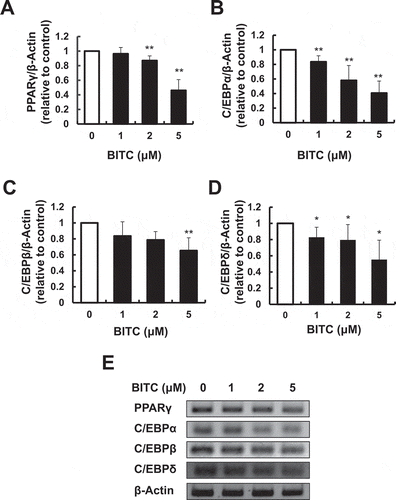
BITC modulated the phosphorylation of the adipogenic protein kinases
Since AMPK is known to be involved in the negative regulation of adipogenesis [Citation9], we examined whether the AMPK activation was involved in the modulating effect of BITC on the lipid accumulation. As shown in ()), the treatment of 5 μM BITC during the early stage for 3 days significantly increased the phosphorylation level of AMPK to 138% of the control level. In addition, the effect of BITC on the phosphorylation level of ERK1/2, one of the other protein kinases to regulate the adipocytes differentiation [Citation11], was determined by Western blotting. As shown in ()), the phosphorylation level of ERK1/2 was significantly decreased by even 1 μM BITC for 2 days after treatment.
Figure 4. Modulating effect of BITC on the phosphorylation of AMPK and ERK1/2.
The confluent 3T3-L1 adipocytes were incubated with the differentiation-inducing media with or without BITC for 3 days for the phosphorylated AMPK (A) or for 2 days for the phosphorylated ERK1/2 (B), then the protein expression level was determined by Western blotting. All values were expressed as means ± SD of three separate experiments (*p < 0.05, **p < 0.01 compared to negative control).
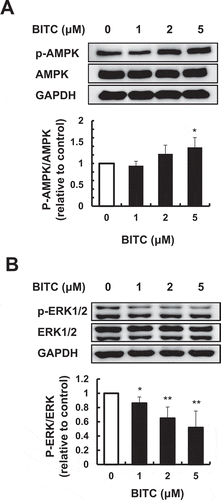
BITC inhibited the glucose uptake through transcriptional regulation
The expression level of GLUT4 was next determined, because it is one of the main target genes of C/EBPα and plays a critical role in glucose uptake by adipocytes [Citation8]. As shown in ()), the expression level of GLUT4 was significantly decreased by the treatment of 2 or 5 μM BITC (70.4% or 62.7% of the control level, respectively) during the early stage for 3 days. Thus, the effect of BITC on the insulin-independent glucose uptake was next examined. The glucose uptake in the BITC-treated 3T3-L1 cells was evaluated by an enzyme-dependent fluorometric assay with 2-DG, a widely-used and non-metabolizable glucose tracer [Citation25]. As shown in ()), the treatment of 5 μM BITC during the early stage for 3 days significantly suppressed the intracellular 2-DG level. These results suggested that BITC might suppress the glucose uptake by the GLUT4 down-regulation.
Figure 5. Inhibitory effects of BITC on the GLUT4 gene expression and glucose uptake.
The confluent 3T3-L1 adipocytes were incubated with the differentiation-inducing media with or without BITC for 3 days, then the gene expression level of GLUT4 was determined by RT-PCR (A) or the glucose uptake level was determined by an enzyme-dependent fluorometric assay (B). All values were expressed as means ± SD of three separate experiments (*p < 0.05, **p < 0.01 compared to negative control).
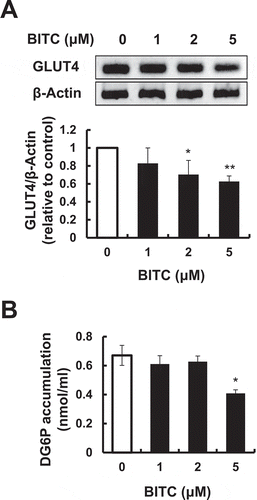
AMPK is involved in the inhibition of adipogenesis by BITC
To determine whether AMPK is involved in the modulating effect of BITC on lipid accumulation, the effects of an AMPK inhibitor, Compound C, on the glucose uptake and lipid accumulation in the 3T3-L1 cells incubated in the differentiation-inducing medium with or without BITC. As shown in ()), Compound C completely counteracted the BITC-induced suppression of the intracellular 2-DG level. Consistently, Compound C also impaired the inhibitory effect of BITC on the lipid accumulation ()). Compound C alone significantly enhanced the basal level of 2-DG in the 3T3-L1 cells, whereas it did not affect the basal level of lipid accumulation. These results suggested that AMPK might, at least partly, be involved in the mechanism underlying the suppression of the glucose uptake and lipid accumulation by BITC.
Figure 6. Counteracting effect of an AMPK inhibitor on the BITC-induced suppression of glucose uptake and lipid accumulation.
(A) The confluent 3T3-L1 adipocytes were incubated with the differentiation-inducing media treated with 5 µM BITC in the presence or absence of 2 µM Compound C for 3 days. The glucose uptake level was determined by an enzyme-dependent fluorometric assay. (B) The confluent 3T3-L1 adipocytes were incubated with the differentiation-inducing media treated with 5 µM BITC in the presence or absence of 2 µM Compound C for 3 days, then cultured in the differentiation-maintaining medium for 3 days. Thereafter, the cells were cultured in DMEM supplemented 10% FBS for another 2 days and stained with Oil Red O. All values were expressed as means ± SD of three separate experiments. Data were analyzed by a one-way ANOVA followed by Tukey’s HSD using XLSTAT software. Different letters above the bars indicate significant differences among treatments for each compound (p < 0.05).
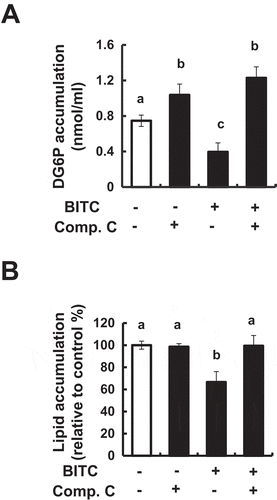
Discussion
In this study, we demonstrated that BITC is a potential inhibitor of the lipid accumulation in the differentiating preadipocytes. BITC improves the high-fat diet-induced liver fat accumulation in vivo [Citation23], thus supporting our findings. Although the continuous treatment of BITC during all the stages (differentiation-inducing, different-maintaining and maturing stages) has also been reported to inhibit the lipid accumulation [Citation26], its molecular mechanism remains to be clarified. The BITC treatment during the early stage, but not in the late stage, contributed to suppression of the lipid accumulation in the differentiating 3T3-L1 preadipocytes (,)). Therefore, BITC might influence the cellular signaling pathways activated by agents in the differentiation-inducing medium (IBMX and dexamethasone), but not that in the differentiation-maintaining medium (insulin). AITC, an aliphatic ITC, has the potential for inhibition of the adipocyte differentiation, which requires the relatively higher concentrations (15–30 μM) and longer incubation (8 days) [Citation27]. Our findings (,)) supported the lower potency of AITC than that of BITC. Sulforaphane, a different aliphatic ITC, is also effective for lipid accumulation at concentrations between 5 and 20 μM mainly through inhibition of the cell mitotic expansion [Citation28]. Esculetin, a coumarin compound derived from medicinal plants, inhibits adipogenesis by down-regulating the PPARγ signaling in 3T3-L1 cells at a concentration of more than 50 μM [Citation29]. A soybean isoflavone, genistein, shows an inhibitory effect on the lipid accumulation at 50 μM [Citation30]. Therefore, BITC is one of the most potent ITCs for inhibiting the lipid accumulation in 3T3-L1 preadipocytes, equivalent to the Ashitaba chalcones [Citation31].
BITC inhibited the gene expressions of the adipogenic transcriptional factors and the phosphorylation level of ERK1/2 with the increased level of AMPK phosphorylation ( and ). C/EBPα and PPARγ are the master regulators for adipogenesis, controlling the adipocyte differentiation through the expression of a variety of other transcriptional factors, such as fatty acid synthase, adipocyte fatty acid binding protein, GLUT4, and adiponectin, whose function leads to the formation of mature adipocytes [Citation32]. PPARγ is heterodimerized with retinoic acid X-receptor [Citation33] to regulate the transcription of the adipocyte-specific genes [Citation32]. C/EBPα is most abundant in mature adipocytes and mainly contributes to the enhanced glucose uptake [Citation32]. C/EBPβ and C/EBPδ are transiently expressed during early differentiation into adipocytes to increase the expression of other adipogenic transcriptional factors such as C/EBPα and PPARγ [Citation34]. Thus, BITC might suppress the C/EBPβ and C/EBPδ expressions at the earlier stage and subsequently suppress C/EBPα and PPARγ during the adipocyte differentiation. The expression of GLUT4, playing a critical role in glucose uptake in adipocytes, is regulated by C/EBP-α during the adipocyte differentiation [Citation32]. BITC suppressed the gene expression of GLUT4 ()), and also actually inhibited the glucose uptake ()). These results suggested that inhibition of the adipocyte differentiation and glucose uptake might play an important role in the inhibition of the lipid accumulation by BITC in 3T3-L1 preadipocytes.
AMPK plays critical roles in modulating many biological pathways including the lipid and glucose metabolism [Citation9]. ACC, a molecular target of AMPK [Citation9], is an essential enzyme for the synthesis of fatty acids. In addition, an AMPK inhibitor cancels the down-regulation of C/EBPα, C/EBPβ and PPARγ induced by the Ashitaba chalcones [Citation31]. Thus, inhibition of the adipocytes differentiation by BITC might involve the enhanced phosphorylation of AMPK. This idea was strongly supported by the finding that Compound C, the AMPK inhibitor, abolished the inhibitory effects of BITC on glucose uptake and lipid accumulation in 3T3-L1 preadipocytes (). These results suggested that the AMPK activation might, at least partly, contribute to the suppression of the glucose uptake and lipid accumulation by BITC, possibly through down-regulation of C/EBPs and PPARγ. In addition, attenuation of the ERK1/2 activation in preadipocytes led to a decrease in the adipocytes differentiation [Citation11]. However, there is a controversial report showing that the activation of ERK1/2 negatively regulated the adipocyte differentiation by the phosphorylation of PPARγ [Citation35]. The present results implied that the inhibition of the ERK1/2 phosphorylation by BITC during the early stage might contribute to the inhibitory effects on the adipocyte differentiation.
In conclusion, the present study provides evidence that BITC is one of the potential natural agents for the prevention against not only obesity, but also obesity-related chronic diseases, because adipocyte differentiation is associated with the pathogenesis and progression of type 2 diabetes, hypertension, and cardiovascular disease [Citation36]. Further studies are necessary to elucidate its more precise mechanisms of anti-adipogenesis not only using in vitro models, but also using in vivo animal models in terms of involvement of the adipocyte differentiation.
Disclosure statement
No potential conflict of interest was reported by the authors.
Additional information
Funding
References
- Unger RH, Clark GO, Scherer PE, et al. Lipid homeostasis, lipotoxicity and the metabolic syndrome. Biochim Biophys Acta. 2010;1801:209–214.
- Spiegelman BM, Flier JS. Adipogenesis and obesity: rounding out the big picture. Cell. 1996;87:377–389.
- Goto T, Takahashi N, Kawada T. Food components modulate obesity and energy metabolism via the transcriptional regulation of lipid-sensing nuclear receptors. J Nutr Sci Vitaminol (Tokyo). 2015;61:S128–S130.
- Wang S, Moustaid-Moussa N, Chen L, et al. Novel insights of dietary polyphenols and obesity. J Nutr Biochem. 2014;25:1–18.
- Goto T, Kim YI, Takahashi N, et al. Natural compounds regulate energy metabolism by the modulating the activity of lipid-sensing nuclear receptors. Mol Nutr Food Res. 2013;57:20–33.
- Farmer SR. Transcriptional control of adipocyte formation. Cell Metab. 2006;4:263–273.
- Siersbaek R, Nielsen R, Mandrup S. PPARgamma in adipocyte differentiation and metabolism-novel insights from genome-wide studies. FEBS Lett. 2010;584:3242–3249.
- Kaestner KH, Christy RJ, Lane MD. Mouse insulin-responsive glucose transporter gene: characterization of the gene and trans-activation by the CCAAT/enhancer binding protein. Proc Natl Acad Sci USA. 1990;87:251–255.
- Carling D, Mayer FV, Sanders MJ, et al. AMP-activated protein kinase: nature’s energy sensor. Nat Chem Biol. 2011;7:512–518.
- Gao Y, Zhou Y, Xu A, et al. Effects of an AMP-activated protein kinase inhibitor, compound C, on adipogenic differentiation of 3T3-L1 cells. Biol Pharm Bull. 2008;31:1716–1722.
- Sale EM, Atkinson PG, Sale GJ. Requirement of MAP kinase for differentiation of fibroblasts to adipocytes, for insulin activation of p90 S6 kinase and for insulin or serum stimulation of DNA synthesis. EMBO J. 1995;14:674–684.
- Nakamura T, Abe-Kanoh N, Nakamura Y. Physiological relevance of covalent protein modification by dietary isothiocyanates. J Clin Biochem Nutr. 2018;62:11–19.
- Nakamura Y, Miyoshi N. Electrophiles in foods: the current status of isothiocyanates and their chemical biology. Biosci Biotechnol Biochem. 2010;74:242–255.
- Nakamura Y, Yoshimoto M, Murata Y, et al. Papaya seed represents a rich source of biologically active isothiocyanate. J Agric Food Chem. 2007;55:4407–4413.
- Nakamura Y, Ohigashi H, Masuda S, et al. Redox regulation of glutathione S-transferase induction by benzyl isothiocyanate: correlation of enzyme induction with the formation of reactive oxygen intermediates. Cancer Res.. 2000;60:219–225.
- Liu Y, Yamanaka M, Abe-Kanoh N, et al. Benzyl isothiocyanate ameliorates acetaldehyde-induced cytotoxicity by enhancing aldehyde dehydrogenase activity in murine hepatoma Hepa1c1c7 cells. Food Chem Toxicol. 2017;108:305–313.
- Miyoshi N, Uchida K, Osawa T, et al. A link between benzyl isothiocyanate-induced cell cycle arrest and apoptosis: involvement of mitogen-activated protein kinases in the Bcl-2 phosphorylation. Cancer Res. 2004;64:2134–2142.
- Abe N, Okuhira M, Tsutsui C, et al. Cytotoxicity of benzyl isothiocyanate in normal renal proximal tubular cells and its modulation by glutathione. J Agric Food Chem. 2012;60:1887–1892.
- Abe N, Hou DX, Munemasa S, et al. Nuclear factor-kappaB sensitizes to benzyl isothiocyanate-induced antiproliferation in p53-deficient colorectal cancer cells. Cell Death Dis.. 2014;5:e1534.
- Murakami A, Matsumoto K, Koshimizu K, et al. Effects of selected food factors with chemopreventive properties on combined lipopolysaccharide- and interferon-γ-induced IκB degradation in RAW264.7 macrophages. Cancer Lett. 2003;195:17–25.
- Tang Y, Abe N, Yoshimoto M, et al. Benzyl isothiocyanate inhibits IL-13 expression in human basophilic KU812 cells. Biosci Biotechnol Biochem. 2015;79:159–163.
- Tang Y, Naito S, Abe-Kanoh N, et al. Benzyl isothiocyanate attenuates the hydrogen peroxide-induced interleukin-13 expression through glutathione S-transferase P induction in T lymphocytic leukemia cells. J Biochem Mol Toxicol. 2018;32:e22054.
- Alsanea S, Liu D. BITC and S-carvone restrain high-fat diet-induced obesity and ameliorate hepatic steatosis and insulin resistance. Pharm Res. 2017;34:2241–2249.
- Green H, Kehinde O. An established preadipose cell line and its differentiation in culture II. Cell. 1975;5:19–27.
- Yamamoto N, Ueda-Wakagi M, Sato T, et al. Measurement of Glucose Uptake in Cultured Cells. Curr Protoc Pharmacol. 2015;71:12–14,1–26.
- Kim M, Cho HJ, Kwon GT, et al. Benzyl isothiocyanate suppresses high-fat diet-stimulated mammary tumor progression via the alteration of tumor microenvironments in obesity-resistant BALB/c mice. Mol Carcinog. 2015;54:72–82.
- Lo CW, Chen CS, Chen YC, et al. Allyl isothiocyanate ameliorates obesity by inhibiting galectin-12. Mol Nutr Food Res. 2018;62:e1700616.
- Choi KM, Lee YS, Sin DM, et al. Sulforaphane inhibits mitotic clonal expansion during adipogenesis through cell cycle arrest. Obesity. 2012;20:1365–1371.
- Shin E, Choi KM, Yoo HS, et al. Inhibitory effects of coumarins from the stem barks of Fraxinus rhynchophylla on adipocyte differentiation in 3T3-L1 cells. Biol Pharm Bull. 2010;33:1610–1614.
- Zhang M, Ikeda K, Xu JW, et al. Genistein suppresses adipogenesis of 3T3-L1 cells via multiple signal pathways. Phytother Res. 2009;23:713–718.
- Zhang T, Sawada K, Yamamoto N, et al. 4-Hydroxyderricin and xanthoangelol from Ashitaba (Angelica keiskei) suppress differentiation of preadiopocytes to adipocytes via AMPK and MAPK pathways. Mol Nutr Food Res. 2013;57:1729–1740.
- Wu Z, Rosen ED, Brun R, et al. Cross-regulation of C/EBP alpha and PPAR gamma controls the transcriptional pathway of adipogenesis and insulin sensitivity. Mol Cell. 1999;3:151–158.
- Kliewer SA, Umesono K, Noonan DJ, et al. Convergence of 9-cis retinoic acid and peroxisome proliferator signalling pathways through heterodimer formation of their receptors. Nature. 1992;358:771–774.
- Yeh WC, Cao Z, Classon M, et al. Cascade regulation of terminal adipocyte differentiation by three members of the C/EBP family of leucine zipper proteins. Genes Dev. 1995;9:168–181.
- Hu E, Kim JB, Sarraf P, et al. Inhibition of adipogenesis through MAP kinase-mediated phosphorylation of PPAR gamma. Science. 1996;274:2100–2103.
- Ntambi JM, Young-Cheul K. Adipocyte differentiation and gene expression. J Nutr. 2000;130:3122S–3126S.