ABSTRACT
A chemoattractant of Ralstonia solanacearum isolated from the activated charcoal-adsorbed fraction of tomato root exudates was identified as ethyl β-d-glucopyranoside by instrumental analyses and comparison with synthetic preparations. Ethyl β-D-glucopyranoside showed unambiguous activity at above 1 µmol/disc. Its stereoisomers and D-glucose were inactive.
Graphical Abstract
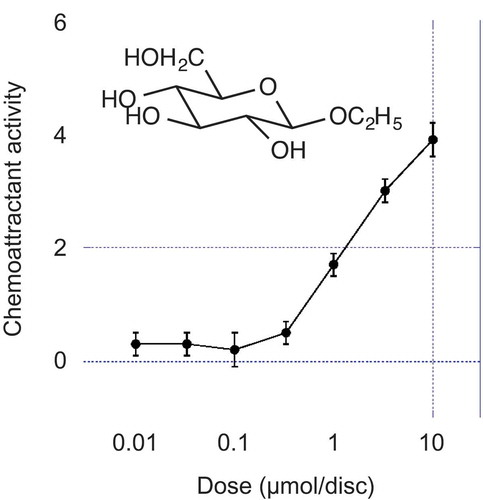
Structure of chemoattractant for Ralstonia solanacearum isolated from tomato root exudates with the guidance of bioassay was identified as ethyl β-D-glucopyranoside.
Chemotaxis is an important property for the infection of a phytopathogenic bacterium, Ralstonia solanacearum [Citation1,Citation2]. However, previous studies on chemotaxis have been limited to examining activities of root exudate constituents, such as amino acids, sugars and organic acids [Citation2,Citation3]. Such substances are secreted by diverse plant species, including non-host plants [Citation4,Citation5], therefore root exudate composition is unreasonable to explain the specific relationship between phytopathogenic bacteria and host plants.
However, several cases are known whereby a specific plant metabolite mediates interaction between a microorganism and a plant [Citation6]. Rhizobium species sense specific secondary metabolites, such as flavonoids, secreted by the host leguminous plant and establishes a mutual relationship with a symbiotic partner [Citation7]. In the chemotaxis of zoospores in zoosporic fungi, various types of species-specific attractants and repellents, such as flavonoids and terpenoids, have been reported [Citation8].
We hypothesized that a specific substance in root exudates from the host plant attracts Ralstonia solanacearum. In this study, we searched for a chemoattractant contained in tomato root exudates using a bioassay-guided approach. We examined the sensitivity and productivity of the chemoattractant with combinations of seven R. solanacearum strains and five tomato cultivars. Ralstonia solanacearum MAFF 730138 (Genebank Project, National Agriculture and Food Research Organization, Tsukuba, Japan) showed chemotactic activity toward the activated charcoal-adsorbed fraction of the root exudates of the tomato cultivar “Oogata-Fukuju”, which does not contain amino acids or organic acids. The chemoattractant was isolated by purification with diol-modified silica gel and NH2-modified silica gel column chromatograph y. Given that the isolated chemoattractant showed a color reaction with the α-naphthol–sulfuric acid reagent but not with 2,3,5-triphenyltetrazolium chloride, ninhydrin, Dragendorff or dinitrophenylhydrazine reagent, the compound was presumed to be a non-reducing and nitrogen-free sugar derivative [Citation9].
We report here elucidation of the structure of the active substance.
Results and discussion
The chemoattractant showed a cationized molecular ion at m/z 231.0840 in high-resolution ESI-MS spectrum, which suggested that the molecular formula was C8H16O6Na (Calcd. 231.0839) and the molecular weight of the attractant was 208. 13C-NMR (Figure S1) and DEPT spectra in CD3OD revealed the presence of a methine carbon that was presumed to be an anomeric carbon of a sugar (δ 104.1), four methine carbons connected to oxygen (δ 78.1–71.6), two methylene carbons (δ 66.2 and 62.7), and a methyl carbon (δ 15.5). A signal at δ 4.25 (d, J = 8.4 Hz) in the 1H-NMR(CD3OD, Figure S2) was assignable as an anomeric proton of a sugar from its chemical shift. Signals at δ 1.22 (3H, t, J = 7.1 Hz), δ 3.60 (1H, dd, J = 9.6, 7.1 Hz) and δ 3.87 (1H, dd, J = 9.6, 7.1 Hz) suggested that the attractant was ethyl glycoside. Comparison of 1H- and 13C-NMR data with those of Li et al. [Citation10] indicated that the attractant was ethyl β-glucopyranoside.
We, thus, synthesized ethyl β-glucopyranoside by applying the method of neighboring-group participation of an acetyl group at C-2 [Citation11] and ethyl α-glucopyranoside by Fischer glycosidation [Citation12] from D- and L-glucose. The products were compared with the natural attractant by HPLC and optical rotation. The Rt of the natural attractant was 14.7 min and was identical to that of the β-anomer . The α-anomer showed a longer Rt (15.4 min) than the β-anomer. Optical rotation of the natural attractant ([α]D – 33.9° (c 0.50, CH3OH)) coincided with that of the synthetic D-isomer ([α]D – 35.9° (c 0.50, CH3OH)) and that reported by Li et al. ([α]D – 35.7° (c 0.78, CH3OH) [Citation10], and was opposite in sign to that of the L-isomer ([α]D + 36.1° (c 0.50, CH3OH)). On the basis of these data, the chemoattractant was identified as ethyl β-D-glucopyranoside .
Figure 1. HPLC chromatograms of the natural chemoattractant and synthetic ethyl β- and α-D-glucopyranosides.
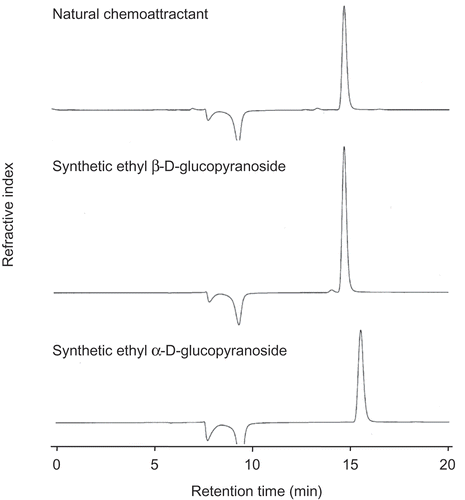
Figure 2. Structure of ethyl β-D-glucopyranoside, the chemoattractant isolated from tomato root exudates.
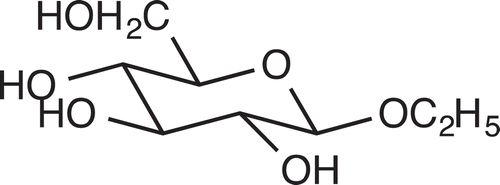
Chemoattractive activity of the ethyl β- and α-D-glucopyranosides was compared with that of L-glutamine, which is a strong chemoattractant for R. solanacearum [Citation2]. Ethyl β-D-glucopyranoside showed unambiguous activity above 1.0 µmol/disc, but the α-isomer did not show chemoattractive activity . The specific activity of ethyl β-D-glucopyranoside was about 1/30 of that of L-glutamine. Ethyl β- and α-L-glucopyranosides (Figure S3), free sugars including D-glucose, D-galactose, D-xylose and D-fructose, and di- and trisaccharides including maltose, lactose, cellobiose and maltotriose showed no chemoattractive activity at 3.3 and 10 µmol/disc.
Figure 3. Chemoattractive activities of ethyl β- and α-D-glucopyranosides and L-glutamine. ●: ethyl β-D-glucopyranoside; ○: ethyl α-D-glucopyranoside; ■: L-glutamine. Error bars indicate the standard error.
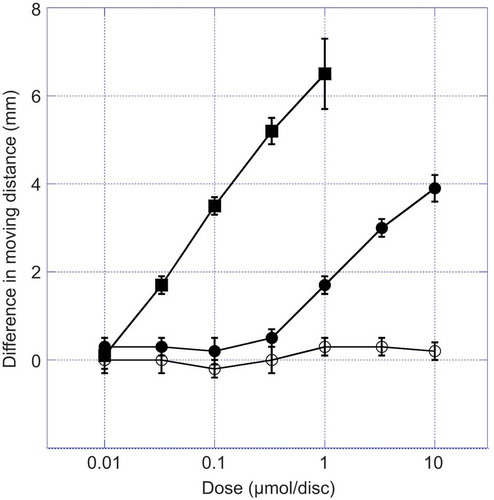
Isolated yield of ethyl β-D-glucopyranoside was 2.2 mg (11 µmol) from 1000 tomato seedlings [Citation9]. Considering loss during the purification process of ethyl β-D-glucopyranoside, the secretion amount is presumed to be comparable to free sugar contents in the root exudate of tomato (0.9–13.3 µmol/1000 seedlings [Citation13], 2.7–41.7 µmol/1000 plants [Citation14]), although free sugars were inactive. By contrast, the secretion amounts of amino acids with strong chemoattractive activity, including aspartic acid, proline, glutamine, isoleucine, leucine, glutamic acid and asparagine [Citation2], are lower than that of sugars (0.6–2.2 µmol/1000 plants [Citation15], 0.2–2.1 µmol/1000 seedlings [our unpublished data]), which is estimated to be 1/10 or less of the secretion amount of ethyl β-D-glucopyranoside. The result that the activity of ethyl β-D-glucopyranoside is weaker, whereas the secretion amount is higher, than those of glutamine, suggests that ethyl β-D-glucopyranoside functions in the rhizosphere.
We selected the tomato cultivar Oogata-fukuju from several cultivars. Oogata-fukuju is a cultivar that was developed in 1952 and older cultivars are generally susceptible to bacterial wilt. We are investigating the relationship between secretion amount of ethyl β-D-glucopyranoside and resistivity to bacterial wilt disease among tomato cultivars. We are also interested in the capability for ethyl β-D-glucopyranoside production by the host plants other than tomato and by non-host plants.
Ethyl β-D-glucopyranoside is reported to be a chemical constituent of tissues in certain plant species, such as peelings of yuzu (Citrus junos Sieb. (Rutaceae)) [Citation16], fruits of fennel (Foeniculum vulgare Miller (Umbelliferae)) [Citation17], cumin (Cuminum cyminum L. (Apiaceae)) [Citation18] and sea buckthorn (Hippophaë rhamnoides L. (Elaeagnaceae)) [Citation19]. To the best of our knowledge, its isolation from plant root exudates and chemoattractive activity for bacteria have not been reported previously. The physiological activities of ethyl β-d-glucopyranoside include a hypotensive effect in the stroke-prone spontaneously hypertensive rat [Citation16] and a bitter taste [Citation20]. Methyl β-D-glucopyranoside is also known to be a plant metabolite, but it was not detected in the course of our bioassay-guided purification of tomato root exudates. It is an interesting question whether the non-detectability is because of lack of activity or small amount of secretion. We are now synthesizing a variety of alkyl sugars to clarify the structure–activity relationship of the chemoattractant.
Strains of R. solanacearum are highly diverse and are characterized by host range, pathogenicity, epidemiological characteristics, and physiological and biochemical properties. We selected strain MAFF 730138 for the bioassay from among tomato isolates evaluated by responsiveness to L-glutamine and tomato root exudates [Citation9]. The sensitivity of various R. solanacearum strains to ethyl β-D-glucopyranoside and its relationship with pathogenicity to tomato, and the chemotactic activity of ethyl β-D-glucopyranoside for other bacterial species, and thus whether the chemoattractant is specific to R. solanacearum, is also of interest.
Experimental
1H and 13CNMR were recorded on a JNM-AL400 spectrometer (JEOL, Tokyo, Japan). Chemical shifts were reported as δ values; CD3OD (δH = 3.30, δC = 49.0) was used as an internal reference. Mass spectra were recorded on a micrOTOF-QII (Bruker-Daltonics, Yokohama, Japan). HPLC experiments were performed with a LC-10 system (Shimadzu, Kyoto, Japan) equipped with a RID-20A differential refractometric detector. An Inertsil Diol (5 µm, 4.6 × 250 mm) column (GL Science, Tokyo, Japan) with a mobile phase of CH3CN-H2O (2:98) and flow rate of 0.5 mL/min were used.
Synthesis of ethyl β-glucopyranoside
To a solution of D-glucose (1.00 g, 5.56 mmol) in dry pyridine (100 mL), acetic anhydride (15 mL) and dimethylaminopyridine (1.3 mg) was added, and the solution was stirred for 30 min at room temperature. Deionized water (100 mL) was added to the reaction mixture and the reaction product was extracted with EtOAc. The crude material was purified by silica gel column chromatography (MeOH/CHCl3 = 1:99) to afford pentaacetyl D-glucose (1.53 g, 3.92 mmol).
To a solution of pentaacetyl D-glucose (1.53 g, 3.92 mmol) in dry CH2Cl2 (40 mL), dry EtOH (0.5 mL) and BF3·OEt2 (0.5 mL, Tokyo Chemical Industry, Tokyo, Japan) were added and the solution was kept at 0°C for 10 h. The reaction was stopped by adding triethylamine (1 mL) and satd. aq. NaHCO3 (30 mL). The reaction product was extracted with EtOAc. The crude product was purified by silica gel column chromatography (EtOAc/hexane = 1:1) to afford ethyl D-glucopyranoside tetraacetate (1.12 g, 2.98 mmol).
To a solution of ethyl D-glucoside tetraacetate (0.500 g, 1.33 mmol) in EtOH (60 mL), 1% phenolphthalein ethanol solution (0.1 mL, Fujifilm Wako Pure Chemical, Osaka, Japan) and 28% NaOMe methanol solution (0.3 mL, Fujifilm Wako Pure Chemical, Osaka, Japan) were added and the solution was stirred for 10 min. The reaction was stopped by adding 1N HCl, and the reaction mixture was neutralized and evaporated to dryness in vacuo. The reaction product was desalinized using an active charcoal column and purified by silica gel column chromatography (MeOH/CHCl3/H2O = 4:5:1) to afford ethyl β-D-glucopyranoside (0.203 g, 0.976 mmol, overall yield 39%). The L-isomer was synthesized using the same method.
Synthesis of ethyl α-glucopyranoside
To a 3% hydrogen chloride methanol solution (30 mL, Kishida Chemical, Osaka, Japan), D-glucose (100 mg, 0.556 mmol) was added, and the solution was heated to reflux for 16 h. The reaction mixture was neutralized with satd. aq. NaHCO3 and evaporated to dryness. The white residue was dissolved in deionized water and the solution was passed through an active charcoal column. The adsorbed product was eluted with 80% aq. acetone. The eluted material was purified using a NH2-silica gel column (MeOH/CHCl3/H2O = 4:5:1) and ethyl α-D-glucopyranoside was obtained (87.6 mg, 0.421 mmol, yield 75.7%). The L-isomer was synthesized using the same method.
Chemotaxis assay
Chemoattractant activity was assayed by the semi-solid agar plate method using R. solanacearum MAFF 730138 as the assay strain [Citation9]. The bioassay with ethyl β-D-glucopyranoside was conducted three times in triplicate (n = 9), those with ethyl α-D-glucopyranoside and glutamine were conducted twice (n = 6) and those with ethyl β- and α-L-glucopyranoside were conducted once (n = 3). The movement distance to the sample disc and that to the negative control disc were measured on the third and seventh days (Figure S3). The difference in movement distances to the sample disc and to the negative control disc at the seventh day and standard errors were calculated and are plotted in . At a glutamine concentration of 1.0 µmol/disc or higher, the bacteria reached the sample disc and thereafter showed no further movement; glutamine also affected the movement to the control disc side (Figure S3), thus the data are not presented in .
Author contributions
The study was conceived by AOo and MN. YK established the bioassay method. AOk contributed to the selection of the R. solanacearum bioassay strain and the tomato cultivar. TH isolated the chemo attractant and elucidated its structure. HK contributed to the experimental design and data analysis. The manuscript was written by TH and MN, and then reviewed by all authors.
Supplemental Material
Download MS Power Point (1.9 MB)Acknowledgments
The authors thank Dr Kazuhiro Nakaho (National Agriculture and Food Research Organization) for technical advice on handling R. solanacearum.
Disclosure statement
No potential conflict of interest was reported by the authors.
Supplementary material
Supplemental data for this article can be accessed here
Additional information
Funding
References
- Tans-Kersten J, Huang H, Allen C. Ralstonia solanacearum needs motility for invasive virulence on tomato. J Bacteriol. 2001;183(12):3597–3605.
- Yao J, Allen C. Chemotaxis is required for virulence and competitive fitness of the bacterial wilt pathogen Ralstonia solanacearum. J Bacteriol. 2006;188(10):3697–3708.
- Hida A, Oku S, Kawasaki T, et al Identification of the mcpA and mcpM genes, encoding methyl-accepting proteins involved in amino acid and L-malate chemotaxis, and involvement of McpM-mediated chemotaxis in plant infection by Ralstonia pseudosolanacearum (formerly Ralstonia solanacearum phylotypes I and III). Appl Environ Microbiol. 2015;81(21):7420–7430.
- Kamilova F, L V K, A I S, et al Organic acids, sugars, and L-tryptophane in exudates of vegetables growing on stonewool and their effects on activities of rhizosphere bacteria. Mol Plant-Microbe Interact. 2006;19(3):250–256.
- Kravchenko LV, Shapozhnikov AI, Makarova NM, et al Exometabolites of bread wheat and tomato affecting the plant-microbe interactions in the rhizosphere. Russ J Plant Pathol. 2011;58(5):936–940.
- Yoneyama K, Natsume M. Allelochemicals for plant-plant and plant-microbe interactions. In: Mori K, editor. Comprehensive natural products II, vol. 4, chemistry and biology. Amsterdam: Elsevier; 2010. p. 539–561.
- Schlaman HRM, Philip DA, Kondorosi E. Genetic organization and transcriptional regulation of rhizobial nodulation genes. In: Spaink HP, Kondorosi A, Hooykaas PJJ, editors. The Rhizobiaceae. Dordrecht: Kluwer Academic Publishers; 1998. p. 361–386.
- Islam Md T, Tahara S. Chemotaxis of fungal zoospores, with special reference to Aphanomyces cochlioides. Biosci Biotechnol Biochem. 2001;65(9):1933–1948.
- Hasegawa T, Okabe A, Kato Y, et al Bioassay-guided isolation of a novel chemoattractant for Ralstonia solanacearum in tomato root exudates. J Gen Plant Pathol. 2018;84(1):20–26.
- Li W, Koike K, Asada Y, et al Biotransformation of low-molecular-weight alcohols by Coleus forskohlii hairy root cultures. Carbohydr Res. 2003;338:729–731.
- Ellervik U, Jansson K, Magnusson G. Gas chromatographic investigation of the boron trifluoride etherate-induced formation and anomerization of glucopyranosides. J Carbohydr Chem. 1998;17(4&5):777–784.
- Helferich B, Schäfer W. Org Syn Coll. 1941;1:364.
- Kravchenko LV, Azarova TS, Leonova-Erko EI, et al. Root exudates of tomato plants and their effect on the growth and antifungal activity of Pseudomonas strains. Microbiol (Moscow). 2003;72(1):37–41.
- Kamilova F, Kravchenko LV, Shaposhinikov AI, et al. Effects of the tomato pathogen Fusarium oxysporum f. sp. Radices-lycopersici and of the biocontrol bacterium Pseudomonas fluorescens WCS365 on the composition of organic acids and sugars in tomato root exudate. Mol Plant-Microbe Interact. 2006;19(10):1121–1126.
- Balasubramanian A, Rangaswami G. Influence of foliar nutrient sprays on the root exudation pattern in four crop plants. Plant Soil. 1969;30(2):210–220.
- Matsubara Y, Mizuno T, Sawabe A, et al Structure and physiological activity of nitrogenous compounds and alkyl glycosides in lemon (Citrus limon Burm. f.), unshiu (Citrus unshiu Marcov.), hassaku (Citrus hassaku Hort.), yuzu (Citrus junos Sieb.) and iyokan (Citrus iyo) peelings. Nippon Nogeikagaku Kaishi (in Japanese). 1989;63(8):1373–1377.
- Kitajima J, Ishikawa T, Tanaka Y. Chem Pharm Bull. 1998;46(10):1643–1646.
- Takayanagi T, Ishikawa T, Kitajima J. Water-soluble constituents of funnel. I Alkyl Glycosides. Phytochemistry. 1998;63(4):479–484.
- K M T, Yang B, G G H, et al Fast analysis of sugars, fruit acids, and vitamin C in sea buckthorn (Hippophaë rhamnoides L.) varieties. J Agric Food Chem. 2006;54(7):2508–2513.
- Birch GG, Lindley MG. Structuralfunctions of taste in the sugar series: effect of aglycones on the sensory properties of simple glycoside structures. J Food Sci. 1974;38(4):665–667.