ABSTRACT
A new facile method was developed for simple green synthesis of methylene-bridged phloroglucinol oligomers using nonthermal dielectric barrier discharge (DBD) plasma in methanolic solution. The chemical structures of these newly generated oligomers 2–5 were determined by interpretation of the spectroscopic data, and the inhibitory activity toward α-glucosidase of all isolates was evaluated. The unusual phloroglcuinol pentamer 5 connected by four methylene linkages showed a much higher potential inhibitory effect against α-glucosidase than the other generated oligomers 2–4 and appeared to be a promising lead for development as a potential antidiabetic agent.
Abbreviations: T2DM, type2 diabetes mellitus; DBD, dielectric barrier discharge; HPLC, high-performance liquid chromatography; IC50, 50% inhibition concentration; NMR, nuclear magnetic resonance; FABMS, fastatom bombardment mass spectrometry
Graphical Abstract
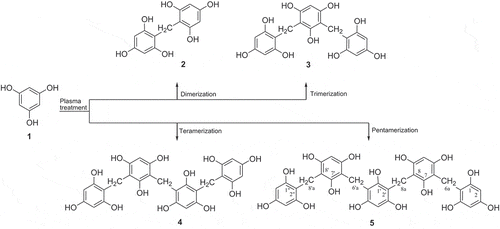
A new method was developed for simple green synthesis of methylene-bridged phloroglucinol oligomers 2–5 with potent α-glucosidase inhibitory activities using nonthermal.
Diabetes mellitus, which is one of the major common chronic diseases of the 21st century, belongs to a group of metabolic diseases defined by high blood glucose levels. Diabetes mellitus is closely associated with cardiovascular disease and is a major cause of morbidity and mortality [Citation1]. Moreover, prolonged high blood glucose levels in diabetes patients can lead to serious diseases that affect the heart, blood vessels, eyes, kidneys, and nerves [Citation2]. Currently, the most effective therapy for type 2 diabetes mellitus (T2DM) is to regulate optimal blood glucose levels after eating. α-Glucosidase is a key enzyme involved in the digestive system that hydrolyzes dietary carbohydrates and eventually produces absorbable glucose [Citation3]. Thus, α-glucosidase inhibitors are considered to be valuable therapeutic agents for treating T2DM. Several α-glucosidases, including Acarbose, Voglibose, and Miglitol, can reversibly inhibit α-glucosidase, consequently delaying the absorption of sugars from the gut; therefore, these compounds have been used clinically in the treatment of T2DM [Citation4]. However, only a few α-glucosidase inhibitors are commercially available, and they are all sugar entities that require complex steps during preparation and can lead to gastrointestinal side-effects such as flatulence and diarrhea. Thus, the discovery of new chemical entities with potential α-glucosidase inhibitory effects have attracted a great deal of attention in recent years [Citation5,Citation6].
Both synthetic and natural phloroglucinol derivatives have shown a vast array of biological efficacies including anti-inflammatory, antimicrobial, antiallergic, and antioxidant properties [Citation7]. Although many phloroglucinols have shown promising possibilities as a new class of drug candidate in various bioassay systems, very few have reached clinical therapy. Several recent reports have focused on the development of new biological activities of these phloroglucinol analogues from natural origins, but relatively few have described their α-glucosidase inhibitory effects [Citation8]. Moreover, the small isolated yields from compounds of natural origin have greatly limited their application. Therefore, a robust synthetic methodology could be better to developed to obtain chemicals possessing potent α-glucosidase inhibitory capacity. Most synthetic methods of producing pharmacological agents require harsh reaction conditions, prolonged reaction time, and tedious extraction procedures and generate oily products [Citation9,Citation10]. Therefore, some novel green methods have been developed to overcome these difficulties on the basis of increasing economic and ecological pressure [Citation11,Citation12]. We recently demonstrated that nonthermal plasma treatment is an effective environmentally friendly technology enabling more rapid, safer and higher chemical yields than conventional synthetic methods for generation of structurally modified natural products with enhanced bioactivity [Citation13,Citation14]. Dielectric barrier discharge (DBD) plasma is known to cause several chemical changes related to abundant generation of reactive oxygen and nitrogen species. However, the advantageous use of plasma treatment technique for induction of various chemical reactions during construction of drug-like molecules with different backbones is still very limited. We report the effective and simple structural condensation of phloroglucinol assisted by DBD plasma treatment. This method resulted in the formation of a new phloroglucinol pentamer 5 and three known phloroglucinol oligomers 2–4 with significantly enhanced biological properties against α-glucosidase compared to the parent compound phloroglucinol.
Results and discussion
A prepared solution containing phloroglucinol (400 mg) in methanol (2.0 L) was directly subjected to DBD plasma for 20, 40, and 60 min [Citation15], during which time condensation patterns were monitored by reversed-phase HPLC (). The dried phloroglucinol treated for 60 min showed the most improved inhibitory activity, with an IC50 value of 15.6 ± 1.7 μg/mL in the α-glucosidase inhibition assay. Chromatographic separation resulted in the isolation of a novel methylene-bridged pentameric phloroglucinol 5, along with three previously known oligomeric phloroglucinols, bis(2,4,6-trihydroxyphenyl)methane (2) [Citation16], 2,4- bis (2ʹ,4ʹ,6ʹ-trihydroxybenzyl)phloroglucinol (3) [Citation16], and bis (2,4,6-trihydroxy-3-(2ʹ4’,6ʹ-trihydroxybenzyl)phenyl)methane (4) [Citation16] (). The isolated compounds were identified by comparison of their spectroscopic data with the literature values.
Figure 2. Selected HMBC correlations of 5 (a) and HPLC chromatogram of plasma treated phloroglucinol for 60 min (b).
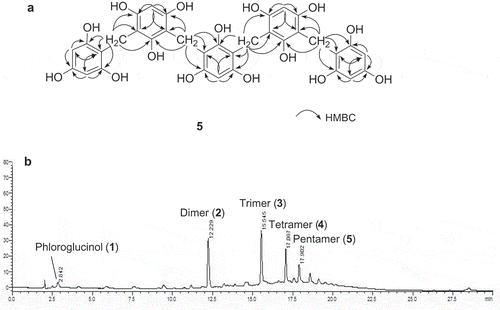
Compound 5 was obtained as a new compound in the form of a brown amorphous powder and showed a dark blue color in the FeCl3 test. A pseudomolecular ion peak at m/z 677.1507 [M−H]− (calculated 677.1506) observed in the negative HRFABMS in combination with 1H and 13C NMR spectroscopic data corresponded to a molecular formula of C34H30O15. The UV spectrum showed a peak at 279 nm with a shoulder at 228 nm that is typical of a phloroglucinol derivatives [Citation17]. The 1H NMR spectrum of 5 () at lower field displayed signals suggestive of a three 1,2,3,4,5-pentasubstitued isolated aromatic rings at δH 6.05 (3H, s, H-4ʹ, −10, −10ʹ) and two 1,2,3,4-tetrasubstituted aromatic ring systems at δH 5.92 (4H, s, H-2, −4, −4ʹ’, −6ʹ’), which further suggests the presence of the phloroglucinol skeleton. In addition to these signals, the 1H NMR spectrum of 5 in the other moiety also revealed the resonances for four characteristic methylene signals at δH 3.72 (4H, s, H-6a, −8ʹa) and δH 3.67 (4H, s, H-6ʹa, −8a). Consistent with these 1H NMR observations, the 13C NMR, HSQC, and DEPT experiments of 5 further displayed the presence of signals for three pentasubstituted aromatic rings at δc 154.9 (C-9, −11ʹ), 154.8 (C-3ʹ, −5ʹ), 154.7 (C-9ʹ, −11), 153.1 (C-1ʹ, −7, −7ʹ), 108.2 (C-8ʹ, −12ʹ), 108.0 (C-2ʹ, −6ʹ), 107.0 (C-8, −12ʹ), 97.2 (C-4ʹ, −10, −10ʹ), two 1,2,3,4-tetrasubstituted aromatic moieties at δc 158.1 (C-3, −5ʹ’), 156.5 (C-3ʹ’, −5), 156.4 (C-1, −1ʹ’), 108.5 (C-2ʹ’, −6), 96.5 (C-2, −4, −4ʹ’, −6ʹ’), and four characteristic methylene carbons at δc 18.5 (C-6a, 8ʹa) and 17.9 (C-6ʹa, −8a) (). Interestingly, the symmetrical structure would lead to virtually identical chemical shifts for both at δH 6.05 and δC 97.2 (H-4ʹ, −10, −10ʹ) and δH 5.92 and δC 96.5 (H-2, −4, −4ʹ’, −6ʹ’). Comparison of the 1H and 13C NMR spectroscopic data of 5 with those of previously reported synthetic bis (2,4,6-trihydroxy-3-(2ʹ4’,6ʹ-trihydroxybenzyl)phenyl)methane (4) [Citation16] showed the signals of both compounds to closely resemble one another, but the resonances for the absence of an aromatic proton at the C-12 position in 5, and the presence of two aromatic protons at C-2, −4 and one methylene proton at C-6a, indicating that a phloroglucinol moiety was linked through the methylene bridge in 5. The linkage positions of the methylene bridge residues in 5 were unambiguously elucidated by key HMBC correlations as shown in ). Thus, the unusual structure of compound 5 was elucidated as shown, and this compound was assigned the trivial name pentaphlorin.
Table 1. 1H and 13C NMR shifts of compound 5.a.
DBD plasma processing of pure phloroglucinol was conducted at room temperature for three each different treated times of 20, 40, and 60 min, which successfully produced phloroglucinol oligomers 2–5 with excellent synthesis rate in aproximately 90% isolated yield from parent phloroglucinol (1) for 60 min treated sample (See Experimental section). To estimate the relationship between α-glucosidase inhibitory activity and degree of condensation of the newly generated compounds, the contents of the active compounds were estimated by reversed-phase HPLC ()) while the condensation pattern of phloroglucinol (1) by plasma treatment was observed in the reacted mixture after 60 min. As the treatment time increased, the formation of relatively inactive phloroglucinol completely disappeared, and the major products 2 and 3 reached maximum synthesis rates up to 29.1% and 31.1% isolated yield in the 60 min phloroglucinol reactant, respectively. Additionally, increasing oligomeric phloroglucinols contents were readily detected by plasma treatment for 60 min ()).
All pure isolated phloroglucinol oligomers obtained in the current investigation were evaluated for their effect on degree of oligomerization degree against the α-glucosidase [Citation18] using acarbose as the positive control. The α-glucosidase inhibitory effects of further oligomerized phloroglucinol tetramer (4) and pentamer (5) were found to be significantly improved over that of their inactive parent phloroglucinol (1) (). Pentaphlorin (5) is a new highly oligomerized phloroglucinol derivative with the methylene bridges between both phloroglucinol units that displayed promising α-glucosidase inhibitory activity with an IC50 value of 2.3 ± 0.4 μM.
Table 2. Inhibitory effects of isolated compounds 2 – 5 on α-glucosidase.
Oligomeric phloroglucinol analogues with methylene linkage have previously been postulated to be crucial for antimalarial and anti-inflammatory activities [Citation19]. Naturally occurring methylene-bridged phloroglucinol derivatives are extremely rare, and the effects of antidiabetic capacity on oligomerization of phloroglucinol through a methylene bridge by plasma treatment are still uncertain. Several synthetic approaches of phloroglucinol with promising biological properties have been developed and commercialized for the purpose of clinical therapies [Citation20]. Here, we describe the oligomerization and isolation of rare phloroglucinol derivatives condensed from original phloroglucinol and report that oligomerization through a methylene linkage is distinctly associated with increased anti-diabetic efficacy.
Conclusion
In the current study, we first established that phloroglucinol (1) can be conveniently oligomerized into a new compound, pentaphlorin (5), as well as a known phloroglucinol dimer (2), phloroglucinol trimer (3), and phloroglucinol tetramer (4). The chemical structures of the newly condensed compounds were elucidated by detailed 1D- and 2D-NMR experiments as well as HRFABMS data interpretation. This study reports the successful green synthesis of potential anti-diabetic phloroglucinol oligomers using a plasma apparatus. This green chemical methodology required less time, cost saving, and used simpler reaction conditions than conventional synthetic methods. Moreover, the newly produced phloroglucinol oligomers exhibited moderate to promising antidiabetic activities against α-glucosidase. The results of our current study suggest that the readily available oligomerization of phloroglucinol induced by DBD plasma could be of interest to design of a new class anti-diabetic agents enabling more rapid, safer and higher chemical yield from products of natural origin. Based on these results, plasma induced oligomerization is a very clean and convenient method that will greatly facilitate pursuit of development of new classes of antidiabetic agents from inactive natural products. Further studies to acquire more information to enable structure-activity relationships are in progress.
Experimental
Chemicals and instruments
Phloroglucinol and acarbose and were purchased from Sigma-Aldrich (St. Louis, MO, USA). All other chemicals used in this study were of analytical grade. UV spectra were obtained using a Hitachi U-2000 spectrophotometer (Hitachi, Tokyo, Japan). 1H and 13C NMR spectra were measured on a Varian VNS600 instrument (Varian, Palo Alto, CA, USA) operated at 600 and 150 MHz, respectively. Chemical shifts are given in δ (ppm) values relative to those of the solvent CD3OD (δH 3.35; δC 49.0) on a tetramethylsilane (TMS) scale. The standard pulse sequences programmed into the instruments were used for each 2D measurement. The JCH value was set at 8 Hz in the HMBC spectra. FABMS were obtained on a Micro Mass Auto Spec OA-TOF spectrometer (Micromass, Manchester, UK). Column chromatography was performed using YMC GEL ODS AQ 120-50S (YMC Co., Kyoto, Japan).
Sample preparation
Phloroglucinol solutions (400 mg of phloroglucinol in 2 L of methanol) were prepared for each treatment and diluted before use. The DBD plasma treatment was carried out using a previously installed on the inner walls of a rectangular, parallelepiped plastic container (240 × 200 × 100 mm). The actuator was made of copper electrodes and a polytetrafluoroethylene sheet and attached to the inner walls of the container. A bipolar square-waveform voltage at 15 kHz was applied to one electrode while the other electrode was grounded. The plasma was generated inside the container with an input power of 250 W. The phloroglucinol solutions were placed in a glass dish at the bottom of the container and treated with plasma for 20, 40, and 60 min. The treated solutions were concentrated immediately using a vacuum evaporator to remove methanol. High-performance liquid chromatography (HPLC) (Shimadzu, Tokyo, Japan) with a photodiode-array (PDA) detector was used for the chromatographic analysis of phloroglucinol oligomers induced by plasma treatment. HPLC analysis was carried out using a YMC-Pack ODS A-302 column (4.6 mm i.d. × 150 mm; YMC Co., Kyoto, Japan), and the solvent system consisted of a gradient mode with 1% HCOOH and CH3CN.
New compounds isolation
A part of the dried exposed phloroglucinol for 60 min (150 mg) was directly passaged to column chromatography over a YMC GEL ODS AQ 120-50S column (2.5 cm i.d. x 42 cm) with aqueous MeOH, to yield pure compounds 2 (43.7 mg, tR 12.2 min), 3 (46.7 mg, tR 15.5 min), 4 (27.9 mg, tR 17.0 min), and 5 (16.3 mg, tR 17.9 min). Reversed-phase HPLC monitoring was accompanied over a YMC-Pack ODS A-302 column (4.6 mm i.d. × 150 mm; YMC Co., Kyoto, Japan), and the gradient solvent system started with 1% formic acid/H2O (flow rate: 1.0 ml/min; detection: UV 280 nm; temperature: 40°C), increased to CH3CN over 30 min.
Assay of α-glucosidase inhibitory activity
A previously reported method with a minor modification [Citation18] was used to evaluate the ability of compounds to inhibit α-glycosidase. Briefly, α-glucosidase (1 μL; EC 3.2.1.20) was incubated in 100 mmol L−1 potassium phosphate buffer (pH 6.8). Sample solution (1 μL) was premixed with 94 μL of 100 mmol L−1 potassium phosphate buffer (pH 6.8). After incubation at 37.5°C for 20 min, substrate (3 mmol L−1 p-NPG) was added to initiate the reaction. The reaction mixture was incubated at 37.5°C for 30 min, after which 100 μL of 0.1 mol L−1 Na2CO3 was added to stop the reaction. The amount of released p-nitrophenol was measured at 410 nm using a UV microplate reader (Infinite F200; Tecan Austria GmBH, Grödig, Austria). The half-maximal inhibitory concentration (IC50) value was calculated by linear regression analysis of activity under the assay conditions. Acarbose was used as a positive control and all assays were conducted in triplicate.
Pentaphlorin (5): brown amorphous powder; UV λ max MeOH nm (log ε): 228 (4.51), 228 (2.37); 1H and 13C NMR, see ; FABMS m/z 677 [M−H]−, HRFABMS m/z 677.1507 [M−H]− (calcd for C34H30O15, 677.1506); 1H and 13C NMR: see .
Author contributions
C.J. and T.H.K. designed the research and prepared the manuscript and S.C., G.H.J., and K.B.L. contributed to the experiments of structure elucidation a biological evaluation, assisted in the design and interpretation of research.
Supporting_information.docx
Download MS Word (727.1 KB)Disclosure statement
No potential conflict of interest was reported by the authors.
Supplemental material
Supplemental data for this article can be accessed here.
Additional information
Funding
References
- Anselmino M. Cardiovascular prevention in type 2 diabetes mellitus patients: the role of oral glucose-lowering agents. J Diabetes Complications. 2009;23:427−433.
- Avogaro A, Fadini GP. The effects of dipeptidyl peptidase-4 inhibition on microvascular diabetes complications. Diabetes Care. 2014;37:2884−2894.
- Israili ZH. Advances in the treatment of type 2 diabetes mellitus. Am J Ther. 2011;18:117−152.
- Asano N, Tomioka E, Kizu H, et al Sugars with nitrogen in the ring isolated from the leaves of Morus bombycis. Carbohydr Res. 1994;253:235–245.
- Benalla W, Bellahcen S, Bnouham M. Antidiabetic medicinal plants as a source of alpha glucosidase inhibitors. Cur Diabetes Rev. 2010;6:247–254.
- Pawar NJ, Parihar VS, Khan A, et al Quarternary indolizidine and indolizidone iminosugars as potential immunostimulating and glycosidase inhibitory agents: synthesis, conformational analysis, biological activity, and molecular docking study. J Med Chem. 2015;58:7820−7832.
- Singh IP, Sidana J, Bansal P, et al Phloroglucinol compounds of therapeutic interest: global patent and technology status. Expert Opin Ther Pat. 2009;19:847−866.
- Moon HE, Islam N, Ahn BR, et al Protein tyrosine phosphatase 1B and α-glucosidase inhibitory phlorotannins from edible brown algae, Ecklonia stolonifera and Eisenia bicyclis. Biosci Biotechnol Biochem. 2011;75:1472−1480.
- Han J, Jones AX, Lei X. Recent advances in the total synthesis of prenylflavonoid and related Diels–alder natural products. Synthesis. 2015;47:1519−1533.
- Wang Z, Yang L, Yang X, et al Advances in the first total synthesis of natural flavonoids. Synth Commum. 2013;43:3093−3114.
- Bernini R, Pasqualetti M, Provenzano G, et al Ecofriendly synthesis of halogenated flavonoids and evaluation of their antifungal activity. New J Chem. 2015;39:2980−2989.
- Macquarrie DJ, Nazih R, Sebti S. KF/natural phosphate as an efficient catalyst for synthesis of 2′-hydroxychalcones and flavanones. Green Chem. 2002;4:56−59.
- Moreau M, Orange N, Feuilloley MGJ. Non-thermal plasma technologies: new tools for bio-decontamination. Biotechnol Adv. 2008;26:610−617.
- Jeong GH, Cho JH, Kim SH, et al Plasma-induced dimerization of phloridzin as a new class of anti-adipogenic agents. Bioorg Med Chem Lett. 2017;27:4889−4892.
- Kim TH, Lee J, Kim HJ, et al Plasma-induced degradation of quercetin associated with the enhancement of biological activities. J Agric Food Chem. 2017;65:6929−6935.
- Kiehlmann E, Lathioor EC, Lai KW. Condensation of formaldehyde with phloroglucinol and its monomethyl ether. Org Prep Proced Int. 1996;28:185−192.
- Okada Y, Ushimaru A, Suzuki R, et al A new phloroglucinol derivative from the brown alga Eisenia bicyclis: potential for the effective treatment of diabetic complications. J Nat Prod. 2004;67:103−105.
- Kim YM, Wang MH, Rhee HI. A novel alpha-glucosidase inhibitor from pine bark. Carbohydr Res. 2004;339:715−717.
- Singh IP, Bharate SB. Phloroglucinol compounds of natural origin. Nat Prod Rep. 2006;23:558−591.
- Singh IP, Sindana J, Bharate SB, et al Phloroglucinol compounds of natural origin: synthetic aspects. Nat Prod Rep. 2010;27:393−416.