ABSTRACT
A majority of the potential health benefits of green tea, including the potential to prevent cognitive decline, have been attributed to epigallocatechin gallate (EGCG). Sunrouge is a green tea cultivar that contains EGCG and several other bioactive components such as quercetin, myricetin, cyanidin and delphinidin. We compared the effects of Sunrouge and Yabukita, the most popular Japanese green tea cultivar, on cognitive function in the senescence-accelerated mouse Prone8. These mice were fed an experimental diet containing Sunrouge extract (SRE) or Yabukita extract (YBE). SRE feeding significantly prevented cognitive decline, whereas YBE feeding had little effect. Moreover, SRE feeding prevented elevation of the amyloid-β42 level while improving the gene expression of neprilysin and decreasing beta-site APP-cleaving enzyme 1 in the brain. These preventive effects of SRE against cognitive decline were attributed to the characteristic composition of Sunrouge and strongly suggest that consumption of this cultivar could protect against age-related cognitive decline.
Graphical Abstract
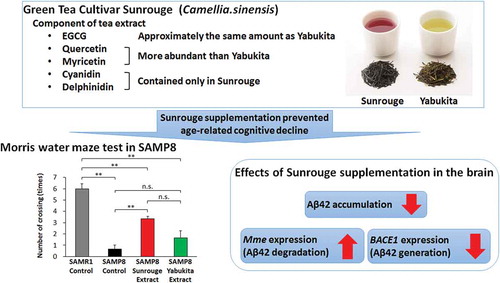
Long-term supplementation with the unique green tea cultivar Sunrouge prevents age-related cognitive decline in SAMP8 by targeting the amyloid cascade.
Alzheimer’s disease (AD) is a common neurodegenerative disease leading to mild cognitive impairment (MCI) and dementia [Citation1]. The number of elderly with dementia around the world is estimated to rise to 114 million by 2050 [Citation2]. Recent studies have identified an asymptomatic phase, or preclinical AD, that precedes MCI and dementia [Citation3,Citation4]. The risk of progression to AD could be reduced by the implementation of prevention strategies during this phase, which is particularly important as no optimal pharmaceutical or other treatment for AD has been discovered [Citation5].
Recent research indicates that green tea has beneficial effects on cognitive function. According to a Japanese human cohort study, green tea consumption correlates significantly with reduced risks of MCI and incident dementia [Citation6–Citation8]. Moreover, a meta-analysis of the association between the intake of tea and the risk of cognitive disorders indicated a correlation between the consumption of tea and a reduced risk of MCI among the elderly [Citation9]. Catechins, the major polyphenolic constituent of green tea, account for > 30% of the dissolved solids in a normal infusion. The most common catechins are epigallocatechin gallate (EGCG), epigallocatechin, epicatechin gallate and epicatechin [Citation10]. Of these, the majority of potential health benefits of green tea consumption have been attributed to EGCG, which was reported to exert both antioxidative and neuroprotective effects against amyloid-β (Aβ)-induced neurotoxicity in rats [Citation11–Citation14]. The green tea cultivar Sunrouge (Camellia sinensis), which is bred in Japan, is rich in anthocyanins and harbors similar levels of catechins as those of Yabukita (C. sinensis), the most popular Japanese green tea cultivar [Citation15,Citation16]. Sunrouge exhibits distinctive bioactivity and composition patterns relative to other green tea cultivars, and was found to have a stronger suppressive effect than Yabukita on acetylcholinesterase (AChE) activity in the human neuroblastoma cell line SK-N-SH [Citation16–Citation18].
The senescence-accelerated mouse Prone8 (SAMP8) was established as a model for accelerated aging, characterized by the early onset of learning and memory deficits and spontaneous overproduction of soluble Aβ in the brain [Citation19–Citation22]. SAMP8 has since been recognized as a suitable animal model of age-related learning and memory deficits [Citation23]. In this study, we investigated the effects of Sunrouge consumption on cognitive decline in SAMP8 mice.
Materials and methods
Experimental diets
The Sunrouge and Yabukita extracts (SRE and YBE, respectively) were prepared as follows. Leaves from both plants (second crops, 2014) were provided by the Institute of Fruit Tree and Tea Science (NARO, Japan). For each extract, 100 g of dried leaf powder was added to 2,000 ml of boiling water and steeped for 10 min. The extract was filtered through a gauze, and the filtrate was centrifuged at 700 × g for 10 min. The supernatant solution was collected and freeze-dried. The experimental diet included 0.20% (w/w) of SRE or YBE in an AIN-93M diet (Oriental Yeast, Tokyo, Japan).
Analysis of catechins, anthocyanins, flavonols and total polyphenols
Each extract was subjected to a composition analysis as described below. Catechin contents were analyzed by Japan Food Research Laboratories. Anthocyanin and flavonol contents were analyzed using high-performance liquid chromatography (HPLC). Solutions of tea extract powders in ultra-pure water were filtered through a membrane (DISMIC-13HP, PTFE, pore size: 0.45 μm; Advantec, Tokyo, Japan) and injected into the HPLC.
For the anthocyanin analysis, HPLC was performed using a LC-30AD pump (Shimadzu, Kyoto, Japan) coupled to a photo-diode array detector SDP-M20A (520 nm) (Shimadzu, Kyoto, Japan) with a YMC-Pack ODS-A column (6.0 mm i.d. × 150 mm, granule diameter; 5 μm; YMC, Kyoto, Japan). A linear grade system comprising the following eluents at a flow rate of 1 mL/min and 40 ºC was used: mobile phase A (H3PO4:H2O = 1:199) and mobile phase B (CH3CN:mobile phase A = 4:6) [Citation24]. The linear gradient elution was programmed to increase from 20% to 55% mobile phase B over 60 min. Quantification was performed using delphinidin-3-O-galactoside, delphinidin-3-O-glucoside, cyanidin-3-O-galactoside and cyanidin-3-O-glucoside (Tokiwa Phytochemical Co. Ltd., Chiba, Japan) as external standards.
For the flavonol analysis, HPLC was performed using a LC-30AD pump coupled to a photo-diode array detector SDP-M20A (340 nm) with a Unison UK-C18 column (4.6 mm i.d. × 150 mm; granule diameter, 3 μm; Imtakt Corporation, Kyoto, Japan). A linear grade system comprising the following eluents at a flow rate of 1 mL/min and 40 ºC was used: mobile phase A (0.2% formic acid:acetonitrile = 85:15) and mobile phase B (CH3CN:mobile phase A = 4:6). The linear gradient elution was programmed to increase from 8% to 76% mobile phase B over 45 min [Citation25]. Quantification was performed using quercetin-3-O-glucoside (Clearsynth Lab Pvt. Ltd., Mumbai, India), quercetin-3-O-galactoside, myricetin-3-O-galactoside and myricetin-3-O-glucoside (Extrasynthese, Genay, France) as external standards.
Total polyphenols were measured according to the Folin–Ciocalteu Method, using Folin and Ciocalteu’s Phenol Reagent (MP Biomedicals, Eschwege, Germany) [Citation26].
Animal experiments
Male SAMP8 and SAMR1 mice were purchased from Japan SLC Inc. (Shizuoka, Japan). All mice were individually housed and maintained in a temperature- and humidity-controlled room with a 12-h light–dark cycle (light from 8 am to 8 pm). All animal studies were performed in accordance with the law (protocol no. 105) and notification (protocol no. 6) of the Japanese government for the welfare of experimental animals. The study protocol was approved by the Animal Care and Use Committee, Kyushu University (Kyushu University, Scientific Research Promotion Division).
Nineteen-week-old mice were divided into four groups as follows: control diet-fed SAMR1 (SAMR1-Cont), control diet-fed SAMP8 (SAMP8-Cont), SRE diet-fed SAMP8 (SAMP8-SRE) and YBE diet-fed SAMP8 (SAMP8-YBE). Mice were given 5 g of the indicated food per day, with free access to tap water. After 23 weeks, mice were subjected to the novel-object recognition and memory retention test and the Morris water maze test. After the behavioral tests, mice that had fasted overnight were anesthetized under isoflurane vapor for blood collection and subsequently sacrificed. Brains were rapidly excised and stored at −80 ºC. Plasma samples were collected via centrifugation (2,000 × g for 20 min at 4 ºC) of blood samples and stored at −80 ºC.
Novel-object recognition and memory retention test
Long-term memory was evaluated using the novel-object recognition and memory retention test [Citation27]. Each mouse was habituated in a cage for 4 h before training. Training comprised the presentation of two blocks for 5 min, and object recognition was scored by the number of approaches to the object. Eighteen hours later, each mouse was tested for memory retention by replacing one of the original blocks with a new object, and recognition was scored during a 5-min testing period.
Morris water maze test
Spatial learning and memory were investigated using the Morris water maze test [Citation28]. A circular plastic pool (diameter, 120 cm; height, 50 cm) was filled with water maintained at 25 ± 1 ºC. An escape platform (diameter, 11 cm) was hidden in the pool at a fixed location 1 cm below the water surface. During training, mice were given three trials per session on 4 subsequent days in the presence of the platform; each trial had a time limit of 120 s and a trial interval of approximately 20 s. When mice found the platform, they were allowed to stay on it for 20 s. Mice that did not find the platform within 120 s were placed on it for 20 s at the end of trial. One day after the final training session, mice were subjected to a probe trial session in which the platform was removed from the pool. The mice were allowed to swim for 120 s to search for it. The escape latency time and number of crossings over the platform area were recorded and analyzed using an overhead camera and ANY-maze video tracking system software (Stoelting Co., Wood Dale, IL, USA).
Measurement of amyloid-β 1–42
Mouse brains were homogenized in a buffer containing 50 mM Tris-HCl (pH 7.5), 1 mM EDTA, 50 mM NaF, 30 mM Na4P2O7 and 1% Triton-X100 (v/v). The amounts of total protein and amyloid-β 1–42 (Aβ42) in the supernatants of brain homogenates were measured using a Bicinchoninic Acid Protein Assay Reagent (Rockford, USA) and Human/RAT β-Amyloid (42) Enzyme-linked Immunosorbent Assay Kit, High-Sensitive (Wako, Kyoto, Japan), respectively, according to the manufacturer’s instructions.
Measurement of AChE activity
AChE activity in the supernatants of brain homogenate was measured using the Amplite Colorimetric Acetylcholinesterase Assay Kit (AAT Bioquest Inc., Sunnyvale, CA, USA) according to the manufacturer’s instructions.
Biochemical analysis of plasma
Plasma levels of aspartate aminotransferase (AST) and alanine aminotransferase (ALT) were measured using transaminase C2-Test Kits (Wako, Kyoto, Japan) according to the manufacturer’s instructions.
RNA isolation and real-time PCR quantification
Total RNA was isolated from the brain of each individual animal using IsogenII Reagent (Nippongene, Toyama, Japan) and purified using SV Total RNA Isolation System (Promega, Madison, WI, USA). cDNA was synthesized from total RNA using the PrimeScript RT Reagent Kit (Takara Bio, Tokyo, Japan). Gene expression was analyzed using quantitative real-time PCR using SYBR green, a Thermal Cycler DiceH Real-Time System (Bio-Rad, Hercules, CA, USA) and the following primer sequences: BACE1, forward: 5´-agggcttgcacctgtaggac-3´ and reverse: 5´-gcctgagtatgacgccagta-3´; neprilysin, forward: 5´-ccaaacttaagcctattcttac-3´ and reverse: 5´-ccattatgaacctccaggac-3´. Beta-actin was selected as an internal control with which to normalize the quantitative real-time PCR results (beta-actin forward: 5´-catccgtaaagacctctatgccaac-3´ and reverse: 5´-atggagccaccgatccaca-3´).
Statistical analysis
Data are presented as means ± standard errors of the means. Morris water maze test training session data were analyzed via two-way ANOVA; all other data were analyzed using Tukey’s test. Prism software (GraphPad, Inc., San Diego, CA, USA) was used for the analysis. A value of p < 0.05 was considered significant.
Results
General observations
The compositions of SRE and YBE are listed in . Although both extracts contained equivalent amounts of EGCG, SRE had lower concentrations of catechins and total polyphenols but higher concentrations of the flavonols: quercetin-3-O-glucoside, quercetin-3-O-galactoside and myricetin-3-O-galactoside. In addition, SRE, but not YBE, contained anthocyanins such as cyanidin-3-O-glucoside, cyanidin-3-O-galactoside, delphinidin-3-O-glucoside and delphinidin-3-O-galactoside. The compositions of the experimental diets that contained 0.2% (w/w) SRE or YBE are listed in . Although the final body weight of SAMR1-Cont was significantly higher than that of SAMP8-Cont, SAMP8-SRE and SAMP8-YBE, no significant difference was observed among those of SAMP8-Cont, SAMP8-SRE and SAMP8-YBE. These results indicate that SRE or YBE feeding did not affect the final body weight. Similarly, SRE or YBE feeding did not affect the serum AST and ALT levels ().
Table 1. Composition of tea extract powders.
Table 2. Composition of the experimental diets.
Table 3. Effects of Sunrouge and Yabukita extracts on weight gain and liver function.
Effect of SRE feeding on cognitive function
To evaluate the effect of SRE feeding on long-term memory decline, we subjected mice to a novel object recognition and memory retention test after the 23-week experimental diet period. During training, mice were presented with two objects, A and B, for 5 min (), and all mice touched each object approximately the same number of times (). At 18 h after training, one of the original objects was replaced by a new object, C. Notably, SAMR1-Cont and SAMP8-SRE mice touched the new object significantly more times than did SAMP8-Cont mice. However, no significant difference was observed between SAMP8-Cont and SAMP8-YBE mice (). Because the moving distance of each group of mice showed no significant difference, it was considered that exercise capacity did not affect these results (Figure S1).
Figure 1. Effect of SRE consumption on long-term memory in SAMP8. Control diet-fed SAMR1 (SAMR1-Cont), control diet-fed SAMP8 (SAMP8-Cont), SRE diet-fed SAMP8 (SAMP8-SRE) and YBE diet-fed SAMP8 (SAMP8-YBE) mice were subjected to the novel object recognition and memory retention test after a 23-week dietary experimental period to evaluate long-term memory. (a) During training, two objects, A and B, were presented to a mouse. During testing, one object was replaced by a novel object, C. Each mouse was tested 18 h after training. If the mouse remembered object A, it touched the novel object C more often. (b) Object preference was scored by the number of approaches to the object {B/(A + B)}. All groups of mice had equal preferences for objects A and B. (c) Object preference was scored by the number of approaches to the object {C/(A + C)}. Data are expressed as means ± standard errors of the means; n = 6; **p < 0.01; n.s.: not significant.
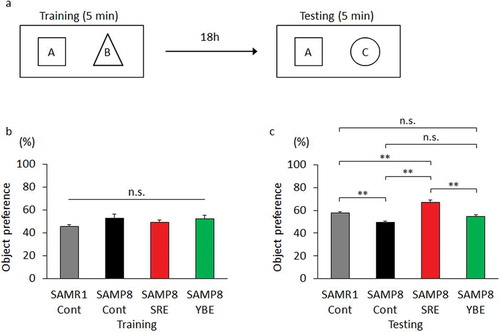
Next, we subjected mice to the Morris water maze test at the end of the experimental diet period to evaluate the effects of SRE on spatial learning and memory decline. In the training trial, SAMR1-Cont mice had a significantly shorter escape latency relative to SAMP8-Cont mice on days 3 and 4, whereas SAMP8-SRE mice had a significantly shorter escape latency relative to SAMP8-Cont mice on days 2 and 3. No significant differences were observed between SAMP8-Cont and SAMP8-YBE mice (). In a probe trial, SAMR1-Cont and SAMP8-SRE mice made a significantly increased number of platform crossings, compared with SAMP8-Cont mice, whereas the latter did not differ significantly from SAMP8-YBE mice (). Although the moving distance of SAMR1-Cont in probe test was significantly larger than that of SAMP8-Cont and SAMP8-YBE, no significant difference was observed among those of SAMP8-Cont, SAMP8-SRE and SAMP8-YBE (Figure S2). It was considered that exercise capacity did not affect the results among SAMP8-Cont, SAMP8-SRE and SAMP8-YBE. These results indicate that long-term SRE consumption could prevent cognitive decline in SAMP8 mice.
Figure 2. Effect of SRE consumption on spatial learning and memory in SAMP8. Control diet-fed SAMR1 (SAMR1-Cont), control diet-fed SAMP8 (SAMP8-Cont), SRE diet-fed SAMP8 (SAMP8-SRE) and YBE diet-fed SAMP8 (SAMP8-YBE) mice were subjected to the Morris water maze test after a 23-week dietary experimental period to assess spatial learning and memory. (a) Escape latency was used as a measure of spatial learning in the training trial. (b) The number of crossings over the area where a platform had previously been placed was used as a measure of spatial learning and memory in the probe trial. Data are expressed as means ± standard errors of the means; n = 6; **p < 0.01; n.s.: not significant.
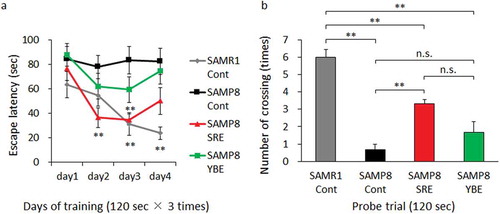
Effect of SRE feeding on Aβ42 generation
Currently, the amyloid cascade is hypothesized to be the cause of AD [Citation29]. Accordingly, we investigated whether SRE feeding could affect this cascade. SAMR1-Cont and SAMP8-SRE mice had significantly lower brain concentrations of Aβ42, compared with SAMP8-Cont mice ().
Figure 3. Aβ42 content and BACE1 and Mme expression in the brain. (a) Aβ42 content in brains collected from control diet-fed SAMR1 (SAMR1-Cont), control diet-fed SAMP8 (SAMP8-Cont) and SRE diet-fed SAMP8 (SAMP8-SRE) mice after a 23-week dietary experimental period. (b) BACE1 expression levels in brains collected from the groups in A. (c) Mme (encodes neprilysin) expression levels in the brains collected from the groups in A. Data are expressed as means ± standard errors of the means; n = 6; *p < 0.05; **p < 0.01; n.s.: not significant.
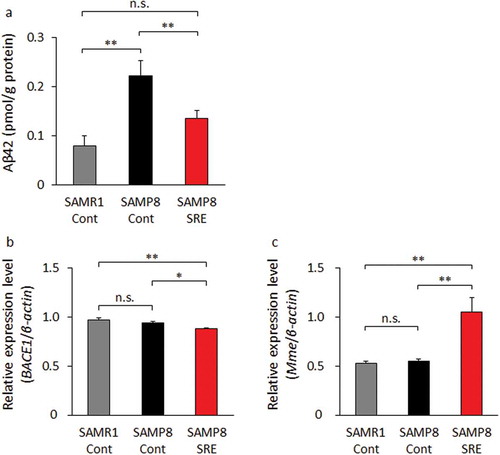
We further investigated the brain expression levels of the Aβ-related genes encoding BACE1, an amyloid precursor protein-cleaving enzyme that generates Aβ42, and neprilysin, an endopeptidase that degrades Aβ42 [Citation30,Citation31]. Notably, the expression of BACE1 was significantly lower, whereas that of Mme (neprilysin) was significantly higher in SAMP8-SRE mice relative to those of SAMR1-Cont and SAMP8-Cont mice (). These results indicate that SRE consumption could prevent increases in Aβ42 levels and improve the expression patterns of Aβ-related genes in the brain.
Effect of SRE feeding on AChE activity
Cholinergic abnormalities have been observed in AD brains, and acetylcholine plays a principal role in cholinergic signaling [Citation32–Citation34]. As AChE is responsible for the degradation of acetylcholine to acetate and choline in the synaptic cleft, and we analyzed the AChE activity in the brains of model mice. Notably, significantly lower AChE activity levels were observed in the brains of SAMP8-SRE and SAMR1-Cont mice relative to SAMP8-Cont mice ().
Figure 4. AChE activity in the brain. AChE activity was evaluated in the brains of control diet-fed SAMR1 (SAMR1-Cont), control diet-fed SAMP8 (SAMP8-Cont) and SRE diet-fed SAMP8 (SAMP8-SRE) mice after a 23-week dietary experimental period. Data are expressed as means ± standard errors of the means; n = 6; **p < 0.01; n.s.: not significant.
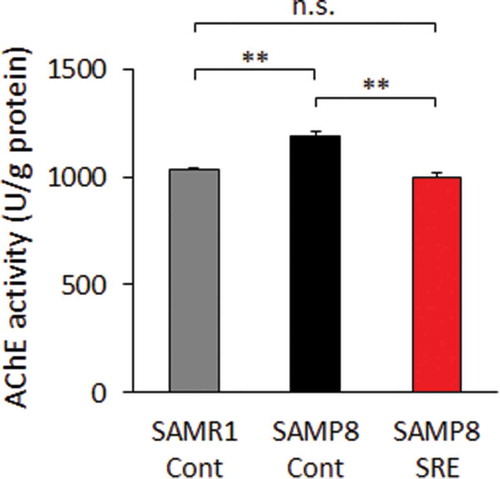
Discussion
We have demonstrated for the first time that Sunrouge extract feeding can prevent age-related cognitive decline more effectively than can Yabukita in mice. A previous cohort study of Japanese found that consumption of green tea was associated with a reduced risk of MCI and incident dementia [Citation6–Citation9]. Considering that Yabukita comprises 76% of the tea growing area in Japan, our results suggest that the consumption of Sunrouge tea may reduce the risk for MCI and incident dementia more effectively. While the two extracts tested in this study contained similar amounts of EGCG, in comparison with YBE, SRE contained higher levels of the flavonols quercetin-3-O-glucoside, quercetin-3-O-galactoside and myricetin-3-O-galactoside as well as the anthocyanins cyanidin-3-O-glucoside, cyanidin-3-O-galactoside, delphinidin-3-O-glucoside and delphinidin-3-O-galactoside, which were not detected in YBE. EGCG has been reported to have neuroprotective effects against amyloid-β (Aβ)-induced neurotoxicity, and a previous study showed that green tea catechin consumption at a mean daily EGCG dose of approximately 53 mg/kg body weight (bw) prevents cognitive impairment in SAMP8 [Citation35]. To investigate the influence of characteristic components of Sunrouge, we administered 0.2% of SRE or YBE, a mean daily EGCG dose of approximately 33 mg/kg bw, to SAMP8 for 23 weeks.
To investigate the effects of long-term SRE consumption on cognitive decline, we performed behavioral tests after a 23-week experimental period. Notably, SRE appeared to effectively prevent cognitive decline, whereas YBE had little impact. A previous study of green tea catechins found that a mean daily EGCG dose of approximately 53 mg/kg bw prevented cognitive decline in SAMP8 [Citation35]. In contrast, SAMP8-SRE and SAMP8-YBE mice in our study received approximate mean EGCG doses of 33 mg/kg bw/day. Therefore, it was considered that the inefficacy of YBE in our study was caused by the lower dose of EGCG. In contrast, the characteristic components of Sunrouge were thought to have prevented cognitive decline because the doses of EGCG in SRE and YBE were equivalent.
Quercetin has been reported to have anti-inflammatory, anti-ischemic, and anti-oxidative effects, and quercetin treatment (50 mg/kg bw/day) was found to improve chronic stress-induced cognitive dysfunction in rats [Citation36–Citation38]. In addition, myricetin was reported to inhibit the formation of AD-related Aβ fibrils and to improve chronic stress-induced cognitive dysfunction in mice when administered at a dosage of 50 mg/kg bw/day [Citation39–Citation41]. Anthocyanins have also been reported to have neuroprotective properties, and mulberry extract treatment (15 mg/kg bw/day cyanidin-3-O-glucoside) was found to improve learning and memory abilities in mice [Citation42,Citation43]. In our study, SAMP8-SRE mice received mean quercetin aglycone, myricetin aglycone, and cyanidin-3-O-glucoside doses of approximately 1.9, 4.2 and 0.034 mg/kg bw/day, respectively. Although these doses were lower than those reported in previous studies, these components and EGCG might have a synergistic or additive preventive effect against cognitive decline [Citation38,Citation41,Citation43]. In addition, this effect might be mediated by unknown Sunrouge components.
An imbalance between Aβ production and removal leads to Aβ accumulation. A previous study demonstrated early-onset learning and memory deficits along with spontaneous soluble Aβ overproduction in SAMP8 [Citation20–Citation22]. BACE1 and neprilysin are prime therapeutic targets for preventing AD by suppressing accumulation of Aβ42. Our results demonstrated that SRE consumption prevented an age-related increase in Aβ42 levels in the SAMP8 brain. In addition, SRE decreased the expression of BACE1 and increased the expression of Mme, suggesting that SRE feeding affects the expression of Aβ-related genes and may inhibit the accumulation of Aβ42. It has been reported that the administration of epicatechin reduced the Aβ level in TASTPM mice, a transgenic mouse model of Alzheimer’s disease, and epicatechin inhibited BACE1 expression in vitro [Citation44]. In addition, the administration of EGCG prevented Aβ42 accumulation and increased neprilysin expression in SAMP8 [Citation45]. Epicatechin and EGCG might affect the expression of BACE1 and Mme in our study. However, Epicatechin and EGCG did not contribute to the superiority of Sunrouge, because the Yabukita extract contained more abundant epicatechin than did Sunrouge. Our data suggest that the characteristic components of Sunrouge were effective in preventing age-related cognitive decline. Further experimentation on the preventive effects of cognitive decline by each component of Sunrouge should be initiated in the future.
AChE inhibition is another therapeutic target to prevent AD. The observation that SRE consumption suppressed the increase in AChE activity in aged SAMP8-SRE mice suggests the potential for this extract to alleviate cholinergic system dysfunction. It has been reported that the administration of green tea polyphenols inhibited AChE activity in mice brain [Citation46]. In addition, the anthocyanin-rich fraction of blueberries had high inhibitory effect on AChE in mice [Citation47]. Sunrouge extract contains cyanidin-3-O-glucoside, cyanidin-3-O-galactoside, delphinidin-3-O-glucoside and delphinidin-3-O-galactoside. Sunrouge anthocyanins might affect AChE activity in the SAMP8-SRE brain.
In conclusion, Sunrouge appears to successfully prevent age-related cognitive decline by targeting the amyloid cascade and cholinergic system. Daily consumption of this green tea cultivar may help to prevent dementia.
Author contributions
M.W., H.N., R.K. and M.M. performed experiments. M.W., H.N., R.K., Y.S. and M.M. were involved in data analysis and interpretation. M.W. and H.T. designed and supervised the project. M.W., H.N., R.K. and H.T. wrote the paper. All authors reviewed the manuscript.
Supplementary_data.pdf
Download PDF (23.3 KB)Acknowledgments
We are grateful to Dr. Atsushi Nesumi (Institute of Fruit Tree and Tea Science, NARO) for kindly donating the Sunrouge and Yabukita tea leaves. This work was supported in part by JSPS KAKENHI Grants 15H02448 (to H.T.).
Disclosure statement
No potential conflict of interest was reported by the authors.
Supplementary material
Supplementary data for this article can be accessed here.
Additional information
Funding
References
- Hardy J, Selkoe DJ. The amyloid hypothesis of Alzheimer’s disease: progress and problems on the road to therapeutics. Science. 2002;297(5580):353–356.
- Wimo A, Winblad B, Aguero-Torres H, et al The magnitude of dementia occurrence in the world. Alzheimer Dis Assoc Disord. 2003;17(2):63–67.
- Rowe CC, Ellis KA, Rimajova M, et al Amyloid imaging results from the Australian Imaging, Biomarkers and Lifestyle (AIBL) study of aging. Neurobiol Aging. 2010;31(8):1275–1283.
- Sperling RA, Aisen PS, Beckett LA, et al Toward defining the preclinical stages of Alzheimer’s disease: recommendations from the National Institute on Aging-Alzheimer’s Association workgroups on diagnostic guidelines for Alzheimer’s disease. Alzheimers Dement. 2011;7(3):280–292.
- Riedel WJ. Preventing cognitive decline in preclinical Alzheimer’s disease. Curr Opin Pharmacol. 2014;14:18–22.
- Kuriyama S, Hozawa A, Ohmori K, et al Green tea consumption and cognitive function: a cross-sectional study from the Tsurugaya project 1. Am J Clin Nutr. 2006;83(2):355–361.
- Noguchi-Shinohara M, Yuki S, Dohmoto C, et al Consumption of green tea, but not black tea or coffee, is associated with reduced risk of cognitive decline. PLoS One. 2014;9(5):e96013.
- Tomata Y, Sugiyama K, Kaiho Y, et al Green tea consumption and the risk of incident dementia in elderly Japanese: the ohsaki cohort 2006 study. Am J Geriatr Psychiatry. 2016;24(10):881–889.
- Ma QP, Huang C, Cui QY, et al Meta-analysis of the association between tea intake and the risk of cognitive disorders. PLoS One. 2016;11(11):e0165861.
- Harbowy EM, Balentine AD. Tea chemistry. Crit Rev Plant Sci. 1997;16:415–480.
- Nagle DG, Ferreira D, Zhou YD. Epigallocatechin-3-gallate (EGCG): chemical and biomedical perspectives. Phytochemistry. 2006;67(17):1849–1855.
- Biasibetti R, Tramontina AC, Costa AP, et al Green tea (-)epigallocatechin-3-gallate reverses oxidative stress and reduces acetylcholinesterase activity in a streptozotocin-induced model of dementia. Behav Brain Res. 2013;236(1):186–193.
- Rezai-Zadeh K, Shytle D, Sun N, et al Green tea epigallocatechin-3-gallate (EGCG) modulates amyloid precursor protein cleavage and reduces cerebral amyloidosis in Alzheimer transgenic mice. J Neurosci. 2005;25(38):8807–8814.
- Rezai-Zadeh K, Arendash GW, Hou H, et al Green tea epigallocatechin-3-gallate (EGCG) reduces beta-amyloid mediated cognitive impairment and modulates tau pathology in Alzheimer transgenic mice. Brain Res. 2008;1214:177–1787.
- Saito T, Honma D, Tagashira M, et al Anthocyanins from new red leaf tea ‘Sunrouge’. J Agric Food Chem. 2011;59(9):4779–4782.
- Maeda-Yamamoto M, Saito T, Nesumi A, et al Chemical analysis and acetylcholinesterase inhibitory effect of anthocyanin-rich red leaf tea (Cv. Sunrouge). J Sci Food Agric. 2012;92(11):2379–2386.
- Fujimura Y, Kurihara K, Ida M, et al Metabolomics-driven nutraceutical evaluation of diverse green tea cultivars. PLoS One. 2011;6(8):e23426.
- Kumazoe M, Fujimura Y, Hidaka S, et al Metabolic profiling-based data-mining for an effective chemical combination to induce apoptosis of cancer cells. Sci Rep. 2015;5:9474.
- Takeda T. Senescence-accelerated mouse (SAM): a biogerontological resource in aging research. Neurobiol Aging. 1999;20(2):105–110.
- Chen GH, Wang YJ, Qin S, et al Age-related spatial cognitive impairment is correlated with increase of synaptotagmin 1 in dorsal hippocampus in SAMP8 mice. Neurobiol Aging. 2007;28(4):611–618.
- Petursdottir AL, Farr SA, Morley JE, et al Lipid peroxidation in brain during aging in the senescence-accelerated mouse (SAM). Neurobiol Aging. 2007;28(8):1170–1178.
- Gong Y, Liu L, Xie B, et al Ameliorative effects of lotus seedpod proanthocyanidins on cognitive deficits and oxidative damage in senescence-accelerated mice. Behav Brain Res. 2008;194(1):100–107.
- Butterfield DA, Poon HF. The senescence-accelerated prone mouse (SAMP8): a model of age-related cognitive decline with relevance to alterations of the gene expression and protein abnormalities in Alzheimer’s disease. Exp Gerontol. 2005;40(10):774–783.
- Terahara N, Takeda Y, Nesumi A, et al Anthocyanins from red flower tea (Benibana-cha), Camellia sinensis. Phytochemistry. 2001;56(4):359–361.
- Monobe M, Nomura S, Ema K, et al Quercetin glycosides-rich tea cultivars (Camellia sinensis L.) in Japan. Food Sci Technol Res. 2015;21(3):333–340.
- Wan C, Yu Y, Zhou S, et al Antioxidant activity and free radical-scavenging capacity of Gynura divaricata leaf extracts at different temperatures. Pharmacogn Mag. 2011;7(25):40–45.
- Wang H, Ferguson GD, Pineda VV, et al Overexpression of type-1 adenylyl cyclase in mouse forebrain enhances recognition memory and LTP. Nat Neurosci. 2004;7(6):635–642.
- Morris R. Developments of a water-maze procedure for studying spatial learning in the rat. J Neurosci Methods. 1984;11(1):47–60.
- Hardy JA, Higgins GA. Alzheimer’s disease: the amyloid cascade hypothesis. Science. 1992;256(5054):184–185.
- Stockley JH, O’Neill C. Understanding BACE1: essential protease for amyloid-beta production in Alzheimer’s disease. Cell Mol Life Sci. 2008;65(20):3265–3289.
- Iwata N, Tsubuki S, Takaki Y, et al Metabolic regulation of brain Abeta by neprilysin. Science. 2001;292(5521):1550–1552.
- Shimohama S, Taniguchi T, Fujiwara M, et al Changes in nicotinic and muscarinic cholinergic receptors in Alzheimer-type dementia. J Neurochem. 1986;46(1):288–293.
- Whitehouse PJ, Martino AM, Antuono PG, et al Nicotinic acetylcholine binding sites in Alzheimer’s disease. Brain Res. 1986;371(1):146–151.
- Mohapel P, Leanza G, Kokaia M, et al Forebrain acetylcholine regulates adult hippocampal neurogenesis and learning. Neurobiol Aging. 2005;26(6):939–946.
- Li Q, Zhao HF, Zhang ZF, et al Long-term green tea catechin administration prevents spatial learning and memory impairment in senescence-accelerated mouse prone-8 mice by decreasing Abeta1-42 oligomers and upregulating synaptic plasticity-related proteins in the hippocampus. Neuroscience. 2009;163(3):741–749.
- Youdim KA, Qaiser MZ, Begley DJ, et al Flavonoid permeability across an in situ model of the blood-brain barrier. Free Radic Biol Med. 2004;36(5):592–604.
- Rogerio AP, Kanashiro A, Fontanari C, et al Anti-inflammatory activity of quercetin and isoquercitrin in experimental murine allergic asthma. Inflamm Res. 2007;56(10):402–408.
- Mohammadi HS, Goudarzi I, Lashkarbolouki T, et al Chronic administration of quercetin prevent spatial learning and memory deficits provoked by chronic stress in rats. Behav Brain Res. 2014;270:196–205.
- Hirohata M, Hasegawa K, Tsutsumi-Yasuhara S, et al The anti-amyloidogenic effect is exerted against Alzheimer’s beta-amyloid fibrils in vitro by preferential and reversible binding of flavonoids to the amyloid fibril structure. Biochemistry. 2007;46(7):1888–1899.
- DeToma AS, Choi JS, Braymer JJ, et al Myricetin: a naturally occurring regulator of metal-induced amyloid-β aggregation and neurotoxicity. Chembiochem. 2011;12(8):1198–1201.
- Wang QM, Wang GL, Ma ZG. Protective effects of myricetin on chronic stress-induced cognitive deficits. Neuroreport. 2016;27(9):652–658.
- Del Rio D, Borges G, Crozier A. Berry flavonoids and phenolics: bioavailability and evidence of protective effects. Br J Nutr. 2010;104(Suppl 3):S67–90.
- Shih PH, Chan YC, Liao JW, et al Antioxidant and cognitive promotion effects of anthocyanin-rich mulberry (Morus atropurpurea L.) on senescence-accelerated mice and prevention of Alzheimer’s disease. J Nutr Biochem. 2010;21(7):598–605.
- Cox CJ, Choudhry F, Peacey E, et al Dietary (-)-epicatechin as a potent inhibitor of βγ-secretase amyloid precursor protein processing. Neurobiol Aging. 2015;36(1):178–187.
- Chang X, Rong C, Chen Y, et al (-)-Epigallocatechin-3-gallate attenuates cognitive deterioration in Alzheimer’s disease model mice by upregulating neprilysin expression. Exp Cell Res. 2015;334(1):136–145.
- Kim HK, Kim M, Kim S, et al Effects of green tea polyphenol on cognitive and acetylcholinesterase activities. Biosci Biotechnol Biochem. 2004;68(9):1977–1979.
- Papandreou MA, Dimakopoulou A, Linardaki ZI, et al Effect of a polyphenol-rich wild blueberry extract on cognitive performance of mice, brain antioxidant markers and acetylcholinesterase activity. Behav Brain Res. 2009;198(2):352–358.