ABSTRACT
Cucurbitaceae plants contain characteristic triterpenoids. Momordica charantia, known as a bitter melon, contains cucurbitacins and multiflorane type triterpenes, which confer bitter tasting and exhibit pharmacological activities. Their carbon skeletons are biosynthesized from 2,3-oxidosqualene by responsible oxidosqualene cyclase (OSC). In order to identify OSCs in M. charantia, RNA-seq analysis was carried out from ten different tissues. The functional analysis of the resulting four OSC genes revealed that they were cucurbitadienol synthase (McCBS), isomultiflorenol synthase (McIMS), β-amyrin synthase (McBAS) and cycloartenol synthase (McCAS), respectively. Their distinct expression patterns based on RPKM values and quantitative RT-PCR suggested how the characteristic triterpenoids were biosynthesized in each tissue. Although cucurbitacins were finally accumulated in fruits, McCBS showed highest expression in leaves indicating that the early step of cucurbitacins biosynthesis takes place in leaves, but not in fruits.
Abbreviations: OSC: oxidosqualene cyclase; RPKM: reads perkilobase of exon per million mapped reads
Graphical Abstract
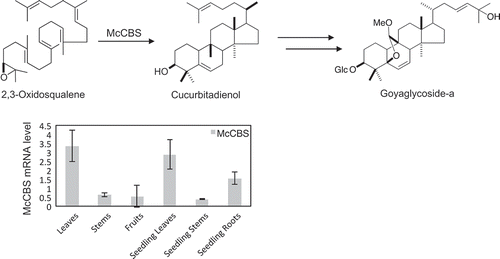
Four oxidosqualene cyclase genes were identified from M. charantia including cucurbitadienol synthase responsible for cucurbitacins biosynthesis.
Secondary metabolites produced by various plants and microorganisms have contributed enormously to improve our quality of life. Such compounds exert specific and potent bioactivities due to their unique and complex structures. Therefore, a large number of drug discoveries have been carried out to utilize natural products as a lead compound. However, in many cases, the amount of natural products obtained from natural sources is not sufficient for drug development. Biosynthetic studies may serve to overcome such difficulty. Identification and overexpression of key biosynthetic genes enables production of desired natural products in a heterologous host. Structural diversity of natural products originates in mainly two steps; a formation of various core carbon skeletons exemplified by terpenoid and polyketide, and a modification of the core structure by various oxygenation and glycosylation reactions. Terpenoid represents one of the largest classes of natural products among plants. Their diversity is formed by terpene synthases, a repertoire of enzymes each catalyzing cyclization of an acyclic precursor in a similar but slightly different manners to produce a variety of structures. Among them, triterpenes are major terpenoids in plants that possess important bioactivities, such as antiviral and anti-inflammatory activities and are often the active constituents of famous medicinal plants [Citation1]. The first step of their biosynthesis is a cyclization of 2,3-oxidosqualene catalyzed by oxidosqualene cyclases (OSCs), which convert the common precursor into more than 100 different carbon skeletons. Several OSC genes exist in a single plant that are responsible for the formation of characteristic triterpenes found in such a plant. Thus, a type of OSCs coded in the genome and the reaction they catalyze dictates the triterpene constituents. Previously, over 80 different OSC genes have been identified from many plants [Citation2]. Furthermore, modification steps mainly involve cytochrome P450 monooxygenases (P450s) that hydroxylates and oxidize various carbons of the core structure [Citation3]. Cyclization of oxidosqualene takes place in two different conformations. One is a chair-chair-chair cyclization of the A/B/C rings that produces majority of triterpenes found in plants such as β-amyrin. The other is a chair-boat-chair cyclization. Both cycloartenol and cucurbitadienol, precursors for plant sterols and cucurbitacins, respectively, are produced in this manner.
Cucurbitaceae is an economically important family including many vegetables and fruits that we consume. Characteristic triterpenoids cucurbitacins are major constituents in this family and confers a bitter taste [Citation4]. A genome analysis of Cucumis sativus has been completed and identified several genes involved in cucurbitacin biosynthesis [Citation5–Citation7]. Cucurbitacins are highly oxygenated triterpenes having a cucurbitane skeleton and various P450s are expected to be involved in their biosynthesis. Momordica charantia, which also belongs to Cucurbitaceae, is known as a bitter melon and consumed as a vegetable mainly in East and South East Asian countries. Due to its strong bitter taste, M. charantia contains many cucurbitacins such as goyaglycosides and momordicosides () [Citation8]. An antidiabetic activity was found in these cucurbitacins from M. charantia [Citation9]. Besides cucurbitacins, multiflorane type triterpene was also found characteristic of Cucurbitaceae such as bryonolic acid from sponge gourd (Luffa cylindrica) [Citation10]. M. charantia also contains several multiflorane types, together with a regular oleanane type, that are found throughout the plant kingdom [Citation8,Citation11]. Therefore, M. charantia would provide several OSC genes involved in triterpene biosynthesis, together with numerous P450 genes responsible for skeletal modifications. In this report, we identified and characterized four OSC genes related to triterpenoids biosynthesis in M. charantia and studied their expression patterns in different tissues.
Materials and methods
Plant materials
Seeds of Momordica charantia (Erabu, cv.) were sown on the mixtures (1:1) of soil and vermiculite and grown for 2–3 weeks in a greenhouse (Kazusa DNA Research Institute) from year 2012 to 2015. The leaves, stems, roots were collected from three independent plants and stored in liquid nitrogen. Plants of M. charantia were cultivated in a vegetable field (Tateyama, Chiba) of Southern Prefectural Horticulture Institute in Chiba Prefectural Agriculture and Forestry Research Center from year 2012 to 2015. The old leaves, young leaves, stems, tendrils, male flowers, female flowers and fruits were collected from three independent plants and stored in liquid nitrogen. Ten different types of tissues were harvested from M. charantia, which were old leaves, young leaves, stems, tendrils, male flowers, female flowers, fruits, seedling leaves, seedling stems and seedling roots for both RNA-seq and quantitative RT-PCR analyses.
Isolation of RNA and RNA-seq using next-generation sequencing
Total RNA from ten different types of tissues from M. charantia, which were old leaves, young leaves, stems, tendrils, male flowers, female flowers, fruits, seedling leaves, seedling stems and seedling roots, were prepared according to the previous protocol [Citation12]. Briefly, each tissue was frozen in liquid nitrogen, ground in a mortar and suspended in HP Buffer containing 100 mM Tris-HCl (pH 9.0–9.5), 10 mM EDTA-2Na (pH 8.0), 2% SDS, 73 mM LiCl, 530 mM NaCl and 400 mM sodium citrate. After centrifugation at 12,000 g, the supernatant was resuspended in CIA (chloroform: isoamylalcohol = 24: 1) and the total RNA was further collected by isopropanol precipitation. The cDNA library was constructed with the creation of blunt-end fragments and the indexed adaptor ligation according to the RNA-seq protocol (Illumina, CA, USA). The triplicated samples of the ten tissues were hybridized to flow cells and cluster amplification was completed using cBot Cluster Generation System (Illumina), and then sequenced by Illumina’s next-generation sequencing instruments Genome Analyzer IIx (at 2012), HiSeq 1000 (at 2012), and HiSeq 1500 Rapid-Run Mode (at 2013) with 100 bp paired end (PE) reads. The RNA-seq data was utilized for discovery of genes involved in triterpene biosynthesis in the basically same manner with the previous report [Citation13]. The obtained Illumina reads were assembled using commercially available CLC Genomics Workbench version 5.5.2 (CLC bio Japan, Tokyo, Japan) using the following parameters: minimum contig length, 800; with perform scaffolding. The assembled contigs were also used as queries against the non-redundant (nr) protein database using the BLASTX program (e-5). The RPKM values were calculated using CLC Genomics Workbench as described previously [Citation13].
Cloning of candidate OSC cDNAs from M. charantia
To isolate OSC genes, first strand cDNAs were synthesized from total RNA prepared from tissues where each OSC genes were found most expressed using an oligo dT primer RACE32 and ReverTra Ace (TOYOBO) according to the manufacturer’s instructions. PCR were carried out with each primer pairs (Table S1) and the first strand cDNAs as a template using PrimeSTAR HS DNA Polymerase (TaKaRa) with a program (98℃, 1 min, 57℃, 2 min, 72℃, 3 min) for 30 cycles. The each amplified 2.3 kb full length OSC fragments were subcloned into pESC-Ura (Agilent Technologies) using each appropriate restriction enzyme sites (McBAS; Sal I and Kpn I, McCAS; Sal I and Xho I, McCBS; BamH I and Sal I, McIMS; BamH I and Xho I) and sequenced. Accession numbers of the four OSC genes are as follows; McCBS (AB781675), McBAS (AB781676), McCAS (AB781677), McIMS (AB781678).
Functional expression of OSC genes in yeast
Each plasmid harboring full length OSC gene, pESC-Ura-McBAS, pESC-Ura-McCAS, pESC-Ura-McCBS, pESC-Ura-McIMS, pYES2-PSX and pYES2-CPQ, were introduced into S. cerevisiae strain GIL77 using Frozen EZ Yeast transformation II kit (Zymo Research). The culture of transformant yeast, induction of genes and product extraction were carried out as described previously [Citation14].
A Gas Chromatograph Mass Spectrometer (GC-MS) analysis was performed using QP2010SE (Shimadzu) with Rtx-5MS column (250 μm i.d. x 30 m, 0.25 μm film thickness, Restek). The column temperature was raised from 250℃ to 320℃ at a rate of 4℃/min and maintained at 320℃ for 12.5 min with a helium gas carrier at 45 cm/sec. The injection temperature was 250℃, electron ionization was performed with 70 eV, ion source temperature was 250℃ and mass scan range was from m/z 50–450 with a 0.5 sec collection interval. All samples were dissolved in acetone and 1 μl injected with a splitless mode.
For NMR analysis, hexane extract from 4 L culture of GIL77 transformed with pESC-Ura-McIMS was purified by silica gel column chromatography with benzene as an eluent. The desired fractions were collected and NMR spectra were measured on a JEOL JNM-AL300 spectrometer (1H at 300 MHz, 13C at 75 MHz) with CDCl3 (99.8% atom 2H, Kanto Chemical) as a solvent with a solvent signal δ 7.26 ppm for 1H and δ 77.0 ppm for 13C as a reference for chemical shifts.
Isomultiflorenol: 1H NMR (300 MHz, CDCl3) δ 0.791 (3H, s), 0.945 (6H, s), 0.958 (3H, s), 0.977 (3H, s), 0.992 (3H, s), 1.045 (3H, s), 1.060 (3H, s), 3.225 (1H, dd, J = 11.7, 4.8 Hz) (see Figure S1).
13C NMR (75 MHz, CDCl3) δ 15.590, 18.960, 19.182, 19.774, 20.769, 24.583, 26.153, 27.452, 27.847, 28.011, 28.274, 30.617, 30.741, 31.472, 32.911, 34.152, 34.292, 34.580, 34.975, 36.668, 36.693, 37.194, 37.539, 38.748, 40.943, 44.059, 50.619, 78.948, 133.445, 134.982 (see Figure S1).
Quantification of transcript level of OSCs
Total RNAs were each extracted from leaves, stems, fruits, seedling leaves, seedling stems and seedling roots of M. charantia, and cDNAs were synthesized using the PrimeScript RT reagent Kit (TaKaRa Bio Inc.). Expression of target genes was analyzed using SYBR Green I Dye (Sigma). Primers for each gene were shown in Table S1. McActin was utilized as a reference.
Metabolite analysis by liquid chromatography-mass spectrometry (LC-MS)
The lyophilized tissues from M. charantia, which were fruits, leaves, stems and roots, were ground using TissueLyser (Qiagen) and 10 mg of them were suspended in methanol. After centrifugation at 15,000 rpm for 10 min, the supernatants were purified with a Sep-Pak C18 column (Waters) and eluted with methanol. The extracts, dried and resuspended in 80% methanol, were injected into a LC-LTQ-FT-ICR-MS (LC, Agilent 1100 series (Agilent); LTQ-FT-ICR-MS (Thermo Fisher Scientific)). The metabolites were separated using a C18 column (Ascentis Express; 4.6 × 250 mm, 3 µm; Sigma-Aldrich) with a flow rate of 500 μL min−1 and the following gradient: water + 0.1% formic acid/acetonitrile + 0.1% formic acid, 85/15 to 5/95% (0.0 to 40.0 min), 5/95% (40.1 to 55.0 min). Full mass scans were recorded in a positive-ionization mode of ESI with high resolution (LTQ-FT-ICR-MS, 100,000@m/z400) and MS/MS were acquired on the five most intense ions from each full scan by ion trap, covering a mass range from m/z 100.0–1500.0. All data obtained from the LC-MS analysis were acquired with the Xcalibur™ software (Thermo Fisher Scientific). Detection and structural speculation of cucurbitacins were determined by comparing the obtained data with the accurate mass, MS/MS spectra.
Results
Search for triterpene biosynthetic genes from M. charantia by RNA-seq
In order to identify genes involved in triterpene biosynthesis from M. charantia, total RNAs from ten different types of tissues, which were old leaves, young leaves, stems, tendrils, male flowers, female flowers, fruits, seedling leaves, seedling stems and seedling roots were prepared for RNA-seq using next-generation sequencing, Genome Analyzer IIx, HiSeq 1000, and HiSeq 1500 Rapid-Run Mode (Illumina). As a result, after assembling and quality checking, we obtained 72,577 non-redundant contigs. RPKM calculations were carried out in order to compare the expression levels.
We explored OSC genes from M. charantia by performing a blast search using well-known plant OSC genes as a query and identified four different OSC genes. These genes exhibited 50–72% sequence identity to each others (Table S2). Phylogenetic analysis was carried out for the obtained genes together with other known OSC genes to reveal that each of the four were distributed into different clades (). Since four major triterpenes were expected to derive from oxidosqualene in M. charantia as shown in , the result was consistent with our prediction. Each of the four genes showed a close similarity to cucurbitadienol, isomultiflorenol, β-amyrin and cycloartenol synthases from other plants. Therefore, the genes were named McCBS, McIMS, McBAS and McCAS, respectively. McCBS showed 88.7% amino acid sequence identity to cucurbitadienol synthase from Cucurbita pepo, while McIMS showed 93.1% identity to isomultiflorenol synthase from Luffa cylindrica. The amino acid sequence alignment showed conservation of motifs such as QW motif and DCTAE motif (). Consistent with our functional prediction, both McCBS and McCAS had Ile residue at a position two residues upstream from the DCTAE motif, while McIMS and McBAS had Val residue at this position. The Ile residue in CAS plays an important role in producing cycloartenol [Citation15] whereas the same Ile residue was also found in CBS from C. pepo [Citation16]. Most other OSCs have Val residue including isomultiflorenol and β-amyrin synthases. Moreover, MWCXC motif also showed a noticeable difference between them. Both McCBS and McCAS had His residue at the X position in this motif indicative of producing a tetracyclic product, while McIMS and McBAS had Tyr residue possibly for a pentacyclic product [Citation17]. Therefore, McCBS was most likely a cucurbitadienol synthase of M. charantia and was involved in cucurbitacins biosynthesis while McIMS likely to be an isomultiflorenol synthase.
Figure 2. Phylogenetic analysis of four OSC genes from M. charantia with various plant OSC genes. The boxed genes represent McCBS, McIMS, McBAS and McCAS. Underline indicates BASs; β-amyrin synthases, and shaded areas are CASs; cycloartenol synthases, CBSs; cucurbitadienol synthases and IMSs; isomultiflorenol synthases. The detail of known OSCs is listed in Table S3.
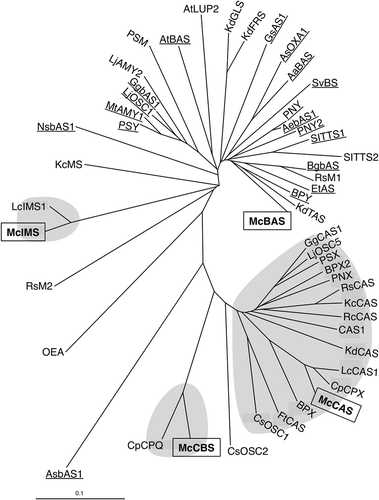
Functional analysis of four OSCs from M. charantia using heterologous expression system in S. cerevisiae strain GIL77
To test the activity of four OSCs from M. charantia, we performed heterologous expressions using S. cerevisiae strain GIL77. This strain lacked native lanosterol synthase activity and had been used for functional analysis of plant OSCs [Citation14]. All four genes were individually introduced into pESC-Ura. Yeast cells expressing each McCAS, McCBS, McBAS or McIMS were cultured, expression induced by galactose and the hexane extracts were analyzed by GC-MS (, ). As a control, yeast cells expressing Pisum sativum cycloartenol synthase (PSX) [Citation18] or C. pepo cucurbitadienol synthase (CPQ) were used. As a result, GC-MS analysis of the extract from yeast expressing McCAS showed a production of cycloartenol as compared with the extract from PSX expressing yeast. McCBS expression also resulted in a production of a peak identical with cucurbitadienol produced by CPQ as shown by both retention time and MS spectra. McBAS expression resulted in a peak that was identical both in retention time and MS spectra to an authentic β-amyrin. On the other hand, McIMS expression gave a single product peak that was absent from the control experiment. In order to identify the product, a large scale culture (4 L) was performed and the product extracted, purified and analyzed by NMR (Figure S1-S3). Eight singlet methyl signals (one overlapped) were observed in 1H-NMR together with a hydroxy methine proton (δ 3.22 dd) typical of a 3α-H of triterpene alcohol (Figure S2). The absence of any olefinic proton indicated the presence of a tetrasubstituted double bond. Accordingly, one set of an olefin was seen in 13C-NMR spectrum (δ 133.4, 135.0), as was a hydroxy bearing carbon (δ 78.9) (Figure S3). Moreover, the absence of vinylic methyl signals around 1.6 ppm suggested of a pentacyclic structure. All the NMR data were in agreement with a product isomultiflorenol and the chemical shifts were assigned as shown in Figure S1 by comparing with literature values for related compounds [Citation19–Citation21]. Therefore, McIMS was identified as the isomultiflorenol synthase of M. charantia. These results demonstrated that four OSCs identified from RNA-seq analysis were confirmed to be involved in triterpenoid biosynthesis in M. charantia.
Figure 4. GC-MS chromatogram of hexane extracts from yeast transformants harboring each OSC gene. These GC-MS chromatograms are in an order from the top; hexane extracts from yeast expressing McCBS, CPQ, PSX, McCAS, McIMS, McBAS, authentic β-amyrin and empty vector. Dotted line shows OS (2,3-oxidosqualene), DOS (dioxidosqualene), Erg (ergosterol), cucurbitadienol, cycloartenol and β-amyrin.
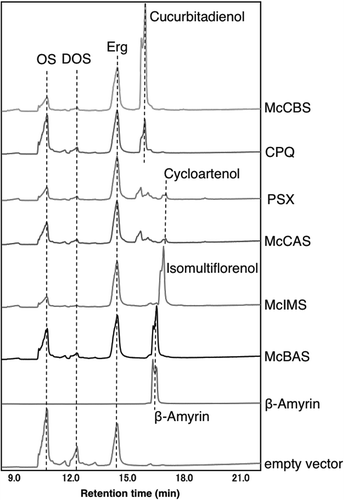
Figure 5. Mass spectra of β-amyrin or cucurbitadienol produced by McBAS or McCBS. These mass spectra are in an order from the top; authentic β-amyrin (retention time: 16.5 min, ), β-amyrin from McBAS-expressing yeast (retention time: 16.5 min, ), cucurbitadienol from CPQ-expressing yeast (retention time: 16.0 min, ) and cucurbitadienol from McCBS-expressing yeast (retention time: 16.0 min, ).
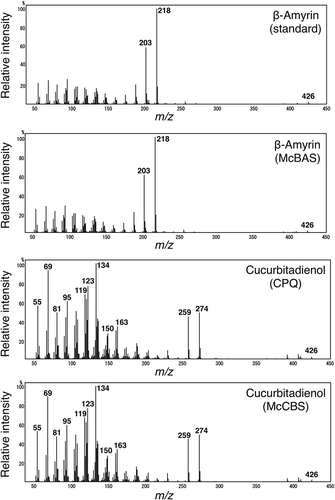
Validation for expression pattern of four OSC genes by RT-PCR analysis
The expression profile deduced from RPKM values of RNA-seq analysis pointed to a discrepancy among OSCs (). McCBS showed the highest read from leaves while low from fruits. Both McIMS and McBAS had reads exclusively from roots. On the other hand, McCAS showed ubiquitous reads from all the tissues tested in comparable amounts. Accordingly, representative mevalonate pathway genes also showed ubiquitous reads from all the tissues, although some large discrepancy in expression pattern was seen among isogenes (Figure S4 and Table S4). Particularly, one of the squalene epoxidase gene (SQE_05234) showed a similar expression pattern to McCBS, which might be an isogene dedicated for cucurbitacins biosynthesis. To confirm the expression patterns of four OSC genes in RNA-seq analysis, we investigated the mRNA levels from six tissues of M. charantia with quantitative RT-PCR analysis. As a result, the expression of McCBS, which encodes cucurbitadienol synthase involved in the biosynthesis of cucurbitacins, was much higher in leaves than in fruits (). On the other hand, the expression of McBAS and McIMS were mostly seen in roots, while McCAS was expressed ubiquitously in all tissues tested. These results were in agreement with RPKM values from RNA-seq data. Surprisingly, McCBS was found mainly expressed in the leaves, even though it had been considered that cucurbitacins are accumulated in fruits where bitter compounds accumulate. This raises the possibility that cucurbitacins or its intermediates might be biosynthesized in the leaves and then transported to the fruits. The expression pattern of McBAS and McIMS, which encodes β-amyrin and isomultiflorenol synthases, respectively, was different from that of McCBS. These results demonstrated the difference in the distribution of each expressed OSC gene in M. charantia.
Metabolite analysis from each tissue of M. charantia
In order to see if the observed expression patterns among different tissues correlated with metabolite profiles, metabolite analysis was carried out on methanolic extracts from each tissue by LC-MS and extracted ion chromatograms were drawn (Figure S5 and Table S5). Due to a lack of a standard sample, two types of cucurbitacins, mono- and diglycosides, were deduced from their molecular masses (Figure S5-E and S5-F). The predicted cucurbitacins peaks were mainly observed in roots, while predicted monoglycosides were also seen in fruits, however both were not observed in leaves (Figure S5-C and S5-D). Therefore, the expression of McCBS in roots seemed to be correlated with metabolites data while that of leaves did not. Since many different cucurbitacins were isolated from M. charantia, cucurbitacins with different molecular mass might be present in leaves. More detailed analysis is certainly needed in the future.
Discussion
A characteristic triterpenoids cucurbitacins exhibit a wide spectrum of pharmacological activities such as anti-inflammatory and anticancer activities [Citation4]. Thus, Cucurbitaceae producing large amounts of cucurbitacins serves not only as a food but also as a medicine. In particular, many cucurbitane triterpenes were isolated from the fruits, seeds and roots of M. charantia [Citation8,Citation22,Citation23]. These triterpenes also exhibit antidiabetic, anticancer and antiviral activities [Citation9,Citation22,Citation24,Citation25]. In this study, we identified the four OSC genes each encoding cucurbitadienol, isomultiflorenol, β-amyrin and cycloartenol synthases using RNA-seq datasets. The number of OSC from M. charantia was consistent with our expectation from the previous report on the isolation of triterpenes produced by M. charantia [Citation8,Citation11]. Both McCBS and McIMS were suggested to be involved in the production of characteristic triterpenoids in Cucurbitaceae. The close relationship of McCBS and McIMS to each of the homologues from C. pepo (CPQ) and L. cylindrica (LcIMS1) [Citation26], respectively, indicated that these genes were conserved among Cucurbitaceae and perhaps originated at an ancestral stage of this genus. Consistent with cyclization mechanisms, CBSs were closer to cycloartenol synthases, while IMSs were close to β-amyrin synthases and others producing pentacyclic triterpenes. Since CASs and BASs were ubiquitously present in higher plants, CBSs and IMSs were probably derived and evolved from CASs and BASs, respectively, mainly in Cucurbitaceae. Physiological roles of cucurbitane and multiflorane type triterpenes in this particular genus are largely unknown at this moment. Future studies may illuminate why these triterpenes evolved in this particular genus and what evolutionary advantages they might have had.
Expression patterns of the four OSCs deduced from RPKM values pointed to a striking fact that McCBS gene was expressed mainly in leaves and not in fruits, although it had been considered that a major accumulation site of cucurbitacins was in fruits where a strong bitter taste was observed. Only a small amount of reads was observed in fruits. Therefore, it was suggested that cucurbitacins might be biosynthesized in leaves, and then transported to fruits. Indeed cucurbitacins have been isolated from leaves or other tissues including roots [Citation22,Citation27]. However, direct comparison of cucurbitacins contents among different tissues have been missing. Our LC-MS analysis of predicted cucurbitacins could not fully support the expression data. The lack of predicted cucurbitacins peaks in leaves do not rule out the presence of cucurbitacins in leaves. Due to a lack of a standard sample, more rigorous analysis is required to study the cucurbitacins profile among different tissues. Our current method reported here would be useful in illuminating this point in the future. Whether a precursor cucurbitadienol is transported to fruits and modified, or a more advanced intermediate or a final product is transported needs to be studied in the future as well. This will require an identification of P450 genes involved in cucurbitacins biosynthesis.
Our identification of major OSCs in M. charantia uncovered the initial steps of triterpenoids biosynthesis and opened up a possibility for a future production of useful cucurbitacins in microbial hosts for a drug development. At the same time, these genes could be utilized for a molecular breeding producing M. charantia lines with altered triterpenoids content, perhaps may control the degree of bitterness.
Author Contribution
Shohei Takase (ST), Kota Kera, Yuya Hirao, Tsutomu Hosouchi, Yuki Kotake, Yoshiki Nagashima and Kazuto Mannen performed experiments. Hideyuki Suzuki and Tetsuo Kushiro supervised the present study, and wrote the manuscript along with ST.
Supplemental_material_Fig_S1-5_and_Table_S1-5_180925.pdf
Download PDF (933.4 KB)Acknowledgments
We would like to thank YAE NOGEI CO., LTD, for giving us seeds of Momordica charantia (Erabu, cv.) and Dr. Shinji Mizuno (Southern Prefectural Horticulture Institute in Chiba Prefectural Agriculture and Forestry Research Center) for his help with the cultivation of M. charantia. We also thank Ms. Sayaka Shinpo (Kazusa DNA Research Institute) for technical support in Illumina sequencing. We also thank Meiji University for financial support.
Disclosure statement
No potential conflict of interest was reported by the authors.
Supplementary material
Supplemental data for this article can be accessed here.
Additional information
Funding
References
- Mahato SB, Sen S. Advances in triterpenoid research, 1990–1994. Phytochemistry. 1997;44:1185–1236.
- Thimmappa R, Geisler K, Louveau T, et al. Triterpene biosynthesis in plants. Annu Rev Plant Biol. 2014;65:225–257.
- Seki H, Tamura K, Muranaka T. P450s and UGTs: key players in the structural diversity of triterpenoid saponins. Plant Cell Physiol. 2015;56:1463–1471.
- Chen JC, Chiu MH, Nie RL, et al. Cucurbitacins and cucurbitane glycosides: structures and biological activities. Nat Prod Rep. 2005;22:386–399.
- Huang S, Li R, Zhang Z, et al. The genome of the cucumber, Cucumis sativus L. Nat Genet. 2009;41:1275–1281.
- Shang Y, Ma Y, Zhou Y, et al. Biosynthesis, regulation, and domestication of bitterness in cucumber. Science. 2014;346:1084–1088.
- Zhou Y, Ma Y, Zeng J, et al. Convergence and divergence of bitterness biosynthesis and regulation in Cucurbitaceae. Nat Plants. 2016;2:1–8.
- Murakami T, Emoto A, Matsuda H, et al. Medicinal foodstuffs. XXI. Structures of new cucurbitane-type triterpene glycosides, goyaglycosides-a, -b, -c, -d, -e, -f, -g, and -h, and new oleanane-type triterpene saponins, goyasaponins I, II, and III, from the fresh fruit of Japanese Momordica charantia L. Chem Pharm Bull. 2001;49:54–63.
- Tan MJ, Ye JM, Turner N, et al. Antidiabetic activities of triterpenoids isolated from bitter melon associated with activation of the AMPK pathway. Chem Biol. 2008;15:263–273.
- Tabata M, Tanaka S, Cho HJ, et al. Production of an anti-allergic triterpene, bryonolic acid, by plant cell cultures. J Nat Prod. 1993;56:165–174.
- Akihisa T, Kimura Y, Kasahara Y, et al. 7-oxodihydrokarounidiol-3-benzoate and other triterpenes from the seeds of cucurbitaceae. Phytochemistry. 1997;46:1261–1266.
- Suzuki Y, Kawazu T, Koyama H. RNA isolation from siliques, dry seeds, and other tissues of Arabidopsis thaliana. BioTechniques. 2004;37:542–544.
- Han R, Takahashi H, Nakamura M, et al. Transcriptome analysis of nine tissues to discover genes involved in the biosynthesis of active ingredients in Sophora flavescens. Biol Pharm Bull. 2015;38:876–883.
- Kushiro T, Shibuya M, Ebizuka Y. β-Amyrin synthase cloning of oxidosqualene cyclase that catalyzes the formation of the most popular triterpene among higher plants. Eur J Biochem. 1998;256:238–244.
- Lodeiro S, Schulz-Gasch T, Matsuda SP. Enzyme redesign: two mutations cooperate to convert cycloartenol synthase into an accurate lanosterol synthase. J Am Chem Soc. 2005;127:14132–14133.
- Shibuya M, Adachi S, Ebizuka Y. Cucurbitadienol synthase, the first committed enzyme for cucurbitacin biosynthesis, is a distinct enzyme from cycloartenol synthase for phytosterol biosynthesis. Tetrahedron. 2004;60:6995–7003.
- Kushiro T, Shibuya M, Masuda K, et al. Mutational studies on triterpene synthases: engineering lupeol synthase into β-amyrin synthase. J Am Chem Soc. 2000;122:6816–6824.
- Morita M, Shibuya M, Lee M-S, et al. Molecular cloning of pea cDNA encoding cycloartenol synthase and its functional expression in yeast. Biol Pharm Bull. 1997;20:770–775.
- Takai M, Tori M, Tsuyuki T, et al. Boron trifluoride etherate-catalyzed backbone rearrangement of 5α,10α-epoxyalnusan-3β-yl acetate and partial synthesis of isomultiflorenol. Bull Chem Soc Jpn. 1985;58:185–193.
- Faure R, Gaydou EM. Application of inverse-detected two-dimensional heteronuclear-correlated NMR spectroscopy to the complete carbon-13 assignment of isomultiflorenyl acetate. J Nat Prod. 1991;54:1564–1569.
- Chang Y-S, Lin M-S, Jiang R-L, et al. 20-Epibryonolic acid, phytosterols and ellagic acid from Coriaria intermedia. Phytochemistry. 1996;42:559–560.
- Chen J, Tian R, Qiu M, et al. Trinorcucurbitane and cucurbitane triterpenoids from the roots of Momordica charantia. Phytochemistry. 2008;69:1043–1048.
- Liu JQ, Chen JC, Wang CF, et al. New cucurbitane triterpenoids and steroidal glycoside from Momordica charantia. Molecules. 2009;14:4804–4813.
- Zeng K, He Y-N, Yang D, et al. New compounds from acid hydrolyzed products of the fruits of Momordica charantia L. and their inhibitory activity against protein tyrosine phosphatase 1B. Eur J Med Chem. 2014;81:176–180.
- Akihisa T, Higo N, Tokuda H, et al. Cucurbitane-type triterpenoids from the fruits of Momordica charantia and their cancer chemopreventive effects. J Nat Prod. 2007;70:1233–1239.
- Hayashi H, Huang P, Inoue K, et al. Molecular cloning and characterization of isomultiflorenol synthase, a new triterpene synthase from Luffa cylindrica, involved in biosynthesis of bryonolic acid. Eur J Biochem. 2001;268:6311–6317.
- Fatope MO, Takeda Y, Yamashita H, et al. New cucurbitane triterpenoids from Momordica charantia. J Nat Prod. 1990;53:1491–1497.