ABSTRACT
Conidiogenone, a diterpene with a unique structure, is known to induce the conidiation of Penicillium cyclopium. The biosynthetic pathway of (−)-conidiogenone has been fully elucidated by the heterologous expression of biosynthetic genes in Aspergillus oryzae and by in vitro enzyme assay with 13C-labeled substrates. After construction of deoxyconidiogenol by the action of bifunctional terpene synthase, one cytochrome P450 catalyzes two rounds of oxidation to furnish conidiogenone. Notably, similar biosynthetic genes are conserved among more than 10 Penicillium sp., suggesting that conidiogenone is a common conidiation inducer in this genus. The cyclization mechanism catalyzed by terpene synthase, which involves successive 1,2-alkyl shifts, was fully elucidated using 13C-labeled geranylgeranyl pyrophosphate (GGPP) as substrate. During the structural analysis of deoxyconidiogenol, we observed broadening of some of the 13C signals measured at room temperature, which has not been observed with other structurally related compounds. Careful examination using techniques including 13C NMR studies at −80 °C, conformational analysis and prediction of the 13C chemical shifts using density functional theory gave insights into this intriguing phenomenon.
Graphical Abstract
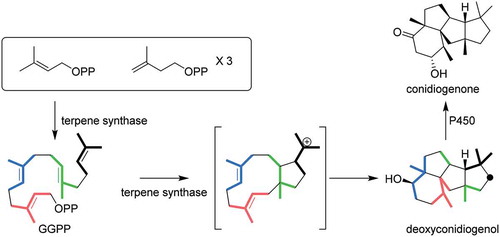
Heterologous expression of bifunctional diterpene synthase and P450 monooxygenase genes from two Penicillium sp. afforded conidation inducing factor conidiogenone.
Diterpene conidiogenone 1 (), first isolated from Penicillium cyclopium, is an inducer of conidiation [Citation1]. In the presence of 20 ng mL−1 of 1, the time required for conidiation of P. cyclopium is decreased [Citation2]. Conidiation refers to the asexual reproduction of filamentous fungi and is a morphogenetic process that they undertake for dispersal. Conidiation is also important for pathogenicity of phytopathogens. Therefore, understanding the cellular mechanism of conidiation is an important and urgent research topic. In addition to 1 and its putative precursor conidiogenol, more than 10 related diterpenes, conidiogenone B-K and conidiogenol B, have been isolated from the genus Penicillium [Citation3–Citation5]. Among the known congeners called cyclopianes, conidiogenone C exhibits cytotoxicity against HL-60 cells, while conidiogenone B shows antibacterial activity against methicillin-resistant Staphylococcus aureus (MRSA). The diverse biological activities of these structurally related diterpenes are very interesting. The unique structural features of the diterpenes, including the highly fused ring systems, 6–8 consecutive stereocenters, and four congested quaternary carbon centers, are also intriguing and pose a challenge for the chemical synthesis of cyclopianes. Asymmetric total synthesis of conidiogenol, conidiogenone, and conidiogenone B was achieved in 2016, and it led to the determination of their absolute configurations [Citation6]. Despite these extensive efforts toward the isolation and chemical synthesis, the biosynthetic pathway for cyclopianes remains to be elucidated.
Bifunctional terpene synthases (BFTSs) are multifunctional enzymes composed of an N-terminal terpene synthase (TS) domain and a C-terminal prenyltransferase (PT) domain. The first reported example of a BFTS was fusicoccadiene synthase (PaFS) derived from Phomopsis amygdali [Citation7]. A second example, phomopsene synthase (PaPS), was also identified in the same strain [Citation8]. Besides these C20 diterpene synthases, BFTSs are also involved in the biosynthesis of C25 sesterterpenes. Since the identification of the first example of a sesterterpene synthase (AcOS) [Citation9], more than 10 BFTSs have been characterized [Citation10–Citation16]. During the course of genome mining of fungal BFTSs, we found one BFTS that is highly conserved among the genus Penicillium. Herein, we report the heterologous production of 1 employing the Aspergillus oryzae expression system and elucidation of the cyclization mechanism of a key terpene synthase by in vitro analysis with 13C-labeled substrates. In addition, broadening of specific signals in the 13C NMR spectrum of deoxyconidiogenol is discussed using the density functional theory (DFT).
Results and discussion
CDNA cloning of the PchDS gene
Our expectation that Penicillium sp. commonly produce conidia-forming factor 1 as well as structurally related congeners prompted us to search gene clusters on their genome. Considering phomopsene and 1 likely share a common biosynthetic pathway for the construction of their characteristic tetracyclic molecular skeletons [Citation8], PaFS and PaPS genes were used as query sequences for BLAST. This enabled us to find BFTS gene Pc20g10860 (PchDS) in the genome of Penicillium chrysogenum (Penicillium rubens Wiconsin 54–1255). We then obtained two strains, P. chrysogenum MAFF305174 and MAFF111241; the former white strain did not produce conidia, but the latter moss green strain did produce conidia. With this information, we attempted to prepare cDNA using total RNA from both strains. Only in the case of the moss green strain did we obtain the cDNA with the expected size of the PchDS gene (2,223 bp encoding 740 amino acid residues) (Figure S1). Recombinant PchDS fused with GST was produced in Escherichia coli and used for enzymatic reactions after affinity column purification. Incubations of PchDS with GGPP gave a new product, 2. In the GC-MS analysis of the extracts, the molecular ion of 2 was observed at m/z 290, indicating that 2 is a diterpene alcohol ()).
Figure 2. GC-MS and LC-MS profiles of the extracts from enzymatic reaction and heterologous expression.
(a). GC-MS profile of PchDS reaction. Upper panel: chromatogram showing total ion current (TIC). Lower panel: MS spectrum of 2. (b). GC-MS profile of metabolites extracted from AO-PrDS and A. oryzae NSAR1. Upper panel: chromatograms extracted at m/z 290. Lower panel: MS spectrum of 2. (c). LC-MS profile of AO-PrDS and AO-PrDS/PrP450. Chromatograms are extracted at m/z 305.
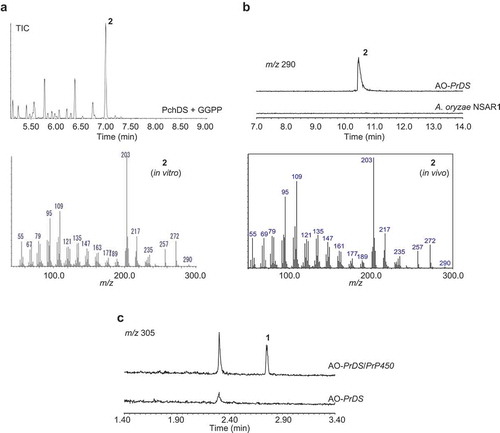
Heterologous expression of the biosynthetic genes derived from Penicillium roqueforti
In the PchDS BLAST search, highly homologous genes were found in other Penicillium sp. One of the genes, PrDS, identified from Penicillium roqueforti showed significant homology to PchDS. We also employed an Aspergillus oryzae expression system to characterize PrDS. First, we introduced PrDS into the plasmid pTAex3 to construct the plasmid pTAex3-PrDS. A. oryzae was transformed with the plasmid to generate the transformant harboring PrDS gene (AO-PrDS). A new metabolite that is identical to the PchDS product 2 was readily identified by GC-MS analysis of the extracts from the resulting AO-PrDS ()). Based on HR-MS data (2: m/z 290.2602 [M]+), the molecular formula of 2 was determined to be C20H34O. The 1H NMR spectrum of 2 showed one oxymethine proton (δH 3.41 (dd, H6)), indicating that 2 contains a secondary alcohol. The presence of five methyl signals (δH 0.93 (d, H18), 0.99 (s, H16), 1.05 (s, H20), 1.14 (s, H19), 1.31 (s, H17)) suggested that 2 is a diterpene derived from geranylgeranyl pyrophosphate (GGPP). Two of the methyl groups (C18 and C19) shared a neighboring carbon (C2), as shown by HMBC correlations from H18 to C2, C3, and C4, and from H19 to C2, C6, and C7. Together with the proton spin system (H18-H3-H4-H5-H6), the presence of an oxygenated six-membered ring was proposed. C14, which exhibited an unusual chemical shift (δC 74.1), was close to the remaining three methyl groups, as indicated by HMBC. The second spin system (H14-H10-H9-H8), as well as HMBC correlations such as H1-C2, C7, and C10, H8-C6, and H10-C15, established the second and third ring systems. Further 2D-NMR analysis confirmed that the planar structure of 2 was deoxyconidiogenol (Figure S2, Table S1). NOEs between H6 and H10, H10 and H16, and H17 and H20 allowed us to establish the relative configuration (Figure S2). Considering that 2 was converted to 1 whose absolute configuration had already been determined [Citation6], the absolute configuration of 2 was established to be 2S,3S,6R,7R,10S,11R,14R. During the structure determination of 2, we observed specific signal broadening for C1, C3, C5, and C19 in the 13C NMR spectrum. We will discuss this unusual observation later.
Given that 2 has the same carbon skeleton as 1, we turned our attention to the oxidative modification of 2. As such, PrP450, which was found in the franking region of PrDS, was introduced into AO-PrDS with the plasmid pAdeA2-PrP450 to construct AO-PrDS/PrP450. LC-MS analysis of the extracts obtained from the resultant transformant led to the identification of a new metabolite with m/z 305 [M + H]+ ()). The NMR data and the optical rotation value of this compound were in good agreement with those of (−)-conidiogenone (1) [Citation1]. These results showed that PrP450 is involved in the oxidative modifications of 2 to give 1, showing that P. roqueforti is a conidiogenone producer. Consequently, P. chrysogenum, which has the homologous P450 gene (PchP450: Pc20g10870), was proposed to be a producing strain as well.
Identification of homologous genes in other Penicillium sp
To investigate the distribution of conidiogenone producers, we conducted a BLAST search using the amino acid sequence of PrDS as a query. Highly homologous genes were found in other Penicillium sp., including P. italicum, P. nalgiovense, P. expansum, P. camemberti, P. chrysogenum, P. solitum, P. nordicum, P. arizonense, P. antarcticum, and P. rubens (each protein shows 77–91% identity). All of them had genes encoding P450s that were homologous to PchP450/PrP450 in the franking region of PchDS/PrDS homologs, suggesting that these participate in the conidiogenone biosynthesis (Figure S3, Tables S2 and S3). Filamentous fungi have many gene clusters, and one of them may be a signal transduction system for conidiogenesis.
Cyclization mechanism mediated by deoxyconidiogenol synthase
In our previous paper, we pointed out that mangicol, phomopsene, and conidiogenone likely share a common biosynthetic pathway for the construction of their characteristic tetracyclic molecular skeletons [Citation8]. The cyclization mechanisms of mangicol and variediene were extensively studied via the labeling pattern of doubly labeled sodium acetate [Citation14,Citation17]. Recently, phomopsene was also studied by enzymatic reaction of phomopsene synthase PaPS with specifically labeled FPP [Citation18]. The cyclization mechanism of another phomopsene synthase of bacterial origin was also characterized employing [13C20]GGPP as a substrate [Citation19]. In this study, we used a different approach to elucidate the mechanism of the construction of the doexyconidiogenol skeleton. For this purpose, we enzymatically synthesized [U-13C]labeled 2 by incubation of [U-13C6]mevalonate using PchDS and a common enzyme mixture for GGPP synthesis with isopentenyl diphosphate isomerase according to a method described previously [Citation20,Citation21]. Similarly, partially 13C-labeled products [13C15]-2, [13C10]-2, and [13C5]-2 were obtained by incubation of various starter units (DMAPP, GPP, and FPP) in the absence of IPP isomerase. Since all carbon atoms were enriched with the 13C isotope, carbon–carbon couplings were observed in the spectrum (, ). Although these data are sufficient to determine the planar structure of the new product, deoxyconidiogenol (2), we do not provide the detailed explanation here to avoid duplication with that for the PrDS described in the previous section.
Table 1. 13C chemical shifts and multiplicity of [13C20]-2 (CDCl3, 150 MHz).
Figure 3. 13C NMR spectra of 13C-labeled 2 enzymatically synthesized by PchDS (CDCl3, 150 MHz).
The solvent signal at δC 77.0 was used as a reference.
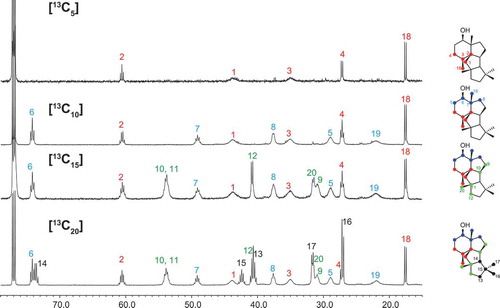
The assignment of the 13C-signals shown in enabled us to identify the isoprene units I (C1 to C4 + C18Me) and II (C5 to C8 + C19Me), which were labeled according to the expected pattern of the GGPP backbone, as seen in . In contrast, the labeling patterns of units III (C9–C10/C11–C12 + C20Me) and IV (C13/C14/C15–C16 + C17Me) were deformed and were different from those of the original GGPP. Comparison of the 13C-NMR spectra of [13C15]-2 and [13C20]-2 revealed the following: 1) C14 of unit IV is inserted between C10 and C11 of unit III by breaking the original C10–C11 bond; 2) the original C13–C14 bond is broken, and a C13–C15 bond is formed. These observations allowed us to propose a cyclization pathway catalyzed by PchDS involving sequential 1,2-alkyl shifts, as shown in . This sequential rearrangement has been observed in the biosynthesis of mangicol, variediene (kojidiene), and phomopsene catalyzed by FgMS [Citation15] (clade B), EvVS [Citation11] (clade C), and PaPS [Citation8,Citation18,Citation19] (clade B), respectively. Including PrDS and PchDS (clade D), all of these terpene synthases utilize the type B cyclization mechanism according to the previous classification [Citation22]. Among the cyclization processes catalyzed by PchDS, 1,2-H shift and subsequent C2-C7 bond formation of the intermediate B-12+, which is also generated during the EvVS- and PaPS-catalysis, are the unique processes to the PchDS-catalyzed cyclization that synthesizes the core skeleton of 2 ().
Deoxyconidiogenol signal broadening
During the structure determination and biosynthetic study, we encountered broadening of some signals in the 13C-NMR spectrum of deoxyconidiogenol measured at room temperature ()). Intriguingly, no other structurally related compounds such as conidiogenone derivatives or synthetic intermediates showed any broadening [Citation3,Citation6]. In addition, this broadening was not observed in the spectrum measured at −80°C ()). To examine this phenomenon in detail, we performed calculations on the conformations of 2 using DFT. After optimization at the B3LYP/6–31 + G(d, p) level, two sets of six conformers (M0001-M0006 and M0007-M0012, Table S2) originating from the flexible five-membered rings were found for chair conformers Iax and IIeq, respectively (). Note that ax and eq refer to the orientation of the C6-hydroxyl group. The energy difference between the type-I and type-II conformers is within 3.14 kJ mol−1 (Table S3).
Figure 4. 13C NMR spectra of deoxyconidiogenol (2) measured at room temperature (a, toluene-d8, 125 MHz) and at −80 °C (b, toluene-d8, 150 MHz).
Chemical shifts are referenced to the methyl signal of toluene-d8 (δC 20.4).
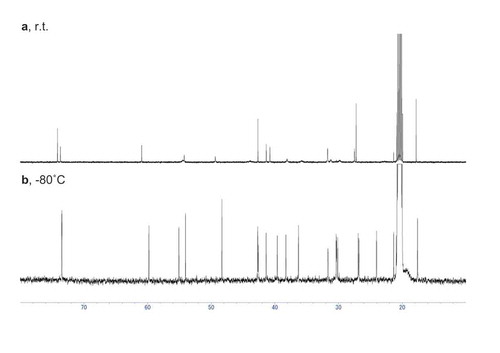
Figure 5. Stereostructures of the two conformers, Iax and IIeq.
M0001-M0006 are superposed for Iax (81% distribution) and M0007 and M0012 are superposed for IIeq (18% distribution).
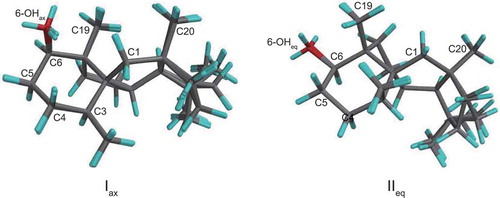
NMR chemical shifts of both I and II were computed by a DFT model (ωB97X-D functional with the 6-31G* basis set), and the results are listed in Table S5 [Citation23–Citation25]. Because of the presence of more than one contributing conformer, a Boltzmann-weighted average was used to represent the computed chemical shifts for each conformer of deoxyconidiogenol. The calculated chemical shifts were nearly identical to those observed at room temperature. Intriguingly, the chemical shifts for the major conformer were also in good agreement with those observed at −80 °C. Only in the DEPT spectrum of 2 at −80 °C were very small signals originating from the minor conformer also observed (Figure S4-S6), and those signals correlated well with the calculated data for the minor conformer. These results clearly indicate that the two conformers interconverted slowly at ambient temperature, and both conformers were observed at −80 °C because interconversion of the two conformers was significantly inhibited at this temperature. The calculated chemical shift difference between the two conformers in Table S6 indicated that significant signal broadening occurred for signals C1, C3, C5, C10, and C19, which all have shift differences larger than 4 ppm. Relatively minor signal broadening was also observed at C8, C9, and C20, with shift differences between 2.86 and 3.33 ppm. Therefore, the energy barrier between the two conformers is critical for the specific signal broadening. This may be why 2 is the only compound to show signal broadening among the conidiogenone derivatives.
Conclusion
We identified the biosynthetic genes for conidiogenone, a conidiation-inducing agent of P. cyclopium. Heterologous expression in A. oryzae facilitated isolation of the biosynthetic intermediate deoxyconidiogenol and the natural product (−)-conidiogenone. The cyclization mechanism catalyzed by the terpene synthase was further analyzed by enzymatic reactions with fully and partially 13C-labeled substrates. Recently, we reviewed cyclopentane-forming di/sesterterpene synthases (CPF-TSs) including bifunctional terpene synthases such as fusicoccadiene synthase (PaFS) and ophiobolin synthase (AcOS). Members of this family are widely distributed in bacteria, fungi, and plants. Based on our classification, deoxyconidiogenol synthases PchDS and PrDS are associated with TypeB_Path II cyclization. Our study provides the first experimental results for CPF-TSs classified in the clade D [Citation22], which will facilitate characterization of other enzymes in this clade. In addition to the homologs conserved among Penicillium sp., the CPF-TSs belonging to this clade can be found in other fungi including the phytopathogen Colletotrichum orbiculare MAFF 240,422 (Table S2). The structure and function of the corresponding diterpenes will be intriguing.
Experimental
General
All reagents commercially supplied were used as received. Column chromatography was carried out on 60N silica gel (Kanto Chemicals). Optical rotations were recorded on JASCO P-2200 digital polarimeter. 1H- and 13C-NMR spectra were recorded on Bruker DRX-500, Bruker AMX-500, and JEOL ECA-600 spectrometer (500/600 MHz for 1H-NMR and 125/150 MHz for 13C-NMR). NMR spectra were recorded in CDCl3 (99.8 atom% enriched, Kanto) and benzene-d6 (99.8 atom% enriched, Kanto). 1H chemical shifts were reported in δ value based on residual chloroform (7.26 ppm) and benzene (7.16 ppm) as a reference. 13C chemical shifts were reported in δ value based on chloroform (77.0 ppm), benzene (128.06 ppm) and toluene (20.4 ppm) as a reference. Data are reported as follows: chemical shift, multiplicity (s = singlet, d = doublet, t = triplet, q = quartet, m = multiplet, br = broad), coupling constant (Hz), and integration. GC-MS analyses were conducted with MS-2010 (Shimadzu). Mass spectra were obtained with a Waters ACQUITY QDa (ESI mode). HRMS was obtained with JMS-T100GCV (JEOL) in FD or FI mode. Oligonucleotides for polymerase chain reactions (PCRs) were purchased from Hokkaido System Science Co., Ltd. PCRs were performed with a BioRad S1000 thermal cycler.
Strains
Escherichia coli HST08 was used for cloning and following standard recombinant DNA techniques. A fungal host strain used in this study was A. oryzae NSAR1, a quadruple auxotrophic mutant (niaD−, sC−, ΔargB, adeA−), for fungal expression. P. chrysogenum mycelia (MAFF305174 and MAFF111241) were obtained from NARO Genebank in National Institute of Agrobiological Sciences in Japan and P. roqueforti IFM48062 was obtained from Medical Mycology Research Center (MMRC), Chiba University.
cDNA cloning of PchDS
Based on information of Pc20g10860 Penicillium chrysogenum (Penicillium rubens Wisconsin 54–1255) ORF 2,223 bp encoding 740 amino acid residues, PchDS open reading frame (ORF) cDNA fragment was amplified by RT-PCR, using a set of specific primers (PchDS-ORF-F: 5ʹ- ATGGCGGACAAAATAACCGACG-3ʹ, PchDS-ORF-R: 5ʹ- TCATTTCTGAAGCGTCTCTTCC-3ʹ) and Advantage 2 polymerase mix (Clonetech), from cDNA pools prepared from the liquid-cultured mycelia of P. chrysogenum MAFF305174 and MAFF111241 as templates. Amplified PCR fragment was subcloned into pGEM-T Easy vector (Promega) and determined its cDNA sequences (accession number of PchDS ORF is LC373222). For checking quality of cDNA templates, RT-PCR was performed to amplify β-tublin cDNA fragments using a specific primer set; tublin-F, CTCCTACGGTGACCTGAAC and tublin-R, AAGCCGACCATGAAGAAG.
Plasmid construction of PchDS cyclase domain
For expression as GST fusion proteins, the ORF cDNA of PchDS cyclase domain (N376) was re-amplified using the subcloned plasmid as templates and primer sets; PchDS-pGEX-F (gtggatccccgaattcaATGGCGGACAAAATAACCGACG) and PchDS-N376-pGEX-R (gtcgacccgggaattTCAAGGGTCTTTGAGCTCACTCT). Amplified PCR fragment was cloned into Eco RI-digested pGEX-4T-3 (GE Healthcare) by using In-Fusion HD cloning kit (Takara Bio).
Genomic DNA preparation
Genomic DNA of Penicillium roqueforti IFM48062 was extracted according to the following method; the mycelia of fungus was collected by filtration, washed with water, and dried using paper towel. The dried mycelia were frozen in liquid nitrogen and crushed by SK-Mill (Tokken). To the frozen powder was added extraction buffer (400 mM of Tris-HCl (pH 8.0), 500 mM of NaCl, 20 mM of ethylenediaminetetraacetic acid (EDTA) and 1 % of sodium dodecyl sulfate) and the suspension was kept at room temperature for 5 min. To the suspension was added phenol:chloroform solution and the mixture was vortexed for 2 sec. After incubation at 65 °C for 60 min, the reaction mixture was centrifuged at 12,000 rpm for 5 min. The supernatant was then treated with RNase at 37°C for 90 min. To the reaction mixture was then added phenol:chloroform solution. After being vortexed for 2 sec, the mixture was centrifuged at 12,000 rpm for 5 min. The supernatant was transferred to a new centrifuge tube and re-extracted twice with phenol:chloroform (1:1) solution followed by chloroform. To the final supernatant was added cold-isopropanol and CH3COONa solution and genomic DNA was recovered by centrifugation at 12,000 rpm for 10 min. The pellet was then washed with 70 % ethanol solution and dried for 15 min. Finally, the isolated DNA was resuspended in TE buffer (10 mM of Tris-HCl (pH 8.0) and 1mM of EDTA) and stored at −20 °C for further use.
Construction of A. oryzae expression plasmids
The PrDS and P450 were amplified from the genomic DNA with primer set as follows:
PrDS-F (SmaI site); TCGAGCTCGGTACCCATGGGCGAAACAATAGCCG,
PrDS-R (SmaI site); CTACTACAGATCCCCTCATTTCTGAGATGCCTCTT,
P450-F (KpnI site); CGGAATTCGAGCTCGATGCTATTACTGTGGTTTGG,
P450-R (KpnI site); ACTACAGATCCCCGGCTACGGGTCACGAACCCG.
PCR reactions were performed with the KOD-Plus-Neo (TOYOBO). Each PCR product was inserted into appropriate restriction site of pTAex3 or pAdeA2 using In-Fusion Advantage PCR cloning kit (Clontech Laboratories) to construct expression plasmids. The nucleotide sequences were identical to those reported for P. roqueforti FM164 (Accession No. HG792016 for genome sequence; PrDS: PROQFM164_S02g003147; PrP450: PROQFM164_S02g003150).
Transformation of Aspergillus oryzae
Transformation of A. oryzae NSAR1 or AO-PrDS (1.0 × 108 cells) was performed by the protoplast-polyethylene glycol method reported previously to construct the following transformants; AO-PrDS and AO-PrDS/P450.
Extraction of metabolites
Mycelia of AO-PrDS and AO-PrDS/P450 were inoculated into a solid medium containing polished rice (100 g) and adenine (10 mg) in 500 mL Erlenmeyer flask. Each culture was incubated at 30°C for 12 days. After extraction with EtOAc, the extract was concentrated in vacuo to afford crude extracts. The crude sample were analyzed by GC-MS equipped with a DB-1ms capillary column (0.32 mm x 30 m, 0.25 μm film thickness; J&W Scientific) at the following conditions; the sample was injected into the column at 100°C in the splitless mode. After a 3 min isothermal hold at 100°C, the column temperature was increased at 14°C min−1 to 268°C, with a 4 min isothermal hold at 268°C. The flow rate of helium carrier gas was 0.66 mL min−1. After partial purification of the crude extracts of AO-PrDS/P450 by silica gel column chromatography (hexane/EtOAc = 1), the crude sample were analyzed by LC-MS equipped with a ACQUITY UPLC® BEH C18 column (50 mm x 2.1 mm) at the following condition; Solvent: A/B = 70/30, 0–0.5 min; A/B = 70/30→0/100, 0.5–3.5 min; A/B = 0/100, 3.5–6.0 min; A/B = 0/100→70/30, 6.0–6.01 min; A/B = 70/30, 6.01–8.0 min (A: H2O+0.1% HCOOH, B: CH3CN+0.1% HCOOH) at a flow rate of 0.7 mL/min with 210–500 nm detection.
deoxyconidiogenol (2) isolated from AO-PrDS
The crude extracts of AO-PrDS were partially purified with silica gel column chromatography (hexane/EtOAc = 50). Further purification with second round silica gel column chromatography (hexane/EtOAc = 10) gave 2 (29.5 mg from 1 kg of rice medium). HR-FDMS calcd. For C20H34O [M+] 290.2610, found 290.2602. [α]D25 4.6 (c 1.0, CHCl3). 1H NMR and 13C NMR data are summarized in Table S1. 1D and 2D NMR spectra are shown in supporting information (page S14-S21).
(−)-conidiogenone (1) isolated from AO-PrDS/PrP450
The crude extracts of AO-PrDS/P450 were partially purified with silica gel column chromatography (hexane/EtOAc = 10 to 1). Further purification with second round silica gel column chromatography (hexane/EtOAc = 10), followed by HPLC equipped with Wakopak ODS (ϕ10 x 250 mm) at the conditions (100% acetonitrile at a flow rate of 5 ml/min) gave 2 (8.1 mg from 1 kg of rice medium). HR-FIMS calcd. For C20H32O2 [M+] 304.2402, found 304.2397. [α]D25 −31 (c 0.2, CHCl3). 1H NMR and 13C NMR data are in good agreement with reported ones [Citation1]. 1H NMR and 13C NMR spectra are shown in supporting information (page S22–S23).
Enzymatic synthesis and purification of various 13C-labeled diterpene alcohol
For biosynthetic study of the PchDS product, fully 13C-labelled compound was enzymatically synthesized according to the method described previously [Citation21,Citation26]. In addition to the 8.8 mg of [U-13C6]mevalonate and six recombinant enzymes needed for synthesizing [U-13C20]GGDP, 7.5 mg of PchDS were added to the 67.5 ml reaction mixture. After the enzyme reaction at 30 °C for 16 h, the reaction products were extracted with n-hexane and separated from polar contaminants by silica gel column chromatography using a stepwise elution with n-hexane/EtOAc = 100/0, 100/8 and then 100/10. For the synthesis of partially 13C-labeled deoxyconidiogenol with [U-13C6]mevalonate, prenyl diphosphate substrates DMAPP, GPP, FDP were added as starter units to the common enzyme mixture eliminating isopentenyl diphosphate isomerase. The enzymatic reactions were analyzed by GC-MS (GC: Agilent 6890; MS: Agilent 5975C MSD) as described previously [Citation20].
To purify the enzyme product, the extract was loaded onto a column packed with silica gel (Bond Elut SI, 500 mg/3 ml; Agilent Technologies, Santa Clara, CA) and eluted with cyclohexane. The flow-through fraction was further purified by HPLC (Silver Column KANTO (5-μm particle, 4.6-mm inner diameter ϕ 250 mm; Kanto Chemical, Tokyo, Japan)) using cyclohexane as the mobile phase at 1 ml min–1 (column temperature, 40°C). The yields of the labeled 2 as follows; [U-13C20]-1: 110 μg; [13C15]-1: PchDS 121.5 μg; [13C10]-1: PchDS 595 μg; [13C5]-1: PchDS 142 μg.
Calculations
Molecular modeling calculations were performed on a PC (operating system: Windows7 Professional; CPU: Intel Xeon E5-1660 v2 processor, 3.70 GHz, 6 cores; RAM: 64 GB). Compound 2 was built on Spartan’16 (Wavefunction Inc. Irvine, CA 92,612 USA). Conformational search was performed with MMFF [Citation27] to give 25 candidate conformers. These were finely optimized with ωB97X-D/6-31G* model and the chemical shifts were calculated with the same model (Tutorial and User’s Guide, Spartan ‘16 for Windows, Macintosh and Linux; Wavefunction inc., 2016). Boltzmann distribution of each conformer was calculated based on the B3LYP/6-31G* after geometry optimization. Final geometries are summarized in supporting information (page S24–S59).
Author contributions
T. S., T. O., C. L., A. M., and H. O. designed the research plan with assistance from T. T., M. H., H. Koshino., and H. Kawaide. T. S. performed research for the PrDS experiments with assistance from T. O. and C. L. K. N., Y. F., T. T., and H. Kawaide performed research for the PchDS experiments. M. H. performed calculation and T. S., T. O., M. H., H. Koshino, and H. O. analyzed the data. T. S., T. O., A. M., and H. O. prepared manuscript with assistance from all authors. All authors reviewed and approved the final manuscript.
18BBB_conidiogenone_SI_R1_without-H.pdf
Download PDF (2.3 MB)Disclosure statement
No potential conflict of interest was reported by the authors.
Supplementary material
Supplementary data for this article can be accessed here.
Additional information
Funding
References
- Roncal T, Cordobés S, Ugalde U, et al Novel diterpenes with potent conidiation inducing activity. Tetrahedron Lett. 2002;43:6799–6802.
- Roncal T, Cordobés S, Sterner O, et al Conidiation in Penicillium cyclopium is induced by conidiogenone, an endogenous diterpene. Eukaryot Cell. 2002;1:823–829.
- Du L, Li DH, Zhu TJ, et al New alkaloids and diterpenes from a deep ocean sediment derived fungus Penicillium sp. Tetrahedron. 2009;65:1033–1039.
- Niu SW, Fan ZW, Xie CL, et al Spirograterpene A, a Tetracyclic spiro-diterpene with a fused 5/5/5/5 ring system from the deep-sea-derived fungus Penicillium granulatum MCCC 3A00475. J Nat Prod. 2017;80:2174–2177.
- Niu SW, Fan ZW, Tang XX, et al Cyclopiane-type diterpenes from the deep-sea-derived fungus Penicillium commune MCCC 3A00940. Tetrahedron Lett. 2018;59:375–378.
- Hou SH, Tu YQ, Wang SH, et al Total syntheses of the tetracyclic cyclopiane diterpenes conidiogenone, conidiogenol, and conidiogenone B. Angew Chem Int Edit. 2016;55:4456–4460.
- Toyomasu T, Tsukahara M, Kaneko A, et al Fusicoccins are biosynthesized by an unusual chimera diterpene synthase in fungi. Proc Natl Acad Sci U S A. 2007;104:3084–3088.
- Toyomasu T, Kaneko A, Tokiwano T, et al Biosynthetic gene-based secondary metabolite screening: a new diterpene, methyl phomopsenonate, from the fungus Phomopsis amygdali. J Org Chem. 2009;74:1541–1548.
- Chiba R, Minami A, Gomi K, et al Identification of ophiobolin F synthase by a genome mining approach: a sesterterpene synthase from Aspergillus clavatus. Org Lett. 2013;15:594–597.
- Ye Y, Minami A, Mandi A, et al Genome mining for sesterterpenes using bifunctional terpene synthases reveals a unified intermediate of di/sesterterpenes. J Am Chem Soc. 2015;137:11846–11853.
- Qin B, Matsuda Y, Mori T, et al An unusual chimeric diterpene synthase from Emericella variecolor and its functional conversion into a sesterterpene synthase by domain swapping. Angew Chem Int Ed Engl. 2016;55:1658–1661.
- Matsuda Y, Mitsuhashi T, Quan Z, et al Molecular basis for stellatic acid biosynthesis: A genome mining approach for discovery of sesterterpene synthases. Org Lett. 2015;17:4644–4647.
- Okada M, Matsuda Y, Mitsuhashi T, et al Genome-based discovery of an unprecedented cyclization mode in fungal sesterterpenoid biosynthesis. J Am Chem Soc. 2016;138:10011–10018.
- Matsuda Y, Mitsuhashi T, Lee S, et al Astellifadiene: structure determination by NMR spectroscopy and crystalline sponge method, and elucidation of its biosynthesis. Angew Chem Int Ed Engl. 2016;55:5785–5788.
- Bian G, Han Y, Hou A, et al Releasing the potential power of terpene synthases by a robust precursor supply platform. Metab Eng. 2017;42:1–8.
- Mitsuhashi T, Rinkel J, Okada M, et al Mechanistic characterization of two chimeric sesterterpene synthases from Penicillium. Chemistry. 2017;23:10053–10057.
- Renner MK, Jensen PR, Fenical W. Mangicols: structures and biosynthesis of a new class of sesterterpene polyols from a marine fungus of the genus Fusarium. J Org Chem. 2000;65:4843–4852.
- Shinde SS, Minami A, Chen Z, et al Cyclization mechanism of phomopsene synthase: mass spectrometry based analysis of various site-specifically labeled terpenes. J Antibiot (Tokyo). 2017;70:632–638.
- Lauterbach L, Rinkel J, Dickschat JS. Two bacterial diterpene synthases from Allokutzneria albata produce bonnadiene, phomopsene, and allokutznerene. Angew Chem Int Edit. 2018;57:8280–8283.
- Sugai Y, Miyazaki S, Mukai S, et al Enzymatic total synthesis of gibberellin A4 from acetate. Biosci Biotech Bioch. 2011;75:128–135.
- Shimane M, Ueno Y, Morisaki K, et al Molecular evolution of the substrate specificity of ent-kaurene synthases to adapt to gibberellin biosynthesis in land plants. Biochem J. 2014;462:539–546.
- Minami A, Ozaki T, Liu C, et al. Cyclopentane-forming di/sesterterpene synthases: widely distributed enzymes in bacteria, fungi, and plants. Nat Prod Rep. 2018. DOI:10.1039/c8np00026c
- Lodewyk MW, Siebert MR, Tantillo DJ. Computational prediction of 1H and 13C chemical shifts: A useful tool for natural product, mechanistic, and synthetic organic chemistry. Chem Rev. 2012;112:1839–1862.
- Nguyen QNN, Tantillo DJ. Using quantum chemical computations of NMR chemical shifts to assign relative configurations of terpenes from an engineered Streptomyces host. J Antibiot. 2016;69:534–540.
- Hong YJ, Tantillo DJ. Is a 1,4-alkyl shift involved in the biosynthesis of ledol and viridiflorol? J Org Chem. 2017;82:3957–3959.
- Sugai Y, Ueno Y, Hayashi K, et al Enzymatic 13C labeling and multidimensional NMR analysis of miltiradiene synthesized by bifunctional diterpene cyclase in Selaginella moellendorffii. J Biol Chem. 2011;286:42840–42847.
- Halgren TA. Merck molecular force field .1. Basis, form, scope, parameterization, and performance of MMFF94. J Comput Chem. 1996;17:490–519.