ABSTRACT
The rice receptor-like cytoplasmic kinase 185 (OsRLCK185) interacts with the chitin receptor complex OsCERK1/CEBiP and positively regulates chitin-induced immune responses including MAP kinase activation, ROS production and defense gene expression. To elucidate the regulatory mechanisms of OsRLCK185-mediated immunity, we searched for interactors of OsRLCK185. OsDRE2a, rice homologs of the yeast Dre2 protein, were identified as novel interactors of OsRLCK185. OsDRE2a interacted with OsRLCK185 at plasma membrane. The conserved cysteine residues in CIAPIN1 domain of OsDRE2a were essential for tight interaction of OsRLCK185. OsDRE2a was phosphorylated by OsRLCK185. The expression of OsDRE2a and OsDRE2b was induced after chitin treatment. Reduction of OsDRE2a and OsDRE2b mRNA levels by RNA interference resulted in the decreased chitin-induced ROS production. Thus, it is likely that OsDRE2 regulates OsRLCK185-mediated immune responses.
Graphical Abstract
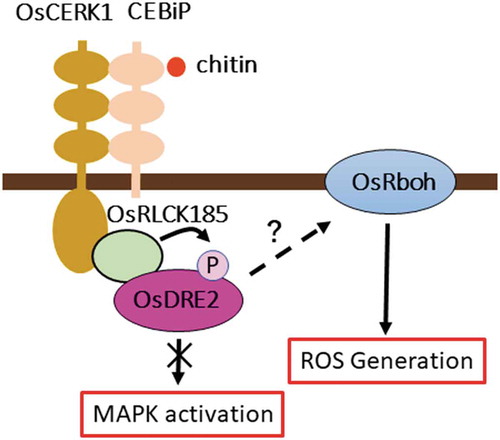
OsDRE2 interacts with OsRLCK185 at plasma membrane and is phosphorylated by OsRLCK185. Silencing of OsDRE2 causes the reduction of chitin-induced ROS production.
KEYWORDS:
The plant immune system is generally initiated by pattern recognition receptors (PRRs) [Citation1]. PRRs recognize conserved microbe-associated molecular patterns (MAMPs) including bacterial flagellin, elongation factor-Tu, peptidoglycan, and fungal chitin. PRRs are plasma membrane (PM)-localized receptor-like kinases (RLKs) or receptor-like proteins (RLPs) [Citation2]. These PRRs form the complexes with other regulatory RLKs in response to MAMPs. This formation initiates a series of immune responses including the production of antimicrobial compounds and callose deposition through the early activation of mitogen-activated protein kinases (MAPKs), production of reactive oxygen species (ROS), and changes in ion fluxes [Citation3]. The rice RLK, OsCERK1 and the RLP, CEBiP, which recognize the fungal cell wall component chitin, are the PRRs in rice that has been examined in the most detail [Citation4,Citation5]. These PRRs possess the extracellular lysin-motif domains that recognize chitin. CEBiP forms a homodimer upon chitin binding that is followed by heterodimerization with OsCERK1, creating a signaling-active sandwich-type receptor complex [Citation6]. The CEBiP/OsCERK1 complex triggers rice immune responses to overcome fungal invasion. We found that rice receptor-like cytoplasmic kinase 185 (OsRLCK185) directly interacts with OsCERK1 and is phosphorylated by OsCERK1 in a chitin-dependent manner [Citation7,Citation8]. Silencing of OsRLCK185 reduces ROS production and MAPK activation induced by chitin perception. OsRLCK185 interacts with and phosphorylates the OsMAPKKK18 and OsMAPKKK24 which are upstream components of the MAPK cascade and regulates chitin-induce MAPK activation [Citation9,Citation10]. In Arabidopsis, BIK1, a member of the RLCK family phosphorylates the N-terminal region of the NADPH oxidase RbohD (respiratory burst oxidase homolog D) in response to MAMPs perception, which rapidly induces ROS production [Citation11,Citation12]. A recent study reported that OsRLCK118 regulates the rice RLK, SDS2-mediated ROS production though direct phosphorylation of the NADPH oxidase OsRbohB [Citation13]. However, how ROS are produced in response to MAMPs in rice remains to be identified.
Iron-sulfur (Fe-S) clusters are the most ancient and versatile metal cofactors. Fe-S clusters are used by a large and diverse group of proteins, serving both structural and catalytic roles [Citation14]. Fe-S clusters function in central metabolic processes such as electron transfer, redox chemistry, enzyme catalysis, and sensing environmental or intracellular conditions to regulate gene expression. In eukaryotes, the maturation of cellular Fe–S proteins is initiated by the mitochondrial ISC (iron–sulfur cluster) assembly machinery [Citation15]. Cytosolic and nuclear Fe–S protein biogenesis additionally depends on the cytosolic Fe–S protein assembly (CIA) machinery. The components of CIA machinery is highly conserved among animal and plant species [Citation16]. The required electrons are initially delivered from NADPH, and then channeled through the FAD and FMN cofactors of the NADPH-dependent diflavinreductase Tah18 to the Fe-S protein Dre2. Dre2 is the yeast counterpart of human Anamorsin (or CIAPIN1, cytokine-induced apoptosis inducer 1) [Citation17]. Dre2 is essential for yeast cell viability. Yeast Dre2 contains two Fe-S clusters, a [2Fe-2S] and [4Fe-4S], and is implicated in cytosolic Fe-S cluster synthesis [Citation18]. Dre2 possesses an N-terminal SAM methyltransferase-like domain, and CIAPIN1 domain at its C terminus. The CIAPIN1 domain contains two cysteine motifs (CX2CXC and twin CX2C). The seven conserved cysteine residues are crucial for Fe–S cluster binding in vivo and are found to be essential for cell growth, as well as functionality within the CIA machinery [Citation19].
We identified OsDRE2a, rice homologs of yeast Dre2, as interactors of OsRLCK185 by yeast two hybrid screening. OsDRE2a was mainly localized in cytosol. However, OsDRE2a interacted with OsRLCK185 at plasma membrane. Furthermore, OsDRE2a was specifically phosphorylated by OsRLCK185. The knockdown of OsDRE2a and OsDRE2b reduced chitin-induced ROS production. Thus, it is likely that the OsDRE2a and OsDRE2b play a positive role in the regulation of rice immunity initiated by the OsCERK1/OsRLCK185 complex.
Materials and methods
Plasmid constructs
The coding region of OsDRE2a was amplified by PCR using the specific primer sets (Table S1). The amplified fragment was subcloned into pENTR/D-TOPO (Invitrogen). Point mutations in OsDRE2a were generated by PCR-mediated substitution of the sequences encoding the corresponding amino acid residues. For RNAi vectors, the coding region of OsDRE2 was amplified, cloned into pENTR/D-TOPO, and transferred into pANDA RNAi vector to create transgenic RNAi plants [Citation20].
Yeast two hybrid assays
The coding regions of OsDRE2a and OsRLCK185 were transferred into pBTM116-GW and pVP16-GW, respectively, by the Gateway system using an LR clonase reaction. The resultant vectors were introduced into cells of Saccharomyces cerevisiae L40 (MATatrp1 leu2 his3 LYS2::lexA-HIS3 URA3::lexA-lacZ). Transformants were selected on minimal medium lacking tryptophan and leucine. The yeast two-hybrid interaction was analyzed based on the requirement of histidine for yeast growth, as previously described [Citation9].
Rice transformation
Calli generated from embryos of rice cultivar Nipponbare were transformed using Agrobacterium tumefaciens EHA101 as described previously [Citation21]. Suspension-cultured cells were also prepared from transformed calli.
Gene expression
Rice cultured cells were treated with 2 µg/mL chitin ((GlcNAc)7) for 3, and 6 h. Total RNA was isolated from chitin-treated rice cultured cells using TRIzol reagent (Invitrogen) and treated with DNase I (Roche). First-strand cDNAs synthesized from 1 µg total RNA were analyzed by quantitative RT-PCR using a Step-One Plus Real-Time PCR system (Applied Biosystems), and normalized against a ubiquitin reference gene. Three biological replicates were used for each experiment, and two quantitative replicates were performed for each biological replicate.
Transient assays using protoplasts
Protoplasts were isolated from cultured rice cells as previously described [Citation7]. Plasmid transformation was performed using the polyethylene glycol (PEG) method. 100 μL aliquots of protoplasts (2.5 × 106 cells/mL) were transformed by mixing with 5 μg of plasmid DNA. For localization analysis and BiFC assays, transfected protoplasts were observed using BIOREVO BZ-9000 fluorescence microscope (KEYENCE).
MAPK assays
For MAP kinase activation assay, rice cultured cells were subcultured for 3 days in a fresh medium. Rice cultured cells were treated with 2 µg/mL chitin ((GlcNAc)7). Proteins were extracted from cells in extraction buffer (50 mM HEPES [pH 7.4], 50 mM β-glycerophosphate, 5 mM EGTA, 5 mM EDTA, 10 mM NaF, 10 mM Na3VO4, 2 mM DTT, and protease inhibitor cocktail [Roche]). The phosphorylation of MAPK proteins was detected by immune blotting with an anti-pMAPK antibody (1:2,000 dilution; Cell Signaling).
Measurement of ROS production
For measurement of ROS, rice cultured cells were subcultured for 3 days in a fresh medium. The medium was removed and replaced by a fresh medium containing 0.5 mM L-012 (Wako) and 10 µg/mL horseradish peroxidase (TOYOBO). Rice cultured cells were treated with 2µg/mL chitin ((GlcNAc)7) and ROS production was quantified using a Mithras LB940 Multimode Microplate Reader (Berthold Technologies).
In vitro phosphorylation assay
The recombinant proteins were expressed using a Cold-Shock bacterial expression system (TAKARA). GST-fused OsDRE2a, OsRLCK185 and OsRLCK185K108E were purified using Glutathione Sepharose 4B (GE Healthcare) and eluted using 50 mM glutathione. OsCERK1-IC was cloned into the pMAL-c2 vector (New England Biolabs). E. coli origami cells were used for recombinant protein expression. MBP- OsCERK1-IC expression was induced with 0.5 mM IPTG at 25°C for 3 h. MBP-OsCERK1-IC was purified by amylose resin (New England Biolab). The kinase activity assay was performed in 40 μL of a reaction mixture containing 50 mM HEPES (pH7.6), 10 mM MgCl2, 100 µM ATP, 1 mM DTT, 1 μg kinase and 1 μg substrate. The assay was initiated by adding 0.4 µL (4 μCi) [32P] γ-ATP, and the reaction mixture was incubated at 25°C for 1 h. The reaction was terminated by the addition of 6xSDS sample buffer and subsequent incubation at room temperature for 10 min. Samples were separated by SDS-polyacrylamide gel electrophoresis. The 32P-labeled bands were detected using an FLA-7000 imaging analyzer (Fuji Film) and Multi Gauge version 3.0 software (Fuji Film).
Results
Identification of OsDRE2 as an OsRLCK185 interactor
We previously showed that OsRLCK185 plays crucial roles in chitin-induced defense signaling in rice. OsRLCK185 functions as a downstream component of OsCERK1 in the chitin signaling pathway and regulates chitin-activated ROS production and MAPK activation [Citation7]. To identify other components that participate in the OsRLCK185-mediated intracellular signaling pathway, we screened the proteins that interacted with OsRLCK185 by a yeast two-hybrid method using a rice cDNA library prepared from rice suspension cells treated with chitin. We identified the rice protein Os03g0182800 and Os04g0674400 as an interactor for OsRLCK185 (Table S1). Os03g0182800 encodes a transcription factor EBP-89 which is predicted to localize in nucleus [Citation22]. Since the localization of EBP-89 was not coincident with that of OsRLCK185, EBP-89 was excluded from the candidate interactors. Os04g0674400 encodes the ortholog of yeast Dre2 and human Anamorsin, which are known to be involved in Fe-S cluster biogenesis and apoptosis during hematopoiesis.
The amino acid similarity between rice and yeast proteins was analyzed by ClustalW alignment ()). The OsDRE2a protein has a SAM methyltransferase like domain at N terminus and CIAPIN1 domain at C terminus. The CIAPIN domain in OsDRE2a contains seven cysteine residues, which are conserved in two motifs, CX2CXC and twin CX2C. These cysteine residues are conserved in eukaryotes [Citation19]. The putative methyltransferase and CIAPIN1 domains of OsDRE2a had 12.5% and 42.9% identities at the amino acid levels, respectively, to yeast Dre2.
Figure 1. OsDRE2a interacts with OsRLCK185 at plasma membrane. a. Comparison of amino acid sequences between OsDRE2a and yeast Dre2.The sequence of yeast Dre2 (GeneBank accession number A7A031) was aligned with the OsDRE2a using ClustalW (http://www.ddbj.nig.ac.jp). Identical amino acid residues are indicated by black box. Shaded boxes indicate SAM methyltransferase like domain and CIAPIN1 domain, respectively. The asterisks indicate conserved cysteine residues in CIAPIN1 domain. b. OsDRE2a interacts with OsRLCK185 in yeast two-hybrid experiments. The growth of yeast colonies on plates (-ULWH) lacking uracil (U), leucine (L), tryptophan (W), and histidine (H) with 0.1 mM 3-aminotriazole (3-AT) indicates a positive interaction. c. Visualization of the interaction between OsDRE2a and OsRLCK185K108E by BiFC analysis in rice protoplasts. GUS was also used as negative control. Venus fluorescence indicates interaction between OsDRE2a and OsRLCK185K108E. Scale bar = 10µm.
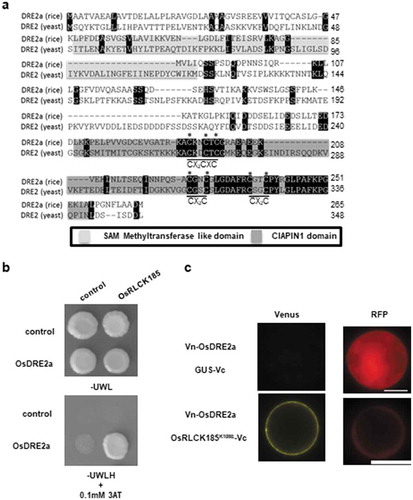
The yeast two hybrid experiments showed that OsDRE2a interacted with OsRLCK185 ()). To analyze the in vivo interaction between OsRLCK185 and OsDRE2a, we performed bimolecular fluorescence complementation (BiFC) assays using transient expression in rice protoplasts. Since the fluorescence for OsRLCK185-GFP was not detectable in rice protoplasts as previously described [Citation7], we used the kinase-inactive mutant OsRLCK185K108E, which was produced by substitution of lysine at the position 108 to glutamate. OsRLCK185K108E tagged with the C-terminal domain (aa 155–238) of Venus (OsRLCK185 K108E -Vc) and OsDRE2a tagged with the N-terminal domain (aa 1–154) (Vn-OsDRE2a) were co-expressed in rice protoplasts with the control fluorescent protein RFP. Venus fluorescence was detected at the plasma membrane (PM) ()). Thus, it is likely that OsRLCK185 and OsDRE2a interact with each other at the PM.
OsDRE2a interacted with OsRLCK185 though the CIAPIN1 domain
To further characterize the interaction between OsDRE2a and OsRLCK185, yeast two-hybrid assays were carried out using N-terminal domain (1–132) and C-terminal domain (133–265) of OsDRE2a. OsRLCK185 interacted with C-terminal domain of OsDRE2a containing the CIAPIN1 domain, but not N-terminal domain containing the SAM methyltransferase like domain ()). To test whether cysteine residues in two motifs of CIAPIN1 domain were essential for the interaction between OsRLCK185 and OsDRE2a, we introduced point mutation into OsDRE2a by substitution of cysteine at two conserved motifs with alanine. OsRLCK185 interacted with OsDRE2aC195A/C198A/C200A ()). However, OsDRE2aC226A/C229A did not interact with OsRLCK185 ()). These results indicated that the cysteine residues in the twin CX2C motif are essential for the interaction with OsRLCK185.
Figure 2. Conserved cysteine residues in the CIAPIN1 domain are important for the interaction between OsDRE2a and OsRLCK185. a. Schematic structures of full-length OsDRE2a (1-266aa), its N-terminus (1–132), and its C-terminus (133–266). Shaded boxes indicate SAM methyltransferase like domain and CIAPIN1 domain, respectively. The numbers indicate the positions of amino acid sequences. b. OsRLCK185 interacts with C-terminus of OsDRE2a. The full length OsDRE2a (1-266aa) was divided into N-terminus (1–132) and C-terminus (133–266). N- terminus contain the SAM methyltransferase like domain. C- terminus contains the CIAPIN1 domain. The growth of yeast colonies on plates (-ULWH) lacking uracil (U), leucine (L), tryptophan (W), and histidine (H) indicated a positive interaction. c. OsRLCK185 didn’t interact with OsDRE2aC226A/C229A in yeast two-hybrid experiment. The growth of yeast colonies on plates (-ULWH) lacking uracil (U), leucine (L), tryptophan (W), and histidine (H) indicated a positive interaction.
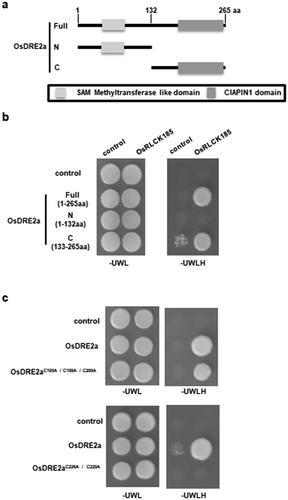
OsRLCK185 phosphorylates OsDRE2a in vitro
Recently, we and another group reported that the OsRLCK185 phosphorylates its downstream components, OsMAPKKK18 and OsMAPKKK24, which triggers activation of immune signaling [Citation9,Citation10]. If OsDRE2a is a downstream component of OsRLCK185, it is possible that OsRLCK185 may phosphorylate OsDRE2a. We purified recombinant proteins of OsRLCK185 and OsDRE2a using a protein expression system in E. coli. The in vitro kinase assay showed that OsDRE2a was phosphorylated by OsRLCK185 ()). Since OsRLCK185 forms a complex with both OsCERK1 and OsDRE2a at the plasma membrane, OsCERK1 may directly phosphorylates OsDRE2a. Therefore, we tested whether OsCERK1 phosphorylates OsDRE2 in vitro. The in vitro kinase assay indicates that OsCERK1 strongly phosphorylated the kinase-inactive mutant OsRLCK185K108E but not OsDRE2a ()). Thus, it is likely that OsDRE2a is specifically phosphorylated by OsRLCK185.
Figure 3. OsDRE2a is phosphorylated by OsRCLK185 but not OsCERK1. a. OsRLCK185 phosphorylates OsDRE2a in vitro. The in vitro phosphorylation reaction was performed using [32P]γ-ATP, and phosphorylated proteins were detected by autoradiography. CBB, Coomassie brilliant blue. b. OsCERK1 does not phosphorylate OsDRE2a in vitro. The in vitro phosphorylation reaction was performed with a recombinant of the intracellular domain of OsCERK1, kinase inactive mutant OsRLCK185K108E and OsDRE2a. The protein loading control was shown by staining with Coomassie Brilliant Blue. The in vitro phosphorylation reaction was performed using [32P]γ-ATP, and phosphorylated proteins were detected by autoradiography.
![Figure 3. OsDRE2a is phosphorylated by OsRCLK185 but not OsCERK1. a. OsRLCK185 phosphorylates OsDRE2a in vitro. The in vitro phosphorylation reaction was performed using [32P]γ-ATP, and phosphorylated proteins were detected by autoradiography. CBB, Coomassie brilliant blue. b. OsCERK1 does not phosphorylate OsDRE2a in vitro. The in vitro phosphorylation reaction was performed with a recombinant of the intracellular domain of OsCERK1, kinase inactive mutant OsRLCK185K108E and OsDRE2a. The protein loading control was shown by staining with Coomassie Brilliant Blue. The in vitro phosphorylation reaction was performed using [32P]γ-ATP, and phosphorylated proteins were detected by autoradiography.](/cms/asset/e4c14c13-97e4-4ba1-8841-323357bfbc50/tbbb_a_1543012_f0003_oc.jpg)
Expression and subcellular localization of OsDRE2a and OsDRE2b
We found an additional OsDRE2a homologous gene (Os04g0682050) in the rice genome by a BLAST database search, which was named OsDRE2b. OsDRE2a and OsDRE2b shared 98% identity at the amino acid level ()). OsDRE2b also possessed a SAM methyltransferase-like domain and CIAPIN1 domain, which are conserved in yeast Dre2. To examine the subcellular localization of OsDRE2a and OsDRE2b in rice cells, we fused green fluorescent protein (GFP) to the C termini of OsDRE2a and OsDRE2b and transiently expressed these constructs in rice protoplasts together with the control fluorescence protein RFP. OsDRE2a and OsDRE2b were both mainly detected at the cytoplasm ()). To analyze the involvement of OsDRE2a and OsDRE2b in chitin-induced immunity, we analyzed the expression patterns of OsDRE2a and OsDRE2b under chitin treatment. Expression of OsDRE2a and OsDRE2b was significantly induced by chitin in rice suspension cells ()), whereas the induced level of OsDRE2a mRNA was much higher than that of OsDRE2b.
Figure 4. Two kinds of OsDRE2 proteins were conserved in rice and the expression of these genes was induced by chitin. a. Comparison of amino acid sequences between OsDRE2a and OsDRE2b.The sequence of OsDRE2b was aligned with that of OsDRE2a using ClustalW. Identical amino acid residues are indicated by black box. b. Subcellular localization of OsDRE2a and OsDRE2b. OsDRE2a-GFP and OsDRE2b-GFP were expressed under the control of 35S promoter in rice protoplasts. Scale bar = 10µm. c. Chitin-induced expression of OsDRE2a and OsDRE2b. The expression of OsDRE2a and OsDRE2b was analyzed by real time RT-PCR using specific primers. Ubiquitin (Ubq) was used as an internal control. Data are means and SDs. The asterisks indicate significant differences from the normal condition (0h) controls by Student’s t-test (*P < 0.01).
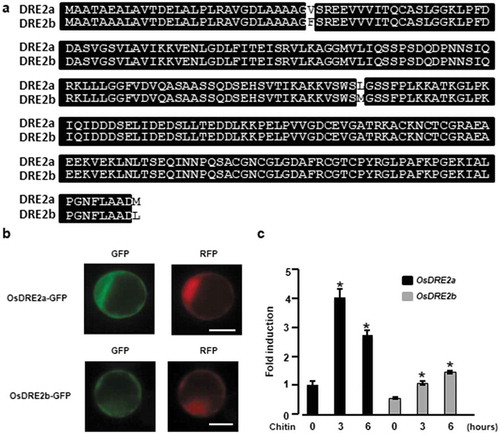
OsDRE2a and OsDRE2b play a role in rice immunity
To analyze whether OsDRE2a and OsDRE2b are involved in chitin response, we generated two transgenic rice suspension cells carrying an OsDRE2a and OsDRE2b RNAi interference (RNAi) construct. These RNAi cell lines were analyzed for silencing of OsDRE2a and OsDRE2b by quantitative RT-PCR ()). Significant reductions in the transcript levels of OsDRE2a and OsDRE2b were observed in both RNAi lines. We treated these RNAi cell lines with chitin. The ROS production induced by chitin was measured by chemiluminescence mediated by L-012, a reagent to detect ROS ()). The ROS production was significantly reduced in both RNAi cell lines as compared with wild type. However, similar levels of chitin-induced MAP kinase activation were detected in wild type and the RNAi cell lines ()).
Figure 5. OsDRE2a and OsDRE2b positively regulate ROS burst. a. Transcript levels of OsDRE2a and OsDRE2b in the OsDRE2a/b RNA interference (RNAi) suspension cells under normal growth condition were determined by quantitative real-time PCR using specific primers. Ubiquitin (Ubq) was used as an internal control. Data are means and SDs. The asterisks indicate significant differences from the wild-type (WT) controls by Student’s t-test (P < 0.01). b. Production of ROS in OsDRE2a/b RNAi cells after chitin treatment. Rice suspension cells were treated with 2 μg/mL chitin in a solution containing 500 μM L-012 and 10 μg/mL horseradish peroxidase. Total relative light units (RLU) during 50 min of treatment are presented to indicate the ROS production. Data are means and SEs (n = 9; Chitin treatment, N = 3; Mock treatment). The asterisks indicate significant differences from the wild-type (WT) controls by Student’s t-test (*P < 0.01, **< 0.05). c. Chitin -induced activation of mitogen-activated protein kinase (MAPK) in OsDRE2a/b RNAi cells. Total protein was extracted from rice suspention cells treated with 2 μg/mL chitin. The activation of MAPK was analyzed by immunoblot with anti-pMAPK antibody. The protein loading control was shown by Ponceau staining. Experiments were repeated at least three times with similar results.
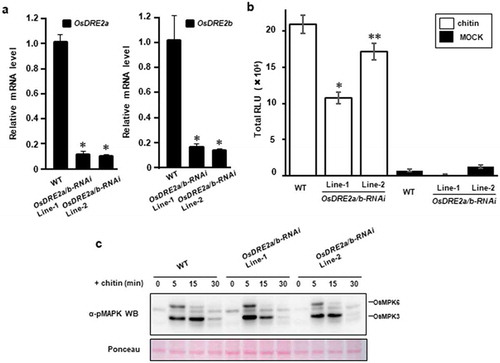
Discussion
OsRLCK185 is a key regulator in chitin- and PGN- mediated signaling in rice [Citation7]. In the present study, we identified the rice protein, OsDRE2a as a possible interactor which may link the OsRLCK185 with downstream components. OsDRE2a interacted with OsRLCK185 at plasma membrane and was phosphorylated by OsRLCK185 in vitro. The expression of OsDRE2a and OsDRE2b was induced by chitin. The OsDRE2a/b RNAi cells showed the reduction of chitin-induced ROS production. These results indicate that OsDRE2 may play a role in OsRLCK185-mediated rice immunity.
In a transient expression system using rice protoplasts, we observed the florescence of GFP-fused OsDRE2a in cytosol ()), which coincided with the observation that DRE2 protein in Arabidopsis was localized in cytosol [Citation23]. Thus, it is likely that OsDRE2a and OsDRE2b are involved in the Fe-S cluster assembly in cytosol in the same ways as other eukaryotes. However, we found that OsDRE2a interacted with OsRLCK185 at plasma membrane ()). These results suggest that OsDRE2a may have an additional function besides the role in Fe-S cluster assembly.
The cysteine residues of the CIAPIN1 domain (CX2CXC and twin CX2C) in rice and yeast proteins were perfectly aligned ()). In yeast, the seven cysteine residues of CIAPIN1 domain in Dre2 are likely candidate sites to coordinate two Fe-S clusters of Dre2 [Citation17]. In addition, the C-terminus of Dre2 is essential for cell survival while the N-terminus of Dre2 is dispensable for survival [Citation18]. On the other hand, the twin CX2C motif of OsDRE2a is required for the interaction with OsRLCK185 ()). Thus, it seems that twin CX2C motif of OsDRE2a functions as a protein-protein interaction domain.
To examine the role of OsDRE2a and OsDRE2b in rice immunity, we attempted to generate knockdown plants of OsDRE2a and OsDRE2b. However, we failed to regenerate transgenic rice plant. Consistent with the result, the knockout mutation of Arabidopsis DRE2 resulted in embryonic lethality [Citation24]. A conditional silencing system will be required to further address their role during pathogen infections.
The phosphorylation of DRE2 protein has not been reported in another organism so far. This is the first report about post-translational modification of DRE2 protein. However, how the phosphorylation affects protein activity and function of OsDRE2a remains unknown. The analysis using phosphomimic OsDRE2a mutant may reveal OsRLCK185-mediated regulation of OsDRE2a in rice immunity.
OsRLCK185 positively regulates MAPK activation and ROS production in chitin signaling. We and another group showed that the chitin-induced MAPK activation is operated through the direct phosphorylation of OsMAPKKK18 and OsMAPKKK24 by OsRLCK185 [Citation9,Citation10]. However how OsRLCK185 regulates ROS production remains unknown. In Arabidopsis, BIK1 RLCK activates AtRbohD by the phosphorylation of its N-terminal regulatory region [Citation11,Citation12]. Therefore, OsRLCK185 may regulate rice Rboh activity in a similar manner to BIK1. In this scenario, OsDRE2a may be involved in OsRLCK185-mediated activation of rice Rbohs. Alternatively, it is also possible that OsDRE2 may control ROS levels independent of Rboh by activating other ROS-generating enzymes and/or by inhibiting ROS scavengers, because OsDRE2 positively regulates ROS levels. Further study will be required to understand molecular mechanism by which OsDRE2 functions as a positive regulator of ROS accumulation.
Author contributions
K.Y., and T.K. designed the project, and wrote the manuscript. K.Y., and Y.Y. performed experiments, and S.N., H.M., and S.Y. analyzed data. All authors discussed the results and commented on the manuscript.
Acknowledgments
We thank Tamo Fukamizo for providing chitin. We also thank Tamon Nishinaka and Yuta Kusumoto for their technical assistance. We thank Tsuyoshi Nakagawa of Shimane University for providing the pGWB vectors.
Disclosure statement
No potential conflict of interest was reported by the authors.
Supplemental material
Supplemental data for this article can be accessed here.
Additional information
Funding
References
- Dangl JL, Horvath DM, Staskawicz BJ. Pivoting the plant immune system from dissection to deployment. Science (New York, NY). 2013;341(6147):746–751.
- Couto D, Zipfel C. Regulation of pattern recognition receptor signalling in plants. Nat Rev Immunol. 2016;16(9):537–552.
- Peng Y, van Wersch R, Zhang Y. Convergent and divergent signaling in PAMP-triggered immunity and effector-triggered immunity. Mol Plant Microbe Interact. 2018;31(4):403–409.
- Kaku H, Nishizawa Y, Ishii-Minami N, et al. Plant cells recognize chitin fragments for defense signaling through a plasma membrane receptor. Proc Natl Acad Sci U S A. 2006;103(29):11086–11091.
- Shimizu T, Nakano T, Takamizawa D, et al. Two LysM receptor molecules, CEBiP and OsCERK1, cooperatively regulate chitin elicitor signaling in rice. Plant J. 2010;64(2):204–214.
- Hayafune M, Berisio R, Marchetti R, et al. Chitin-induced activation of immune signaling by the rice receptor CEBiP relies on a unique sandwich-type dimerization. Proc Natl Acad Sci U S A. 2014;111(3):E404–13.
- Yamaguchi K, Yamada K, Ishikawa K, et al. A receptor-like cytoplasmic kinase targeted by a plant pathogen effector is directly phosphorylated by the chitin receptor and mediates rice immunity. Cell Host Microbe. 2013;13(3):347–357.
- Yamaguchi K, Yamada K, Kawasaki T. Receptor-like cytoplasmic kinases are pivotal components in pattern recognition receptor-mediated signaling in plant immunity. Plant Signal Behav. 2014;8(10):e25662.
- Kawasaki T, Yamada K, Yoshimura S, et al. Chitin receptor-mediated activation of MAP kinases and ROS production in rice and Arabidopsis. Plant Signal Behav. 2017;12(9):e1361076.
- Yamada K, Yamaguchi K, Yoshimura S, et al. Conservation of chitin-induced MAPK signaling pathways in rice and arabidopsis. Plant Cell Physiol. 2017;58(6):993–1002.
- Kadota Y, Sklenar J, Derbyshire P, et al. Direct regulation of the NADPH oxidase RBOHD by the PRR-associated kinase BIK1 during plant immunity. Mol Cell. 2014;54(1):43–55.
- Li L, Li M, Yu L, et al. The FLS2-associated kinase BIK1 directly phosphorylates the NADPH oxidase RbohD to control plant immunity. Cell Host Microbe. 2014;15(3):329–338.
- Fan J, Bai P, Ning Y, et al. The monocot-specific receptor-like kinase SDS2 controls cell death and immunity in rice. Cell Host Microbe. 2018;23(4):498–510 e495.
- Lill R. Function and biogenesis of iron-sulphur proteins. Nature. 2009;460(7257):831–838.
- Pena-Diaz P, Lukes J. Fe-S cluster assembly in the supergroup Excavata. J Biol Inorg Chem. 2018;23(4):521–541.
- Balk J, Pilon M. Ancient and essential: the assembly of iron-sulfur clusters in plants. Trends Plant Sci. 2011;16(4):218–226.
- Shibayama H, Takai E, Matsumura I, et al. Identification of a cytokine-induced antiapoptotic molecule anamorsin essential for definitive hematopoiesis. J Exp Med. 2004;199(4):581–592.
- Zhang Y, Lyver ER, Nakamaru-Ogiso E, et al. Dre2, a conserved eukaryotic Fe/S cluster protein, functions in cytosolic Fe/S protein biogenesis. Mol Cell Biol. 2008;28(18):5569–5582.
- Netz DJ, Genau HM, Weiler BD, et al. The conserved protein Dre2 uses essential [2Fe-2S] and [4Fe-4S] clusters for its function in cytosolic iron-sulfur protein assembly. Biochem J. 2016;473(14):2073–2085.
- Miki D, Shimamoto K. Simple RNAi vectors for stable and transient suppression of gene function in rice. Plant Cell Physiol. 2004;45(4):490–495.
- Hiei Y, Ohta S, Komari T, et al. Efficient transformation of rice (Oryza sativa L.) mediated by Agrobacterium and sequence analysis of the boundaries of the T-DNA. Plant J. 1994;6(2):271–282.
- Yang HJ, Shen H, Chen L, et al. The OsEBP-89 gene of rice encodes a putative EREBP transcription factor and is temporally expressed in developing endosperm and intercalary meristem. Plant mol biol. 2002;50(3):379–391.
- Buzas DM, Nakamura M, Kinoshita T. Epigenetic role for the conserved Fe-S cluster biogenesis protein AtDRE2 in Arabidopsis thaliana. Proc Natl Acad Sci U S A. 2014;111(37):13565–13570.
- Couturier J, Touraine B, Briat JF, et al. The iron-sulfur cluster assembly machineries in plants: current knowledge and open questions. Front Plant Sci. 2013;4:259.