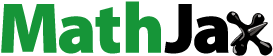
ABSTRACT
The release profile of d-limonene and ethyl hexanoate was investigated using a dynamic vapor sorption (DVS) system coupled with gas chromatography. The flavors were encapsulated by spray drying using Saccharomyces cerevisiae cells from which β-glucan had been partially extracted. Relative humidity (RH) was stepped from 20% to 50, 60, 70, and 80% at 30, 40, 50, and 60ºC. The maximum release flux for d-limonene and ethyl hexanoate was around 12 and 28 mg/s∙m2∙g-powder at 80% RH and 60ºC incubation. The Weibull distribution function was well fitted with the experimental data to analyze release kinetics. The release mechanism parameter was greater than 1.0, which indicates a controlled release with initial induction time. The activation energy for ethyl hexanoate (6 kJ/mol) was lower than d-limonene (41 kJ/mol) at 80% RH, which indicates higher affinition of ethyl hexanoate to migrate from the lipid bilayer membrane towards the water phase.
Graphical Abstract
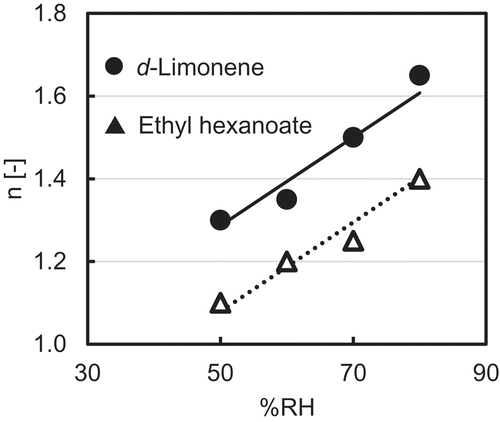
Relation of release mechanism number as a function of RH
The flavor compounds in any type of food directly influence taste perception in the human or animal brain. Flavor, the multisensory integration, reaches the olfactory bud via two pathways: (a) orthonasal (during sniffing) and (b) retronasal (during mastication). According to Chartier [Citation1], around 80–90% of the taste of food comes from the nose. Sweetness and saltiness are also induced by the flavor of food [Citation2]. Numerous studies have discussed the positive correlation between smell perception and taste perception [Citation3–Citation7]. The importance of volatile flavor compounds in foods is recognized. Therefore, product development in terms of using different flavors in processed foods is a continual process in the food industries all over the world. At the same time, the loss of added flavor during different processing steps due to high volatility and poor stability of those compounds cannot be ignored. To overcome these challenges, encapsulation has been used in the food industry since the 1930s, and research has been conducted since then to develop this technique. Carbohydrates (modified starch, maltodextrin, cyclodextrin) and proteins (whey protein, soy protein) are the most usable wall materials to encapsulate flavor components [Citation8,Citation9]. However, other biopolymers (chitosan, alginate, gelatin) [Citation10,Citation11], lipid bilayer (liposome) [Citation12,Citation13], or whole cells (yeast cells) [Citation14,Citation15] are also being used to protect flavorings from environmental shock.
The selection of wall material depends on several factors: nature of core material, desired product objectives, economics, process of encapsulation, and approval by the Food and Drug Administration (US) or European Food Safety Authority [Citation16]. Saccharomyces cerevisiae cell, a natural and nutritious encapsulant, fulfills the above criteria and successfully encapsulate different types of active compound such as fish oil [Citation17], flavor [Citation18,Citation19], curcumin [Citation20,Citation21], photosynthetic resveratrol [Citation22], and water soluble antioxidant [Citation23]. Using yeast cells for flavor encapsulation probably started in the 1970s, when it was first used to encapsulate water soluble flavor [Citation24]. Encapsulation efficiency depends on hydrophobicity and several other factors, but the encapsulation process was gradually modified to encapsulate all types of flavor. Additionally, the yeast cell itself is a thermostable encapsulant, from which flavor is released only above a very high temperature of 243ºC [Citation25]. The cell wall components also provide some extra benefits: mannoprotein improves thermal stability [Citation26], and beta-glucan and mannan have antioxidant properties [Citation27,Citation28].
The advantages of using yeast cells for flavor encapsulation was overviewed by Pham-Hoang et al. [Citation29] and Paramera et al. [Citation30]. Active compounds from a capsule should be released in a controlled manner, which is one of the prerequisite criteria for a successful encapsulation technique. Besides the purpose of storage, controlled release of core material has several advantages. According to Pothakamury & Barbosa-Cánovas [Citation31], the benefits of controlled release are (a) controlled rate of release of active materials over a prolonged time, (b) reduced loss of ingredients (vitamins, minerals, etc.) during processing, and (c) the possibility of separating reactive components. However, several factors govern the release of active material from wall material.
Research has been carried out to investigate the factors related to the release of active/core material from wall material [Citation32–Citation35]. Furuta et al. [Citation36] found that both the composition of carrier material and relative humidity are responsible for different types of release behavior of core material. Yoshii et al. [Citation37] found a relationship between release of encapsulated ethyl butyrate from spray-dried maltodextrin/gum arabic or soy matrices and storage relative humidity. Storage relative humidity is usually studied experimentally with a static method by using a saturated salt solution in a desiccator to investigate the flavor release profile [Citation38,Citation39]. However, this static process takes a long time to conduct a whole set of experiments. Additionally, samples need to be taken out many times from a storage container, which may result in fluctuations in temperature, relative humidity, and moisture gain, or loss of the remaining samples. Consequently, this traditional method increases the chance of obtaining an inaccurate release profile, which is the major limitation of this method.
The dynamic vapor sorption (DVS) technique overcomes these obstacles to provide a release profile of encapsulated flavor as a function of relative humidity. DVS uses a temperature-controlled incubator where relative humidity is generated by mixing dry and saturated gas flow for which an accurate proportion is controlled by using a mass flow controller [Citation40]. Numerous studies have been carried out to rapidly analyze volatile release using DVS instruments along with atmospheric chemical ionization mass spectrometry or a gas chromatography flame ionization detection method [Citation41,Citation42]. Normand et al. [Citation25] observed that not only temperature but also relative humidity triggers flavor release from yeast cells. They observed the release behavior of d-limonene using a DVS system by monitoring the weight change of empty yeast cells. To the best of our knowledge, no study had been done to investigate the flavor release profile from encapsulated spray-dried yeast cells using DVS–gas chromatography, which precisely identifies the peak of individual compounds.
The purpose of this study was to analyze the real-time release profile of encapsulated d-limonene (orange-flavored compound) and ethyl hexanoate (pineapple-flavored compound) from spray-dried Saccharomyces cerevisiae cells (β-glucan partially extracted) using a home-built DVS coupled with GC–FID. d-Limonene (very hydrophobic compound, log P = 4.8) and ethyl hexanoate (comparatively less hydrophobic compound, log P = 2.8) were used as model flavors. Another objective of this study was to establish a kinetic model during flavor release from yeast cells.
Materials and methods
Materials
Saccharomyces cerevisiae cells were kindly gifted from Fuji Foods Corporation (Yokohama, Japan). The obtained yeasts were initially drum-dried (moisture content ~5%) cells with β-glucan partially extracted. The flavor compounds d-limonene and ethyl hexanoate were purchased from Wako Pure Chemical Industries Ltd. (Osaka, Japan).
Methods
Preparation of encapsulated flavor powder with yeast cells (Saccharomyces cerevisiae)
The two flavor compounds (d-limonene and ethyl hexanoate) were encapsulated in Saccharomyces cerevisiae cells (β-glucan partially extracted), and the encapsulated cells were then spray dried following the method described in our previous study [Citation15]. All the operating conditions remained the same with the procedure.
Analysis of flavor release flux from the encapsulated powder using the DVS–GC method
The rapid flavor release flux of the flavor encapsulated yeast powder was measured using a home-built DVS system, which was connected to a gas chromatography–flame ionized detector. The procedure followed the method proposed by Yamamoto et al. [Citation43]. The general principle of this DVS system was to produce a humidification condition by producing vapor saturation through bubbling of nitrogen gas in distilled water in a 500 mL bottle. The nitrogen flow was 100 mL/min controlled by a flow control unit (MC-10A; Kofloc, Kyoto, Japan). The bubbling bottle was placed in a water bath in which the heat was supplied by a waterproof heater with a temperature controller (DSSP93; Shimaden, Tokyo, Japan). Around 100 mg encapsulated powder was placed in a sample holder plate (13 mm i.d. × 1 mm in height) and tightly packed by pressing the powder with a thin spatula. The sample plate was positioned in a low temperature incubator (IJ 300 W; Yamato Scientific Co., Ltd., Tokyo, Japan) using a glass vial (16 mm i.d. × 80 mm in height) in which an isothermal condition was maintained. The released flavor from the sample through moisture sorption was collected from the headspace and automatically sampled every 5 min into a GC–FID using a time-automated switching valve (A6-G6 W; Valco Instruments Co., Inc., Texas). The operating conditions of the GC–FID with a packed-type glass column (2.1 m × 3.2 mm i.d.) were as follows: column temperature, 130ºC; injector temperature, 140ºC; and detector temperature, 200ºC. The relative humidity (RH) of the nitrogen gas was monitored by a temperature and humidity transmitter (HMP 233; Vaisala KK, Tokyo, Japan). The humidity was changed in a stepwise manner, where the isothermal condition was 30, 40, 50, and 60ºC. Initially, RH was set at 20% and the sample was left in this condition for 2 h. After the allocated time, the RH was suddenly increased to 50, 60, 70, and 80% for each constant temperature, and sampling continued for another 2 h. To obtain the desired RH, the temperature of the water bath was calculated according to Antoine’s equation [Citation44]. The release data using DVS are indicated one set at one condition and are not statistically average value. However, total flavor content and surface flavor content were measured as triple replication.
The flavor release flux of the encapsulated powder was calculated using equations 1 and 2 [Citation45] as follows:
Where, F is the release flux of flavor (mg/s∙m2∙g-powder), N is the flavor release rate (mg/s), Cg is the flavor concentration (mg/mL N2), V is the nitrogen flow rate (mL/s), A is the area of the sample holder plate (1.3 × 10−4 m2), and m is the mass of the sample (g).
Results and discussions
Flavor release profile from the encapsulated yeast powder using the DVS instrument
shows the release behavior of d-limonene from the encapsulated yeast powder with a stepwise change in RH under the DVS system. At an initial constant humidity of 20% RH, the release flux from the d-limonene encapsulated yeast powder decreased gradually and became close to zero. The initial release might be due to the release of d-limonene adsorbed on the surface of the yeast powder. The total and surface d-limonene content in encapsulated powder were 120 mg/g-dry powder [Citation15], and 1.03 mg/g-dry powder, respectively. Although most of the total flavor was encapsulated in the yeast cells, not all the flavor could penetrate inside the lipid bilayer membrane. Some flavor might be near the yeast cell wall. The easier release of that flavor compared with the inside flavor of the lipid bilayer membrane is probably the reason for the initial higher release flux. The skeleton of the yeast cell is mostly composed of β-glucan and chitosan [Citation46] and provides rigidity for the cell wall [Citation47]. In the present study, using partially extracted β-glucan yeast cells raises the possibility of easier release of flavor from near the cell wall.
Figure 1. Release profile of encapsulated d-limonene in stepwise relative humidity at different incubation temperature (● 20% RH to 50% RH, ▲ 20% RH to 60% RH, ■ 20% RH to 70% RH, ♦ 20% RH to 80% RH) (dotted line indicates the starting point to stepped humidity change).
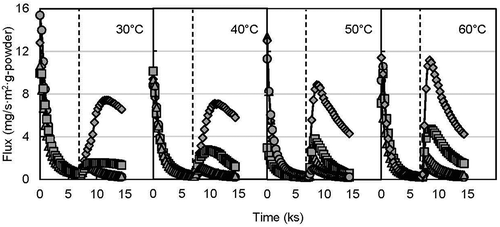
After the stepwise humidity changes, the release flux of d-limonene increased gradually to the maximum fluxes at 80% RH at 30, 40, 50, and 60ºC. The creation of several holes from the swelling of the protein layer of the cell wall is a consequence of the moisture sorption by yeast cells [Citation48], which facilitates volatile release from the cells. Flavor compounds from encapsulated yeast can be released either by evaporation through holes or by diffusion through the cell wall [Citation25]. In other humidity cases, the release flux increased only a little. The moisture content of the d-limonene encapsulated yeast powder was 8.15, 7.91, 9.05, and 13% at 50, 60, 70, and 80% RH, respectively, at 40ºC incubation temperature. The result indicates the proportional relationship between %RH and moisture content of the powder. The decreasing value of release flux after the top peak might be due to the solubilized gelled form of β-glucan, caused by absorbing water. Bohn et al. [Citation41] also found a slower rate of volatile release due to the collapse of the amorphous matrix. However, the release flux increased at the beginning of each level of RH, which might be due to the sudden adsorption of moisture by the cell wall. As expected, not only RH but also temperature triggered flavor release. The release flux was highest (~12 mg/s∙m2∙g-powder) at 60ºC incubation compared with 30, 40, and 50ºC. The higher temperature elevates the molecular movement of volatile compounds, which governs the diffusivity of that compound. Chalier et al. [Citation49] obtained a higher apparent diffusivity of carvacrol from soy protein isolate-coated paper by increasing storage temperature and RH.
illustrates the release profile of ethyl hexanoate under the same experimental conditions mentioned above for d-limonene. The release trends were also similar to the release of d-limonene. However, the initial release fluxes were more than double (~40 mg/s∙m2∙g-powder) the release fluxes of d-limonene (~12 mg/s∙m2∙g-powder), which might be due to the higher holding capacity of ethyl hexanoate (total flavor 225 mg/g-dry yeast powder) by the yeast [Citation15] in the encapsulation. Furthermore, the hydrophilic properties of ethyl hexanoate (log P = 2.8 [Citation50]) also assisted the release of ethyl hexanoate during absorption of moisture. In contrast, the release flux of d-limonene was lower than ethyl hexanoate which might be due to higher log P value (4.8 [Citation51]) (very low solubility in water) and lower holding capacity of d-limonene in yeast. Thus, d-limonene release might be limited with moisture absorption compared to ethyl hexanoate. In addition, the release flux did not drop at 30 and 40ºC even at 80% RH, which indicates the availability of encapsulated ethyl hexanoate. After a stepwise change of RH to 50 and 60%, the release flux for d-limonene and ethyl hexanoate remained almost zero (except the small peak at a sudden change in RH). This result agrees with that of Normand et al. [Citation25] who found that flavor release from yeast cell occurs when the equilibrium relative humidity exceeds 70%. A dry and low temperature storage condition is suggested to avoid undesirable flavor release from yeast-based encapsulant.
Analysis of the flavor release flux
Flavor release behavior kinetics from a single microcapsule such as spray-dried emulsified flavor are categorized typically by 0, 0.5, and 1st order kinetics [Citation52]. Various mathematical models are used to explain the kinetics of flavor release. Avrami’s equation (Weibull equation) is a model used extensively to clarify encapsulated flavor [Citation32] or gas [Citation53] release kinetics. The flavor release kinetics of this study was described using a modified Avrami equation (3, 4, 5) or Weibull distribution function [Citation54,Citation55].
where Y is the flavor release fraction at time t from the stepped RH change, k is the release rate constant, and n is the release mechanism parameter. The double logarithm of the above equation is
The flavor release fraction (Y) can be replaced by the flavor release amount to total released amount of flavor:
where Mt is the integrated released flavor (mg/m2∙g-powder) and M∞ is the total amount of released flavor after stepwise change of RH (mg/m2∙g-powder). Mt and M∞ can be defined as: Mt = and M∞ =
The integrated flavor release area was calculated by accumulating area under the curve obtained from the stepped RH change (right side of dotted line in and ) using trapezoidal rules in Excel 2013. The integration of the flavor release flux was obtained as Mt and M∞. By using equation (5), the release rate constant k and the flavor release mechanism number n were obtained by plotting the left-hand side of the equation (5) against the natural logarithm of time as shown in for the d-limonene encapsulated yeast powder and for the ethyl hexanoate encapsulated yeast powder. Upward linear lines were observed for each RH and incubation temperature condition. The parameters used are shown in . The correlation coefficient (R2) for all conditions was 1.0, which indicates the well-fitted data results of time and integrated flavor release. The slopes for d-limonene were a little steeper than those for ethyl hexanoate. The slope and mechanism number for both flavor releases increased with humidity.
Table 1. Maximum integrated release of flavor (M∞), release rate constant (k), and release mechanism parameter (n) under different humidity conditions at different incubation temperatures.
Analysis of the parameters of release kinetics
The mechanism number n and the total amount of released flavor after stepwise RH change M∞ as a function of RH are shown in . As expected, M∞ increased sharply with RH. The highest value of the maximum integrated released flavors for both types were found at 80% RH than 50, 60, and 70% for all incubation temperatures. The increasing amount of flavor release with RH is due to the moisture sorption properties of the yeast cell. Water molecules migrate to the deeper side via the protein layer and glucan layer of the yeast cell wall [Citation48]. The formation of gel or solubilization of β-glucan by absorbing water perhaps initiates the flavor release behavior of yeast cells. According to Bouquerand et al. [Citation56], flavor release from particles to water occurs when polymer is completely solvated or forms a hydrogel.
Figure 5. Graphical illustration of (a) release mechanism number (● d-limonene; ∆ ethyl hexanoate) and (b) maximum integrated release (●○ 30°C, ▲∆ 40°C, ■□ 50°C, and ♦◊ 60°C; solid keys: d-limonene and open keys: ethyl hexanoate) as a function of RH.
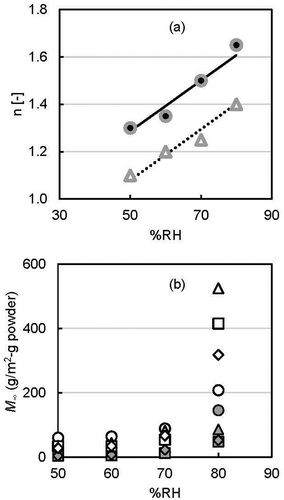
The upward straight lines denote the positive correlation of n with RH. The slopes of the correlation line of n to RH for d-limonene and ethyl hexanoate were the same, 0.0108. The values of n (release mechanism parameter) for both the flavors were greater than 1.0. A release with induction period (initial constant stage or initial slow stage of release flux) occurs if the value of n is higher than 1.0 [Citation36]. Therefore, it can be said that the entrapped flavors from yeast cells followed controlled release with a lag time in the release time course. The mass diffusion coefficient perhaps plays a role in this type of behavior.
Release kinetic constant
As shown in , the rate constant of ethyl hexanoate increased from 1.9 × 10−4 s−1 to 3.23 × 10−4 s−1 at 50% RH for 30 and 60ºC incubation, respectively, whereas at 80% RH the values did not change much. On the other hand, the rate constants for d-limonene were 0.7 × 10−4 s−1 and 2.7 × 10−4 s−1 at 80% RH for 30 and 60ºC incubation, respectively. Li & Lu [Citation57] also noted that the d-limonene release rate constant increased when storage temperature was increased. The relationship between rate constants and RH is controversial in other studies of release kinetics. Ho et al. [Citation53] found a positive correlation of rate constant with RH for ethylene release from ethylene-α-CD complex, whereas Yoshii et al. [Citation37] found the opposite trend for ethyl butyrate release from 20 and 30% maltodextrin blended gum arabic. This contradiction might be due to the difference in the release mechanism number. Comparative slower migration of volatile compounds from the inside deeper lipid bilayer membrane may lead to above-mentioned type of release characteristics. Sultana et al. [Citation15] also found a 2-stage release from yeast cells where the 1st stage showed rapid release. Environmental humidity changes the water distribution in spray-dried powder, which modifies the powder structure and diffusion coefficient of flavor [Citation43]. However, the uncontrolled swelling of the external layer due to moisture absorption causes the breakdown of cell protection and allows exchange with an external medium [Citation25].
Analysis of activation energy and associated release parameter
Activation energy (E) is the minimum energy above which a chemical reaction takes place. Therefore, it affects the chemical stability of core compounds in dried powder. Activation energy was calculated by using the Arrhenius equation and plotting the value of rate constants against the reverse value of temperature (). The activation energies for d-limonene were approximately 3, 9, 20, and 41 kJ/mol at 50, 60, 70, and 80% RH, respectively. In contrast, the value for ethyl hexanoate (14 kJ/mol) remained the same for 50, 60, and 70% RH, and it decreased to 7 kJ/mol at 80% RH.
Figure 6. Arrhenius plot of encapsulated flavor release rate constant with incubation temperature (●,○ 50% RH; ▲, ∆ 60% RH; ■, □ 70% RH; ♦, ◊ 80% RH; solid keys: d-limonene and open keys: ethyl hexanoate).
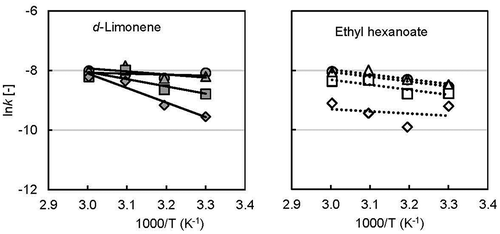
A graphical representation ()) shows the above results. However, d-limonene required more energy than ethyl hexanoate under high RH conditions. The hydrophilic behavior of ethyl hexanoate may have an affinity to migrate towards water faster compared with hydrophobic d-limonene. The activation energies of d-limonene, l-menthol, AITC, and ethyl-n-butyrate have been reported as 123, 111, 54, and 66 kJ/mol [Citation36], respectively, which indicates that various factors are involved in dissociating enclosed flavor. Volatile compounds with similar physical properties can still have different activation energies based on the coated material and release mechanism. The natural logarithm of frequency factor/pre-exponential factor (k0) was plotted against the activation energy E ()), and a good correlation line (R2 ≥ 0.99) was obtained for both flavors. This good correlation might indicate a common release mechanism for both flavors from yeast cells.
Conclusion
The kinetics of hydrophobic (d-limonene) and hydrophilic (ethyl hexanoate) flavor release from partially extracted β-glucan yeast cells were analyzed in this study. The real-time release flux of those flavors was measured by changing humidity in a stepwise manner using a home-built dynamic vapor sorption system coupled with a gas chromatography. The amount of release flux was maximal at 80% RH and 60ºC incubation. The initial higher flux represented the surface flavor or the flavors near the cell wall (outside the lipid bilayer membrane). Both flavors exhibited a linear relationship for integrated release with analysis time. The release mechanism parameters were positively influenced by relative humidity. Both types of flavor showed the similar nature of release pattern. However, the effect of RH on flavor release from yeast cells under continuously ramped humidity compared with stepped humidity is still under consideration.
Author Contributions
H. Yoshii and A. Sultana planned the study. A. Sultana conducted the whole experiment and wrote the manuscript. H. Yoshii guided the experiment and commented on the manuscript.
Acknowledgment
Afroza Sultana thanks the Japan Ministry of Education, Culture, Sports, Science and Technology for providing a scholarship (Monbukagakusho: MEXT).
Disclosure statement
No potential conflict of interest was reported by the authors.
Additional information
Funding
References
- Chartier F. Taste buds and molecules: the art and science of food, wine, and flavor. Reiss, L. (trans.). New Jersey: John Wiley and Sons, Inc.; 2012.
- Djordjevic J, Zatorre RJ, Jones-Gotman M. Effects of perceived and imagined odors on taste detection. Chem Senses. 2004;29:199–208.
- Choi NE, Han JH. How flavor works: the science of taste and aroma: taste is regulated by flavor, and flavor is regulated by the brain. UK: John Wiley & Sons Ltd.; 2015.
- Auvray M, Spence C. The multisensory perception of flavor. Conscious Cogn. 2008;17:1016–1031.
- Shimemura T, Fujita K, Kashimori Y. A neural mechanism of taste perception modulated by odor information. Chem Senses. 2016;41:579–589.
- Small DM, Prescott J. Odor/taste integration and the perception of flavor. Exp Brain Res. 2005;166:345–357.
- Spence C. Multisensory flavor perception. Cell. 2015;161:24–35.
- Jafari SM, Assadpoor E, He Y, et al. Encapsulation efficiency of food flavours and oils during spray drying. Dry Technol. 2008;26:816–835.
- Madene A, Jacquot M, Scher J, et al. Flavour encapsulation and controlled release - A review. Int J Food Sci Technol. 2006;41:1–21.
- Hasani S, Ojagh SM, Ghorbani M. Nanoencapsulation of lemon essential oil in Chitosan-Hicap system. Part 1: study on its physical and structural characteristics. Int J Biol Macromol. 2018;115:143–151.
- Pabast M, Shariatifar N, Beikzadeh S, et al. Effects of chitosan coatings incorporating with free or nano-encapsulated Satureja plant essential oil on quality characteristics of lamb meat. Food Control. 2018;91:185–192.
- Sherry M, Charcosset C, Fessi H, et al. Essential oils encapsulated in liposomes: A review. J Liposome Res. 2013;23:268–275.
- Lin L, Zhu Y, Thangaraj B, et al. Improving the stability of thyme essential oil solid liposome by using β-cyclodextrin as a cryoprotectant. Carbohydr Polym. 2018;188:243–251.
- Sultana A, Miyamoto A, Hy Q L, et al. Microencapsulation of flavors by spray drying using Saccharomyces cerevisiae. J Food Eng. 2017;199:36–41.
- Sultana A, Tanaka Y, Fushimi Y, et al. Stability and release behavior of encapsulated flavor from spray-dried Saccharomyces cerevisiae and maltodextrin powder. Food Res Int. 2018;106:809–816.
- Amrita K, Dabur RS, Kapoor CM. Application of microencapsulation technology in dairy industry. Indian Food Ind. 1999;18:57–64.
- Czerniak A, Kubiak P, Białas W, et al. Improvement of oxidative stability of menhaden fish oil by microencapsulation within biocapsules formed of yeast cells. J Food Eng. 2015;167:2–11.
- Bishop JRP, Nelson G, Lamb J. Microencapsulation in yeast cells. J Microencapsul. 1998;15:761–773.
- Pham-Hoang BN, Voilley A, Waché Y. Molecule structural factors influencing the loading of flavoring compounds in a natural-preformed capsule: yeast cells. Colloids Surf B Biointerfaces. 2016;148:220–228.
- Paramera EI, Konteles SJ, Karathanos VT. Stability and release properties of curcumin encapsulated in Saccharomyces cerevisiae, β-cyclodextrin and modified starch. Food Chem. 2011;125:913–922.
- Paramera EI, Konteles SJ, Karathanos VT. Microencapsulation of curcumin in cells of Saccharomyces cerevisiae. Food Chem. 2011;125:892–902.
- Shi G, Rao L, Yu H, et al. Stabilization and encapsulation of photosensitive resveratrol within yeast cell. Int J Pharm. 2008;349:83–93.
- Shi G, Rao L, Yu H, et al. Yeast-cell-based microencapsulation of chlorogenic acid as a water-soluble antioxidant. J Food Eng. 2007;80:1060–1067.
- Serozym Laboratories. Modification of micro-organisms - by addition of neodymium or magnesium chlorides or onion juice. French patent FR 2179528. 1973.
- Normand V, Dardelle G, Bouquerand P, et al. Flavor encapsulation in yeasts: limonene used as a model system for characterization of the release mechanism. J Agric Food Chem. 2005;53:7532–7543.
- Wu J, Guan Y, Zhong Q. Yeast mannoproteins improve thermal stability of anthocyanins at pH 7.0. Food Chem. 2015;172:121–128.
- Jaehrig SC, Rohn S, Kroh LW, et al. In vitro potential antioxidant activity of (1→3), (1→6)-β-d-glucan and protein fractions from Saccharomyces cerevisiae cell walls. J Agric Food Chem. 2007;55:4710–4716.
- Križková L, Ďuračková Z, Šandula J, et al. Antioxidative and antimutagenic activity of yeast cell wall mannans in vitro. Mutat Res Genet Toxicol Environ Mutagen. 2001;497:213–222.
- Pham-Hoang BN, Romero-Guido C, Phan-Thi H, et al. Encapsulation in a natural, preformed, multi-component and complex capsule: yeast cells. Appl Microbiol Biotechnol. 2013;97:6635–6645.
- Paramera EI, Karathanos VT, Konteles SJ. Yeast cells and yeast-based materials for microencapsulation. In: Gaonkar AG, Vasisht N, Khare AR, et al., editors. Microencapsulation in the food industry: A practical implementation guide. USA: Academic Press; 2014. p. 267–281.
- Pothakamury UR, Barbosa-Cánovas GV. Fundamental aspects of controlled release in foods. Trends Food Sci Technol. 1995;6:397–406.
- Shiga H, Yoshii H, Taguchi R, et al. Release characteristics of flavor from spray-dried powder in boiling water and during rice cooking. Biosci Biotechnol Biochem. 2003;67:426–428.
- Soottitantawat A, Takayama K, Okamura K, et al. Microencapsulation of l-menthol by spray drying and its release characteristics. Innovative Food Sci Emerg Technol. 2005;6:163–170.
- Takashige S, Hermawan DA, Shiga H, et al. Flavor release behavior from emulsified d-limonene in spray-dried powders with various wall materials. Jpn J Food Eng. 2017;18:53–58.
- Yoshii H, Sakane A, Kawamura D, et al. Release kinetics of (-)-menthol from chewing gum. J Incl Phenom Macrocyclic Chem. 2007;57:591–596.
- Furuta T, Soottitantawat A, Neoh TL, et al. Effect of microencapsulation on food flavors and their releases. In: Devahastin S, editor. Physicochemical aspects of food engineering and processing. US: CRC Press; 2011. p. p 4–35.
- Yoshii H, Soottitantawat A, Liu XD, et al. Flavor release from spray-dried maltodextrin/gum arabic or soy matrices as a function of storage relative humidity. Innovative Food Sci Emerg Technol. 2001;2:55–61.
- Nguyen TVA, Yoshii H. Release behavior of allyl sulfide from cyclodextrin inclusion complex of allyl sulfide under different storage conditions. Biosci Biotechnol Biochem. 2018;82(5):848–855.
- Soottitantawat A, Yoshii H, Furuta T, et al. Effect of water activity on the release characteristics and oxidative stability of d-limonene encapsulated by spray drying. J Agric Food Chem. 2004;52:1269–1276.
- Levoguer CL, Williams DR. The characterisation of pharmaceutical materials by dynamic vapour sorption. Application Note 101. Available from Surf Meas Syst., London, UK.
- Bohn DM, Cadwallader KR, Schmidt SJ. Development and validation of a dynamic vapor sorption-fast gas chromatography-flame ionization detection method for rapid analysis of volatile release from glassy matrices. J Agric Food Chem. 2005;53:3149–3155.
- Dronen DM, Reineccius GA. Rapid analysis of volatile release from powders using dynamic vapor sorption atmospheric pressure chemical ionization mass spectrometry. J Food Sci. 2003;68:2158–2162.
- Yamamoto C, Neoh TL, Honbou Y, et al. Evaluation of flavor release from spray-dried powder by ramping with dynamic vapor sorption–gas chromatography. Dry Technol. 2012;30:1045–1050.
- Rodgers RC, Hill GE. Equations for vapour pressure versus temperature: derivation and use of the Antoine equation on a hand-held programmable calculator. Br J Anaesth. 1978;50:415–424.
- Yamamoto C, Neoh TL, Honbou H, et al. Kinetic analysis and evaluation of controlled release of d-limonene encapsulated in spray-dried cyclodextrin powder under linearly ramped humidity. Dry Technol. 2012;30:1283–1291.
- Ishihara S, Hirata A, Nogami S, et al. Homologous subunits of 1,3-beta-glucan synthase are important for spore wall assembly in Saccharomyces cerevisiae. Eukaryot Cell. 2007;6:143–156.
- Utsugi T, Minemura M, Hirata A, et al. Movement of yeast 1,3-β-glucan synthase is essential for uniform cell wall synthesis. Genes Cells. 2002;7:1–9.
- Dardelle G, Normand V, Steenhoudt M, et al. Flavour-encapsulation and flavour-release performances of a commercial yeast-based delivery system. Food Hydrocoll. 2007;21:953–960.
- Chalier P, Ben Arfa A, Guillard V, et al. Moisture and temperature triggered release of a volatile active agent from soy protein coated paper: effect of glass transition phenomena on carvacrol diffusion coefficient. J Agric Food Chem. 2009;57:658–665.
- Dury-Brun C, Hirata Y, Guillard V, et al. Ethyl hexanoate transfer in paper and plastic food packaging by sorption and permeation experiments. J Food Eng. 2008;89:217–226.
- Onken J, Berger RG. Effects of R-(+)-limonene on submerged cultures of the terpene transforming basidiomycete Pleurotus sapidus. J Biotechnol. 1999;69:163–168.
- Soottitantawat A, Partanen R, Neoh TL, et al. Encapsulation of hydrophilic and hydrophobic flavors by spray drying. Jpn J Food Eng. 2015;16:37–52.
- Ho BT, Joyce DC, Bhandari BR. Release kinetics of ethylene gas from ethylene-α-cyclodextrin inclusion complexes. Food Chem. 2011;129:259–266.
- Avrami M.Kinetics of phase change. II Transformation-time relations for random distribution of nuclei. J Chem Phys. 1940;8(2):212–224.
- Neoh TL, Yoshii H, Furuta T. Encapsulation and release characteristics of carbon dioxide in α-cyclodextrin. J Incl Phenom Macrocyclic Chem. 2006;56:125–133.
- Bouquerand PE, Maio S, Normand V, et al. Swelling and erosion affecting flavor release from glassy particles in water. AIChE J. 2004;50:3257–3270.
- Li PH, Lu WC. Effects of storage conditions on the physical stability of d-limonene nanoemulsion. Food Hydrocoll. 2016;53:218–224.