ABSTRACT
Lactoferrin (LF) is a naturally occurring iron-binding glycoprotein with a variety of biological functions. It has increasing demand every year and huge market potential. In this study, we explored the feasibility of expressing human LF (hLF) in edible algae C. reinhardtii. A codon-optimized hLF gene was synthesized, inserted into pCAMBIA-1301C and transformed into C. reinhardtii SP strain. In total, 7 hLF-expressing clones were selected with clone 121 exhibiting the highest expression level. The hLF-containing algal extract significantly inhibited the growth of bacteria such as Escherichia coli and Klebsiella variicola. During acute toxicity experiment no acute toxicity was detected, especially on changes of the body weight and histopathology of organs. The recombinant hLF possessed a similar or modestly reduced stability compared to commercial hLF standard. Our data indicated that expression of hLF in C. reinhardtii is feasible and paved a way to commercial production of lactoferrin using edible Chlamydomonas expression system.
Abbreviations: atrazine chlorohydrolase gene (atzA); bovine serum albumin (BSA); human LF (hLF); lactoferrin (LF); Luria-Bertani (LB); quantitative reverse transcriptase PCR (qRT-PCR) ; SDS polyacrylamide gel electrophoresis (SDS-PAGE); Tris-acetate phosphate (TAP); western blotting (WB)
Graphical abstract
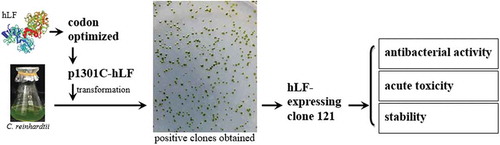
Expression of human lactoferrin in Chlamydomonas reinhardtii had significant antibacterial activity, stable physical-chemical property and no acute toxicity to mice.
Lactoferrin (LF) is an 80 kDa iron-binding glycoprotein, belonging to the transferrin family, and was first found in bovine milk and subsequently isolated from human milk [Citation1]. Subsequent studies reported the presence of human LF (hLF) in body fluid such as eye drops, bile and saliva [Citation2]. LF contains two symmetrical, highly homologous lobe-shaped domains, connected by a hinge region. The N-terminal domain of LF has been reported to have antibacterial activity whereas the C-lobe has been suggested to play important roles in the recovery of stomach diseases, diabetes and corneal damage [Citation3]. LF has a diverse range of functions including promotion of iron binding, broad-spectrum antibacterial activity, antiviral activity, anti-tumor activity, immunomodulatory, anti-oxidizing ability and promotion of the proliferation of intestinal epithelial cells [Citation4]. Therefore, LF is commonly used as an additive in the formulation of fetal powder milk, yoghurt and animal forages [Citation5]. The diverse functions render LF an attractive target of protein production, with the bovine LF [Citation6] and human LF [Citation7] being the most investigated.
A number of heterologous protein expression systems could serve as the production of recombinant LF, including bacteria, fungi, plants, mammalian cells and transgenic animals. Each of these systems offers distinct advantages in terms of protein yield, ease of manipulation and cost of operation [Citation8]. As LF is a glycoprotein, bacteria-expressed LF typically exhibited atypical structures due to the lack of glycosylation [Citation9]. Although LF expressed from plants can be glycosylated, the difficulties in sowing, planting and harvesting impede the rapid, large-scale production. While transgenic animals can serve as good host for producing functional LFs, they pose the risk of viral contamination.
C. reinhardtii has been used as a model organism for molecular genetic studies for more than 30 years [Citation10]. C. reinhardtii has a rapid cell division rate of 8 h to 12 h per generation, is generally recognized as safe (GRAS) microorganism with little genetic drift, making it a protein factory with high biosafety [Citation11]. The nuclear and plastid gene transfer systems have been well established [Citation12]. Importantly, protein expressed in the alage eliminates the risk of contamination of animal viruses and endotoxins [Citation13]. It is not surprising that C. reinhardtii has been proposed as a platform for production of high valuable proteins. Examples of successfully expressed proteins in C. reinhardtii include antibodies, immunotoxins, vaccination and other therapeutic proteins [Citation14]. And as one of the edible microalgae, transgenic C. reinhardtii expressed phytase had been used as food additive with no need of protein purification [Citation15]. This finding indicated that C. reinhardtii can be used for health care products expression, such as hLF with biological functions including antibacterial, antiviral and immunomodulatory activities.
In this study, we explored the feasibility of expressing hLF in C. reinhardtii. The recombinant hLF in the algae protein extract exhibited significant antibacterial activity and little toxicity to mice. Compared with commercial hLF expressed in rice, the recombinant hLF in algae showed similar or modestly lower stability under temperature, pH, pepsin and trypsin challenge. These data indicated that it is feasible to express LF in C. reinhardtii and thus shed the light on production of recombinant hLF using edible algae expression system.
Materials and methods
C. reinhardtii culture
The C. reinhardtii SP strain used in this study was a gift from Prof. Fuchen Shi, Nankai University and cultured in liquid or agar Tris-acetate phosphate (TAP) medium as described [Citation16]. The liquid medium was used for algae amplification and agar medium for transformation selection. The culture was incubated at 24°C under a 16-h light/8-h dark cycle with 50 μmol/m2/s light intensity of a PRX-450C incubator (Haishusaifu Experimental Instrument Factory, Ningbo, China). To test if expression of recombinant lactoferrin affects the growth of Chlamydomonas cells, OD750 of the cultures were monitored for 9 day. The morphology of the algal cells was inspected by an optical microscope (Olympus, Tokyo, Japan). Algae cells at a density of 1 × 106 cells/mL were collected by centrifugation for preparation of electro-competent cells or extraction of genomic DNA.
Vector construction and transformation
Plant binary expression vector pCAMBIA-1301 (RA Jefferson, CAMBIA, Australia) was used for cloning hLF. The hygromycin phosphotransferase (HPT) gene in the vector was replaced with an atrazine chlorohydrolase gene (atzA) to improve the selection efficiency [Citation17]. An additional Kozak sequence was introduced before the start codon of the atzA gene to improve the expression [Citation18]. A 35S promoter was chosen to facilitate the expression of hLF and atzA in C. reinhardtii [Citation19]. The recombinant hLF gene was synthesized using GenBank sequence M93150.1 as a reference, codon optimized (Figure S1) and then inserted into the BamHI/SalI site of pCAMBIA-1301C vector. The recombinant plasmid was verified by DNA sequencing and named as p1301-syn-hLF.
p1301-syn-hLF was transferred into C. reinhardtii SP competent cells by electroporation [Citation20]. One μg of plasmid DNA was used for transformation of 1 × 106 C. reinhardtii cells. Empty vector pCAMBIA-1301C was also transformed into C. reinhardtii as a negative control. Transformed algae cells were first cultured in liquid medium for 24 h in darkness for recovery and then transferred to TAP agar plates supplemented with 0.2 mg/L atrazine and cultured for 7 days until the large single colonies appeared. Approximately 500 colonies were obtained and 100 larger colonies were selected for PCR analysis. The colonies were inoculated into TAP liquid medium containing 0.2 mg/L atrazine. The genomic DNA of each clone was isolated using a modified Cetyltrimethyl Ammonium Bromide (CTAB) method [Citation21]. Primers atzA-F1/atzAF-863 and hLF-check-F/hLF-check-R (Table S1) were used for detecting the presence of hLF and atzA. PCR reactions were carried out as follows: 94°C for 5 min; 35 cycles of 94°C for 30 s, 58°C for 1 min 30 s, and 72°C for 60 s; and 72°C for 7 min. The PCR products were verified by DNA sequencing.
qRT-PCR analysis of hLF-containing clones
Total RNA was isolated from algae cells using the RNAprep Pure Plant Kit (Tiangen Biotech Co., Ltd, Beijing, China) as recommended by the manufacturer. The reverse transcription reaction was performed at 75°C for 15 min, 42°C for 100 min and then 47°C for 60 min in a reaction containing 5 μg total RNA (10 μL), 0.5 μL primer Smart-3-end-Gan (10 pmol/μL), 1.5 μL nuclease-free dNTP (10 mM/L), 4 μL 5 × RT-first buffer, 1 μL RNase Inhibitor (40 U/μL) and 1 μL RNase H-free Reverse Transcriptase M-MLV (200 U/μL) (Takara Biotechnology Co., Ltd., Dalian, China).
qRT-PCR was performed in 25 μL reaction solution containing 100 ng cDNAs (2 μL), 0.4 μM/L each of forward and reverse primers (Table S1), 1 × SYBR Premix Ex TaqTM (Takara Biotechnology, Ltd., Dalian, China). Reactions were carried out in CFX Connect Real-time System (Bio-Rad Laboratories, California, USA) using the following cycling conditions: pre-denaturation at 95°C for 30 s, 40 cycles of 95°C for 5 s and 58°C for 30 s. Relative expression level was calculated by the 2−∆∆CT method [Citation22] with actin (Accession No. D50839) as the internal control. Three biological replicates were performed for each sample.
Extraction of total proteins and quantification of hLF protein in hLF-expressing clones
C. reinhardtii cells were collected by centrifugation at 12,000 g for 10 min at 4°C. The cell pellet was suffered freeze-thaw cycles, and then ground in extraction buffer containing 50 mM Tris-HCl, 40 mM NaCl, 0.1% Tween-20 and 1 mM phenylmethanesulfonyl fluoride [Citation23]. Cells were lysed by SCIENTZ-IID sonicator (Ningbo Scientz Biotechnology Co., Ltd, Ningbo, China). Cell lysates were centrifuged at 12,000 g for 30 min at 4°C. To remove salts and low MW components, the supernatant was dialyzed in dialysis buffer (50 mM sodium phosphate, 0.5 M NaCl) overnight at 4°C using dialysis bag MD77 (8000–14,000) (Shanghai 3bio Biotechnology Co., Ltd., Shanghai, China). Then the solution was concentrated using PEG20000 and lyophilized using Novalyple-NL500 vacuum freeze dryer (Thermo Fisher Scientific Inc., MA, USA). Lyophilized protein was reconstituted in phosphate buffered saline (137 mM NaCl, 2.7 mM KCl, 10 mM Na2HPO4, and 2 mM KH2PO4). Total soluble protein concentration was determined by Bradford method [Citation24] using bovine serum albumin (BSA) as a standard.
Approximately 40 μg of total protein from each positive clone and 1 μg commercial hLF were blotted and resolved on a 10% SDS-PAGE (SDS-polyacrylamide gel electrophoresis), and then transferred onto 0.45 μm PVDF membranes using a DYCZ-40G protein transmembrane instrument (Liuyi Experimental Instrument Factory, Beijing, China) at 350 mA for 2.5 h. The membrane was blocked with 5% milk powder for 1 h at room temperature. hLF was detected with a 1: 8000 dilution (v/v) of Anti-human Lactoferrin Antibody (Jackson Immuno Research Laboratories, Inc., PA, USA). After incubation at 4°C overnight, the membrane was washed four times with TBST (Tris-Buffered Saline and Tween-20) buffer containing 20 mM Tris-HCl, pH7.5, 150 mM NaCl and 0.1% Tween-20. The hybridization signals were visualized by ECL detection system (Tanon Science & Technology Co., Ltd. Shanghai, China). The gel stained with Coomassie served as loading control. Three biological replicates were performed for each sample. Quantity one ver4.62 (Bio-Rad Laboratories, Inc. California, USA) was used to quantify the protein of interest in each sample. The recombinant hLF concentration was assessed compared with known concentration of commercial standard hLF using western blotting. The expression level of the recombinant hLF was calculated as: concentration ×100%/total soluble protein concentration per lane (40 µg).
Assessing the antibacterial activity of algae-expressed hLF protein extract
Antibacterial activity of hLF was analyzed on Escherichia coli DH-5α and Klebsiella variicola IV-3 as described [Citation25]. The bacteria were inoculated on to LB (Luria-Bertani) agar plates and cultured at 37°C overnight. Single colonies were chosen for inoculation in liquid LB medium, grown at 37°C overnight. The next day, bacteria were diluted to a cell density of initial 106 CFU (colony forming units)/mL in LB medium supplemented with 27.5 mg/mL algae protein extract from clone 121 (relative to 0.5 mg/mL recombinant hLF), 0.5 mg/mL of the commercial hLF or 27.5 mg/mL of the empty vector clone protein extract. OD600 of the culture was monitored for a 12 h period. Three biological replicates were performed for each sample. Statistical analyses were performed using the S-N-K method (95% confidence) with IBM SPSS Statistics 11.0 (SPSS Inc., Chicago, IL, USA).
Assessing the acute toxicity of algae-expressed hLF protein extract in mice
All animal experiments in this study were approved and supervised by the Institutional Animal Care of Experimental Animal Center, Nankai University, China. Efforts were made to minimize animal suffering and approaches were used to minimize the number of animals used.
Seven weeks old Kunming (KM) mice were housed and cared under controlled conditions with a temperature of 22 ± 3°C, a relative humidity of 50 ± 20% and a 12-h light/12-h dark cycle, with water and food available in a specific-pathogen free (SPF) facility at Nankai University. Acute toxicity study of recombinant hLF was performed following the Acute oral toxicity-Fixed Dose Procedure supported by the OECD (Organization for Economic Co-operation and Development) 420 Guidelines [Citation26]. The male and female mice (15 each) were randomly divided into three groups, respectively. Prior to the experiment, the animals were initially acclimated for 7 days, and then withheld food but no water for 4 h. Physiological saline (0.9% NaCl), BSA or recombinant hLF from algae protein extract aqueous suspension was administered intragastrically for four times with a four-hour interval, and the total dose was up to 2000 mg/kg. After 2 h of the last dose, all the mice were housed with water and food routinely until the end of this experiment. Individual weights were determined at the start of fasting (day 0) and on the test days 3, 5, 7, and 14. The animals were observed for the clinical signs of toxicity during the first 30 min after dosing, and then once every two hours in the first daily-time, once daily thereafter until the end of the study. The behavior, body weight, excretion, food and water consumption were recorded for 14 days. Afterwards, the mice were sacrificed on the 14th day. Mouse organs and tissues were excised and weighted. Relative organ weight was calculated as 100 × organ weight (g)/body weight (g). After that, livers and kidneys were well prepared for the following histopathology assay.
Histopathology assay
Livers and kidneys harvested from each animal were fixed in 10% neutral buffered formalin solution, dehydrated through standard procedures and embedded in paraffin [Citation27]. Subsequently, paraffin blocks were sliced at 5 μM thickness, and then stained with hematoxylin and eosin using an H & E Staining Kit (Solarbio, Beijing, China) to illustrate the changes in morphology. Stained slices were observed by an optical microscope (Olympus, Tokyo, Japan).
Assessing the stability of recombinant hLF in the algae protein extract
The hLF-expressing algae protein extract and freeze-dried powder were prepared as described above. The stability of the recombinant hLF was assessed by quantifying the remaining hLF using WB (western blotting) after different treatment. For the temperature stability test, reconstituted extract was incubated at different temperatures vary from 20°C to 100°C with twenty as the interval. For the pH stability test, freeze-dried powder was incubated at phosphate buffered saline described above with different pH vary from 1 to 9. To test the pepsin stability, protein freeze-dried powder was treated with artificial gastric fluid (37 mM NaCl, 0.03 M HCl, pH1.6) [Citation28] containing 3.2 mg/mL pepsin (Sangon Biotech Co., Ltd, Shanghai, China). For the trypsin stability test, protein freeze-dried powder was treated with artificial intestinal fluid (30 mM K2HPO4, 160 mM NaH2PO4) [Citation28] containing 20 mg/mL pancreatin (Sangon Biotech Co., Ltd, Shanghai, China). Quantity One ver4.62 was used to process and analyze the data. Commercial purified hLF [recombinant human lactoferrin expressed in rice, ≥90% (SDS-PAGE), Sigma-Aldrich, CA, USA; Cat. No. L4040] mixed with protein extract from empty vector clone under the same concentration was used as a reference. Three biological replicates were performed for each sample.
Results
Development and identification of hLF-expressing algae clones
The codon-optimized hLF gene was inserted into pCAMBIA-1301C to obtain the hLF-expressing recombinant vector p1301-syn-hLF ()). After transformed into C. reinhardtii, the larger single colonies were selected on atrazine-resistant agar plates. After inoculated into liquid TAP medium, the cultures were harvested for genomic DNA isolation. The presence of both the atrazine resistant gene and the hLF gene were detected by PCR. In total, seven positive C. reinhardtii clones, namely 51, 71, 97, 101, 103, 106 and 121, were selected and used for subsequent experiments.
Figure 1. Development and characterization of hLF-expressing C. reinhardtii clones. (a) Schematic representation of hLF construct for expressing hLF. The syn-hLF gene in the vector is driven by the Cauliflower mosaic virus (CaMV) 35S promoter and terminated by the nos terminator. The atzA, atrazine chlorohydrolase gene is used as the selection marker. The kozak sequence was introduced to improve the expression of atzA; polyA, CaMV 35S polyA. Rbsc-3A is a gene present in the original plasmid. (b) qRT-PCR quantification of hLF mRNA expression in hLF-expressing C. reinhardtii clones. The algae clone transformed with empty vector was used as negative control. β-actin was used as an internal control. Data are shown as mean ± SD (n = 3). (c) Western blotting analysis of hLF protein expression in hLF-expressing C. reinhardtii clones. Total protein (40 μg) from each clone and the negative control strain were resolved by SDS-PAGE. Commercial lactoferrin (c-hLF, 1 μg) was used as a positive control. nc, negative control. Top, western blotting. Bottom, Coomassie stain.
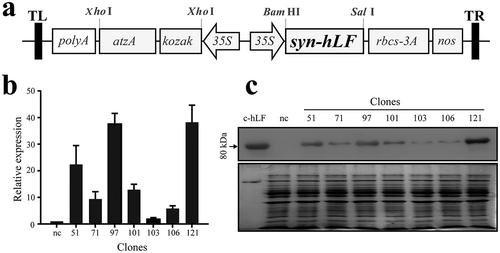
Next we determined the mRNA expression level in the selected hLF-expressing positive clones using quantitative reverse transcriptase PCR (qRT-PCR). Significant difference of hLF expression was detected among these clones, with clone 97 and 121 exhibiting the highest mRNA level ()). Then we determined the protein expression level of these positive clones. The expression level of hLF proteins was generally consistent with the expression levels of mRNA ()). By comparing with standard hLF using western blotting, the expressed recombinant hLF from clone 121 was determined as 0.73 μg per 40 μg total soluble proteins loaded in each lane. Hence, the expressed hLF constituted 1.82% of the total soluble protein in the cell extract. In addition, expression of recombinant hLF in C. reinhardtii did not affect cell proliferation (Figure S2a) or morphology (Figure S2b).
Antibacterial activity of algae-expressed hLF
Two bacteria, E. coli and K. variicola IV-3, were chosen for the antibacterial activity analysis. Compared to the negative control, clone 121 extract significantly inhibited the growth of both E. coli and K. variicola IV-3. The inhibitory effect appeared at 8 h against E. coli, 6 h against K. variicola IV-3 and became more prominent at later time points. Besides, the antibacterial activity of clone 121 extract was equally to the standard commercial hLF as the data indicated ().
Figure 2. Antibacterial activity of the C. reinhardtii-expressed hLF protein extract against two bacteria strains, Escherichia coli DH-5α (a) and Klebsiella variicola IV-3 (b). The two strains (106 CFU/mL) were incubated with 27.5 mg/mL of algae protein extract containing 0.5 mg/mL recombinant hLF from clone 121 for 12 h at 37°C. The algae clone with empty vector pCAMBIA-1301C (27.5 mg/mL) was used as a negative control. The commercial hLF (0.5 mg/mL) used as a positive control. The antibacterial activity was determined by the value of OD600. Data are shown as mean ± SD (n = 3).
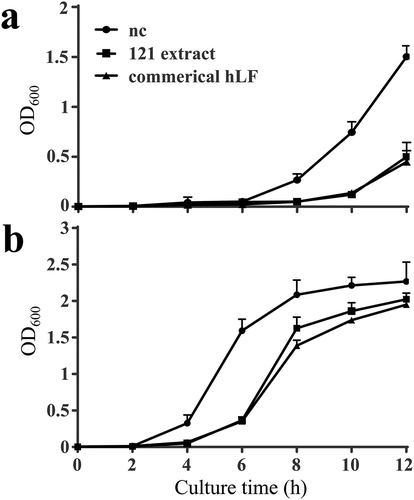
The acute toxicity of algae-expressed hLF
To explore the biosafety of algae-expressed hLF, we examined the acute toxicity of hLF-containing cell extract from clone 121 on KM mice. No death or toxicity signs such as tremors, convulsions, diarrhea, lethargy or coma were observed for all the mice during the whole experiment. No significant difference in food and water consumption (Data not shown) as well as body weight () was observed in these treated mice. Fourteen days after the treatment, mice were sacrificed for investigating the morphological changes on mouse organs and tissues. No significant difference was observed in the weight of the liver and kidney among these three groups (). In addition, no abnormal histopathology in the liver and kidney was observed in the treated mice with 121 extract compared to the control groups (). Taken together, these data suggested that the hLF-containing algae extract did not lead to acute toxicity in KM mice.
Table 1. Body weight increase of mice after a single oral administration of recombinant hLF, BSA and 0.9% NaCl in the acute toxicity study.
Table 2. The relative weights of kidney and liver after oral administration of recombinant hLF, BSA and 0.9% NaCl in mice.
Figure 3. Histologic assessments of liver and kidney sections after oral administration 0.9% NaCl, BSA and 121 protein with 2000 mg/kg in acute toxicity study. Formalin-fixed and paraffin-embedded liver and kidney sections were stained with hematoxylin and eosin. Then visualized at 400 × original magnification, the scale bar is 50 μm.
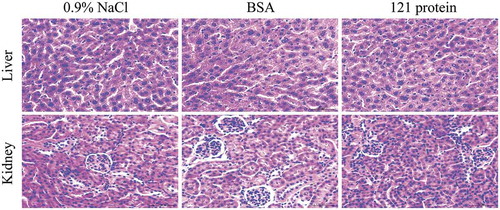
The stability of algae-expressed hLF
To assess the stability of hLF, we treated the hLF-expressing 121 protein under different conditions and detected the retention of hLF after the treatment. Commercial hLF mixed with protein extract from empty vector clone was used as control. Compared to commercial hLF, 121 protein extract exhibited similar stability under most temperatures (20°C, 40°C, 60°C and 80°C), while only showed difference at the extreme temperature (100°C), with commercial hLF retaining nearly 30% of proteins ()).
Figure 4. Stability of C. reinhardtii-expressed hLF protein extract under different temperature (a), pH (b), pepsin digestion (c) and trypsin digestion (d). Clone 121-expressed hLF protein extract was subjected to different treatment conditions, and the retained protein was determined by western blotting compared to that of the untreated samples. Commercial purified recombinant human lactoferrin expressed in rice mixed with protein extract from empty vector clone under the same concentration was used as the control. Data are shown as mean ± SD (n = 3). n.s. indicates no significant difference (p < 0.05) between C. reinhardtii-expressed hLF protein extract and commercial hLF. * indicates a significant difference.
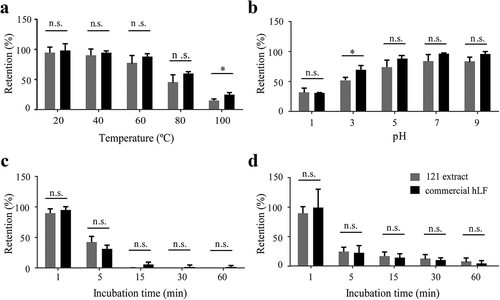
For pH stability, commercial hLF and recombinant hLF only showed difference in the retention of proteins under pH 3 ()). For pepsin and trypsin stability, recombinant hLF displayed similar stability to the commercial hLF, and no difference was observed with prolonged incubation. It was also noted that both commercial hLF and recombinant hLF were very sensitive to pepsin digestion, and nearly all hLF proteins were degraded after incubation for 15 min ().
Discussion
Lactoferrin is a multi-functional glycoprotein with a wide range of applications, including antibacterial, antiviral and immunomodulatory activities. Bovine LF (bLF) has been approved by European Food Safety Authority (EFSA) as a safe additive [Citation29]. Despite the progress on the function characterization of LF, large-scale, highly efficient production of recombinant LF remains a major obstacle. In this study, we investigated the feasibility of expressing recombinant hLF in C. reinhardtii and characterized the antibacterial activity, acute toxicity and stability under different conditions. As far as we know, this is a new system for functionally expressed hLF that never reported.
Chlamydomonas reinhardtii has many advantages compared with traditional systems for molecular farming, which include low production costs, absence of human pathogens and the ability to fold and assemble complex proteins accurately [Citation30]. Compared with hLF expression system in rice, production of hLF in C. reinhardtii would not require the occupation of farmland or the use of pesticides that may cause environmental pollution. These advantages could facilitate large-scale production of hLF and may reduce the overall production cost of hLF.
However, the limited efficiency of transgene expression from the C. reinhardtii nuclear genome hampered itself as a preferred expression host to some extent [Citation31]. To our knowledge, codon usage and GC content are key factors to the efficiency of nuclear gene expression in C. reinhardtii. In this study, we synthesized a fully codon-optimized hLF gene that has a similar GC content as the coding sequences in C. reinhardtii nuclear genome. After selection, we obtained seven hLF positive clones, among which clone 121 showed highest hLF expression level. Thus, the use of codon optimized syn-hLF facilitates the expression of hLF as high as 1.82% of the total soluble protein in C. reinhardtii. Besides, we noted that the mRNA and protein expression of hLF were highly variable among the seven clones, suggesting that the integration position of the hLF in the C. reinhardtii genome maybe an important influence factor for protein expression. Therefore, a relatively large number of transgenic clones was generated and selected in the study to guarantee the obtainment of high-expressing hLF clone.
Microalgae, such as Chlorella and Spirulina, have been recognized as supplement for human nutrition for several decades as these algae are abundant in protein and nutrient contents. Similarly, as an edible green alga, C. reinhardtii had successfully expressed the HIV p24 proteins [Citation32], human erythropoietin [Citation33], malarial vaccines GBSS-AMA1 and GBSS-MSP1 [Citation34] and forage additive xylanase1 protein [Citation35]. Therefore, transgenic microalgae themselves developed in the study, when being viewed as food, would provide beneficial nutrients to the animals. Our research demonstrated that the hLF-containing algae extract is safe and nontoxicity, which is also supported by other researches to some extent [Citation36,Citation37]. Further research is also needed to test the beneficial effect of hLF-expressing algae to animals.
Currently available selectable marker genes for nuclear transformation in C. reinhardtii focus on antibiotic resistance genes, herbicide resistance genes and genes encoding protein or enzymes necessary for normal vital activities [Citation38]. However, antibiotic resistance genes may limit the clinical use of therapeutic protein expression in C. reinhardtii. Enzymes are usually appropriate for complement metabolic deficiencies of auxotrophic mutant strains [Citation38]. However, the acquisition of auxotrophic mutant strains is not so convenient sometimes. It is necessary to develop new marker genes for C. reinhardtii expression system. Our study is the first report using atzA gene as a selectable marker in C. reinhardtii transformation. To improve the expression, an additional Kozak sequence was introduced before the start codon. It works well in the process of positive clone acquisition. Besides, this gene conferred the transgenic algae the ability to degrade atrazine remained in the environment. So, the atzA gene as a new selectable marker gene is recommended in C. reinhardtii transformation.
In an effort to optimize the expression of hLF in Chlamydomonas, recombinant protein accumulation level was up to 1.82% of the total soluble protein. Compared to recombinant proteins expressed in Chlamydomonas in other studies, we obtained a relatively high expression level. A codon optimized YFP in UVM strains reached more than 1% of the total soluble protein, which was claimed by the authors as the highest expression level of a recombinant protein made from a single-copy nuclear transgene in Chlamydomonas [Citation39]. Other researches involved foreign protein expression in Chlamydomonas reported a much lower expression level, such as 10 mg per liter [Citation40], 0.25% of total soluble protein [Citation34], 100 µg per liter [Citation35]. With this work, we proposed that codon optimization is one of key reasons for effective expression in Chlamydomonas; the selection of algae strain and the GC content of objective gene also should be taken into consideration.
Conclusions
In conclusion, we explored the feasibility of expressing hLF in algae C. reinhardtii in this study. The soluble recombinant hLF reached 1.82% of the total cellular soluble proteins. The recombinant hLF exhibited significant antibacterial activity and little toxicity to mice. Compared with commercial hLF, the recombinant hLF showed similar or modestly lower stability under temperature, pH. These data indicated that expression of hLF in C. reinhardtii is feasible and thus paved the way to commercial production of lactoferrin in edible Chlamydomonas.
Author contributions
D.C. and J.L. designed the research; X.P., Y.T., and W.X. performed the research; Y.Y. and X.C. supervised the research; D.C. X.P, and J.L. analyzed the data; X.P. and J.L. wrote the paper; D.C. and X.C. revised the paper.
SI-Pang_XN-BBB-final.doc
Download MS Word (374 KB)Acknowledgments
We wish to thank the Institutional Animal Care of Experimental Animal Center, College of Life Sciences, Nankai University for technical support.
Disclosure statement
No potential conflict of interest was reported by the authors.
Supplementary material
Supplemental data for this article can be accessed here
Additional information
Funding
References
- Mayeur S, Spahis S, Pouliot Y, et al. Lactoferrin, a pleiotropic protein in health and disease. Antioxid Redox Signal. 2016;24(14):813–836.
- Garcia-Montoya IA, Cendon TS, Arevalo-Gallegos S, et al. Lactoferrin a multiple bioactive protein: an overview. Biochim Biophys Acta. 2012;1820(3):226–236.
- Sharma S, Sinha M, Kaushik S, et al. C-lobe of lactoferrin: the whole story of the half-molecule. Biochem Res Int. 2013;2013:271641.
- Expósito LM, Montes RL, Rodríguez LM, et al. Multifunctional capacity and therapeutic potential of lactoferrin. Life Sci. 2018;195:61–64.
- Wang X, Wang X, Hao Y, et al. Research and development on lactoferrin and its derivatives in China from 2011–2015. Biochem Cell Biol. 2017;95(1):162–170.
- Legrand D. Overview of lactoferrin as a natural immune modulator. J Pediatr. 2016;173(Suppl):S10–S15.
- Nandi S, Suzuki YA, Huang J, et al. Expression of human lactoferrin in transgenic rice grains for the application in infant formula. Plant Sci. 2002;163(4):713–722.
- Pang XN, Hong X, Wei X, et al. Research progress in physicochemical characteristics of lactoferrin and its recombinant expression systems. Hereditas. 2015;37(9):873–884. [Article in Chinese].
- Almond RJ, Flanagan BF, Antonopoulos A, et al. Differential immunogenicity and allergenicity of native and recombinant human lactoferrins: role of glycosylation. Eur J Immunol. 2013;43(1):170–181.
- Scaife MA, Nguyen GT, Rico J, et al. Establishing Chlamydomonas reinhardtii as an industrial biotechnology host. Plant J. 2015;82(3):532–546.
- Mayfield SP, Manuell AL, Chen S, et al. Chlamydomonas reinhardtii chloroplasts as protein factories. Curr Opin Biotechnol. 2007;18(2):126–133.
- Rochaix JD. The three genomes of Chlamydomonas. Photosynth Res. 2002;73(1–3):285–293.
- Specht E, Miyake-Stoner S, Mayfield S. Micro-algae come of age as a platform for recombinant protein production. Biotechnol Lett. 2010;32(10):1373–1383.
- Almaraz-Delgado AL, Flores-Uribe J, Pérez-España VH, et al. Production of therapeutic proteins in the chloroplast of Chlamydomonas reinhardtii. AMB Express. 2014;4:57.
- Yoon SM, Kim SY, Li KF, et al. Transgenic microalgae expressing Escherichia coli AppA phytase as feed additive to reduce phytate excretion in the manure of young broiler chicks. Appl Microbiol Biotechnol. 2011;91:553–563.
- Yagi T, Yamashita K, Okada N, et al. Hydrogen photoproduction in green algae Chlamydomonas reinhardtii sustainable over 2 weeks with the original cell culture without supply of fresh cells nor exchange of the whole culture medium. J Plant Res. 2016;129:771–779.
- Gong W, Zhao L, Hu B, et al. Identifying novel salt-tolerant genes from Dunaliella salina using a Haematococcus pluvialis expression system. Plant Cell Tissue Org Cult. 2014;117:113–124.
- Wang H, Chen X, Hao X, et al. High throughput screening atrazine chlorohydrolase mutants with enhanced activity through Haematococcus pluvialis expression system. Sheng Wu Gong Cheng Xue Bao. 2011;27(4): 620–628. [Article in Chinese].
- Díaz-Santos E, Vega M, Vila M, et al. Efficiency of different heterologous promoters in the unicellular microalga Chlamydomonas reinhardtii. Biotechnol Prog. 2013;29(2):319–328.
- Zhang R, Patena W, Armbruster U, et al. High-throughput genotyping of green algal mutants reveals random distribution of mutagenic insertion sites and endonucleolytic cleavage of transforming DNA. Plant Cell. 2014;26(4):1398–1409.
- Mirimin L, Roodt-Wilding R. Testing and validating a modified CTAB DNA extraction method to enable molecular parentage analysis of fertilized eggs and larvae of an emerging South African aquaculture species, the dusky kob Argyrosomus japonicus. J Fish Biol. 2015;86(3):1218–1223.
- Chang S, Chen W, Yang J. Another formula for calculating the gene change rate in real-time RT-PCR. Mol Biol Rep. 2009;36(8):2165–2168.
- Campos-Quevedo N, Rosales-Mendoza S, Paz-Maldonado LMT, et al. Production of milk-derived bioactive peptides as precursor chimeric proteins in chloroplasts of Chlamydomonas reinhardtii. Plant Cell Tissue Org Cult. 2013;113(2):217–225.
- Jones CG, Hare JD, Compton SJ. Measuring plant protein with the Bradford assay. J Chem Ecol. 1989;15:979–992.
- Li Q, Hong X, Chen XW, et al. Expression and characterization of recombinant cellulases from an isolated Klebsiella. Afr J Microbiol Res. 2015;9(38):2057–2066.
- Dekanski D, Spremo-Potparević B, Bajić V, et al. Acute toxicity study in mice of orally administrated TiO2 nanoparticles functionalized with caffeic acid. Food Chem Toxicol. 2018;115:42–48.
- Wang JY, Fang M, Boye A, et al. Interaction of microRNA-21/145 and Smad3 domain-specific phosphorylation in hepatocellular carcinoma. Oncotarget. 2017 May 9;8(49):84958–84973.
- Kim SN, Lee JH, Shin H, et al. Effects of in vitro-digested ginsenosides on lipid accumulation in 3T3-L1 adipocytes. Planta Med. 2009;75:596–601.
- EFSA. Panel on dietetic products, nutrition and allergies (NDA). Scientific opinion on bovine lactoferrin. EFSA J. 2012;10(7):2811.
- Rosales-Mendoza S, Paz-Maldonado LMT, Soria-Guerra RE. Chlamydomonas reinhardtii as a viable platform for the production of recombinant proteins: current status and perspectives. Plant Cell Rep. 2012;31:479–494.
- Shao N, Bock R. A codon-optimized luciferase from Gaussia princeps facilitates the in vivo monitoring of gene expression in the model alga Chlamydomonas reinhardtii. Curr Genet. 2008;53:381–388.
- Barahimipour R, Neupert J, Bock R. Efficient expression of nuclear transgenes in the green alga Chlamydomonas: synthesis of an HIV antigen and development of a new selectable marker. Plant Mol Biol. 2016;90(4–5):03–18.
- Eichler-Stahlberg A, Weisheit W, Ruecker O, et al. Strategies to facilitate transgene expression in Chlamydomonas reinhardtii. Planta. 2009;229(4):873–883.
- Dauvillée D, Delhaye S, Gruyer S, et al. Engineering the chloroplast targeted malarial vaccine antigens in Chlamydomonas starch granules. PLoS One. 2010;5(12):e15424.
- Rasala BA, Lee PA, Shen Z, et al. Robust expression and secretion of Xylanase1 in Chlamydomonas reinhardtii by fusion to a selection gene and processing with the FMDV 2A peptide. PLoS One. 2012;7(8):e43349.
- Cerven D, DeGeorge G, Bethell D. 28-Day repeated dose oral toxicity of recombinant human apo-lactoferrin or recombinant human lysozyme in rats. Regul Toxicol Pharmacol. 2008;51(2):162–167.
- Appel MJ, van Veen HA, Vietsch H, et al. Sub-chronic (13-week) oral toxicity study in rats with recombinant human lactoferrin produced in the milk of transgenic cows. Food Chem Toxicol. 2006;44(7):964–973.
- Neupert J, Shao N, Lu Y, et al. Genetic transformation of the model green alga Chlamydomonas reinhardtii. Methods Mol Biol. 2012;847:35–47.
- Barahimipour R, Strenkert D, Neupert J, et al. Dissecting the contributions of GC content and codon usage to gene expression in the model alga Chlamydomonas reinhardti. Plant J. 2015;84:704–717.
- Lauersen KJ, Berger H, Mussgnug JH, et al. Efficient recombinant protein production and secretion from nuclear transgenes in Chlamydomonas reinhardtii. J Biotechnol. 2013;167:101–110.