ABSTRACT
A facile and efficient method has been developed for the optical resolution of racemic jasmonic acid (JA) on a relatively large scale and was successfully utilized for the preparation of optically pure (+)-JA and (−)-JA. We indicated that (+)-JA has lower growth inhibitory activity than (−)-JA in the rice seedling growth test and confirmed in line with an earlier observation that their respective biologically-active forms, (+)-JA-Ile and (−)-JA-Ile, show comparable inhibitory activities. We compared the metabolism of (+)-JA and (−)-JA into (+)-JA-Ile and (−)-JA-Ile, respectively, in the JA-deficient rice cpm2, and found that the exogenously applied (+)-JA was metabolized to the corresponding Ile conjugate less efficiently as compared with (−)-JA. Such metabolic rate difference may cause a discrepancy between biological potencies of (+)-JA and (−)-JA in rice.
Abbreviations: FW: fresh weight; Ile: isoleucine; JA: jasmonic acid; JA-Ile: jasmonoyl-l-isoleucine; LC-ESI-MS/MS: liquid chromatography and electrospray ionization tandem mass spectrometry; MeJA: methyl jasmonate; OPDA: 12-oxophytodienoic acid
Graphical abstract
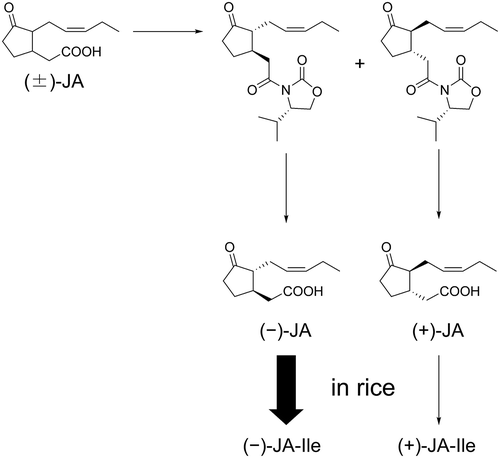
Facile optical resolution of (±)-JA to (+)-JA and (−)-JA, and their conversion to (+)-JA-Ile and (−)-JA-Ile.
Jasmonates are phytohormones that regulate the growth and development of plants and play crucial roles in plant defense against a variety of abiotic and biotic stresses [Citation1,Citation2]. They are biosynthesized from α-linolenic acid which is released from the chloroplast membrane lipids. In chloroplasts, α-linolenic acid is converted into 12-oxo-phytodienoic acid (OPDA) by sequential action of lipoxygenase, allene oxide synthase, and allene oxide cyclase. In the next step, OPDA moves into peroxisomes, where it is reduced by OPDA reductase to 8-[3-oxo-2-{(Z)-2-pentenyl}cyclopentyl]octanoic acid (OPC-8:0) and three subsequent rounds of β-oxidation yield (+)-7-iso-jasmonic acid ((+)-7-iso-JA). In the cytosol, (+)-7-iso-JA is converted by JAR1 (Jasmonate Resistant 1) into the biologically active form, (+)-7-iso-jasmonoyl-l-isoleucine ((+)-7-iso-JA-Ile). (+)-7-iso-JA spontaneously epimerizes to a more stable trans-isomer, (−)-JA (5), to form an equilibrated mixture of the two in the cells. The in vivo ratios of (+)-7-iso-JA to (−)-JA (5) have been shown to range from 35:65 to 20:80 [Citation3,Citation4], although such equilibrium is pH-dependent and hence should be fluctuating. Similar epimerization has also been observed between (+)-7-iso-JA-Ile and (−)-JA-Ile [Citation5].
In 1981, Yamane et al. attempted the optical resolution of racemic methyl jasmonate (MeJA, 1) to obtain a small amount of optically active unnatural (+)-MeJA [Citation6]. The authors found that (+)-MeJA showed considerably high inhibitory activity against the growth of rice seedlings, although the activity was lower than that of naturally-occurring (−)-MeJA [Citation6]. On the other hand, Jikumaru et al. reported that unnatural (+)-JA-Ile and natural (−)-JA-Ile showed similar inhibitory activity in the rice seedling test [Citation7]. More recently, Fonseca et al. have reported that (+)-JA-Ile is more active than (−)-JA-Ile in root-inhibition assays of Arabidopsis seedlings and protein pull down assays of COI1- jasmonate ZIM-domain (JAZ) interactions [Citation5]. In addition, Guranowski et al. have reported that Arabidopsis JAR1 (AtJAR1) is able to convert (+)-JA (6) to (+)-JA-Ile, although the yield is two orders of magnitude lower than that of (−)-JA (5) to (−)-JA-Ile [Citation8]. These results suggest that (+)-JA (6) can be expected to become bioactive after conversion to (+)-JA-Ile in rice. However, the metabolism of (+)-JA (6) in rice has not been thoroughly investigated so far.
In this study, we developed a facile and efficient method for optical resolution of racemic JA to obtain (+)-JA (6) and (−)-JA (5) on a relatively large scale, and assayed the growth inhibitory activities of (+)-JA, (−)-JA, and their Ile conjugates in rice seedlings to re-investigate the previously published results [Citation6,Citation7]. Furthermore we investigated the metabolic rates of (+)-JA (6) and (−)-JA (5) into their Ile conjugates in rice, and discussed why the growth inhibitory activity of (+)-JA is lower than that of (−)-JA.
Materials and methods
General experimental precedures
1H- and 13C-NMR were recorded on an ECA-500 spectrometer (JEOL) at 500 MHz and 125 MHz, respectively. Specific optical rotations were recorded on a DIP-300 polarimeter (JASCO, Hachioji, Japan).
Chemicals
Racemic MeJA (1) and [9,10-2H2]-JA were purchased from Wako Pure Chemical Co. Ltd (Osaka, Japan) and Tokyo Chemical Industry Co. Ltd (Tokyo, Japan), respectively. (+)-JA-Ile and (−)-JA-Ile were synthesized as previously described [Citation7]. [13C6]- (+)-JA-Ile and [13C6]-(−)-JA-Ile were similarly synthesized using [13C6]-Ile (Cambridge Isotope Laboratories Inc., Tewksbury, MA, USA) [Citation9].
(±)-JA (2)
Racemic MeJA (1) (2.24 g, 10.0 mmol) was dissolved in a mixture of THF (60 mL) and water (20 mL), and 1 M NaOH (20 mL) was added dropwise to the solution. After stirring overnight at room temperature, the reaction mixture was concentrated under reduced pressure, and the residue was acidified to pH 2 with 6 M HCl. The mixture was extracted three times with diethyl ether, and the combined extracts were washed with water and brine successively. After drying over anhydrous sodium sulfate, the solvent was evaporated to obtain (±)-JA (2) (2.03 g, 97% yield). This material was used for the next reaction without further purification.
N-((3S)-jasmonoyl)-(4S)-4-isopropyl-2-oxazolidinone (3) and n-((3R)-jasmonoyl)-(4S)-4-isopropyl-2-oxazolidinone (4)
A solution of 1.6 M n-butyllithum in n-hexane (1.36 mL, 2.00 mmol) was added to a solution of (S)-4-isopropyl-2-oxazolidione (0.258 g, 2.00 mmol) in THF (10 mL) at −78°C under argon with stirring, and the stirring was continued for 30 min at the same temperature. To this solution, a mixed acid anhydride suspension, which had been prepared by adding isobutyl chloroformate (0.300 g, 2.20 mmol) to a solution of N-methylmorpholine (0.222 mL, 2.20 mmol) and (±)-JA (2) (0.420 g, 2.00 mmol) in THF (5 mL) at −15°C with stirring, was added and stirred for 30 min at −78°C, and then for 2 h at 0°C. The reaction was stopped by adding saturated NH4Cl aq (2.5 mL) and the resultant reaction mixture was extracted three times with ethyl acetate. The combined organic layers were washed with water and saturated NaCl aq, and then dried over anhydrous Na2SO4. The solvent was evaporated under reduced pressure. The residue was purified by silica gel flash chromatography (2.6 × 15 cm) using ethyl acetate-n-hexane (3:7, v/v) as the eluent to isolate the fast-moving N-((3R)-jasmonoyl)-(4S)-4-isopropyl-2-oxazolidinone (4), and slow-moving N-((3S)-jasmonoyl)-(4S)-4-isopropyl-2-oxazolidinone (3). The Rf values of compounds 3 and 4 were 0.26 and 0.36, respectively, on thin layer chromatography under the following conditions: adsorbent, Silica gel 60 F254 (Merck, Darmstadt, Germany); solvent system, ethyl acetate-n-hexane (3: 7, v/v).Compound 3: 0.168 g, 22% yield. [α]D= +79.4 (c = 1.47, methanol). 1H NMR δ (CDCl3) 0.87 (3H, d, J = 6.9 Hz), 0.91 (3H, d, J = 7.0 Hz), 0.91 (3H, t, J = 7.7 Hz), 1.49 (1H, m), 1.95 (1H, m), 2.02 (2H, m), 2.10 (1H, m), 2.26 (1H, m), 2.34 (4H, m), 2.41 (1H, m), 3.08 (1H, dd, J = 17.0 Hz, J = 9.0 Hz), 3.17 (1H, dd, J = 17.0 Hz, J = 4.5 Hz), 4.20 (1H, dd, J = 9.2 Hz, J = 3.0 Hz), 4.27 (1H, t, J = 8.7 Hz), 4.43(1H, m), 5.26 (1H, m), 5.41 (1H, m). 13C-NMR δ (CDCl3) 14.27, 14.90, 18.12, 20.76, 25.69, 27.34, 28.62, 37.58, 37.87, 39.98, 54.16, 58.64, 63.62, 125.17, 134.17, 154.30, 171.88, 219.44. Compound 4: 0.081 g, 13% yield. [α]D = +28.6 (c = 1.73, methanol). 1H-NMR δ (CDCl3) 0.85 (3H, d, J = 6.8 Hz), 0.90 (3H, d, J = 6.8 Hz), 0.90 (3H, t, J = 7.5 Hz), 1.44 (1H, m), 1.93 (1H, m), 2.01 (2H, m), 2.09 (1H, m), 2.25 (1H, m), 2.35 (4H, m), 2.42 (1H, m), 2.92 (1H, dd, J = 17.1 Hz, J = 9.7 Hz), 3.28 (1H, dd, J = 17.1 Hz, J = 4.0 Hz), 4.20 (1H, dd, J = 9.1 Hz, J = 2.8 Hz), 4.27 (1H, t, J = 8.7 Hz), 4.43 (1H, m), 5.25 (1H, m), 5.41 (1H, m). 13C-NMR δ (CDCl3) 14.23, 14.80, 18.10, 20.73, 25.56, 27.52, 28.55, 37.24, 37.93, 40.31, 54.12, 58.58, 63.62, 125.13, 134.15, 154.24, 171.80, 219.36.
(−)-JA (5)
Compound 3 (0.147 g, 0.46 mmol) and lithium hydroxide monohydrate (0.0384 g, 0.91 mmol) were dissolved in a mixture of THF (7 mL) and water (2 mL). To this solution, 30% H2O2 aq (0.183 mL, 1.79 mmol) was added dropwise at 0°C. The mixture was stirred for 30 min at 0°C and then overnight at room temperature. The reaction mixture was washed with dichloromethane, and acidified to pH 2 with 1 M HCl. The solution was extracted three times with diethyl ether, and the combined ether layers were washed with water and brine. The organic layer was dried over anhydrous Na2SO4. The product was purified by silica gel flash chromatography (1.0 × 15 cm) eluted with ethyl acetate-n-hexane (4:6, v/v) followed by solvent evaporation to obtain 5 (39.1 mg, 40% yield). [α]D = -88.2 (25°C, c = 0.820, methanol, Lit. -81.4 13). 1H NMR δ (CDCl3) 0.94 (3H, t, J = 7.6 Hz), 1.51 (1H, m), 1.91 (1H, m), 2.03 (2H, m), 2.11 (H, m), 2.31 (3H, m,), 2.35 (3H, m), 2.75 (1H, m), 5.24 (1H, m), 5.44 (1H, m), 8.26 (1H, br). 13C-NMR δ (CDCl3) 14.28, 20.76, 25.63, 27.34, 37.92, 37.92, 38.89, 54.04, 124.95, 134.42, 178.20, 219.44.
(+)-JA (6)
Compound 4 (0.087 g, 0.27 mmol) was treated in the same manner as in the subsection (−)-JA (5) to obtain 6 (41.0 mg, 72% yield). [α]D = +85.8 (25°C, c = 0.782, methanol, Lit. +84.7 13). 1H-NMR δ (CDCl3) 0.94 (3H, t, J = 7.6 Hz), 1.51 (1H, m), 1.91 (1H, m), 2.03 (2H, m), 2.11 (1H, m), 2.31 (3H, m,), 2.35 (3H, m), 2.75 (1H, m), 5.24 (1H, m), 5.44 (1H, m), 8.26 (1H, br). 13C-NMR δ (CDCl3) 14.28, 20.76, 25.63, 27.34, 37.92, 37.92, 38.89, 54.04, 124.95, 134.42, 178.20, 219.44.
Plant materials
In this study, we used Oryza sativa L. “Nihonmasari” as the wild-type rice. The cpm2 mutant was isolated from γ-ray-mutagenized lines of Nihonmasari as described previously [Citation9]. Rice plants were grown under natural daylight in a glasshouse.
Chiral analysis of JA
Optically active jasmonates were subjected to combined liquid chromatography and electrospray ionization tandem mass spectrometry (LC-ESI-MS/MS) comprising a quadrupole tandem mass spectrometer (Agilent 6460 Triple Quadrupole mass spectrometer) with an electrospray ion source and an Agilent 1200 separation module equipped with a Sumichiral OA-7500 column (length 250 mm, diameter 2 mm; Sumika Chemical Analysis Service, Tokyo, Japan). The mobile phases used were 0.05% acetic acid in water (solvent A) and 0.05% acetic acid in acetonitrile (solvent B). Elution was conducted using a linear gradient of solvent B from 3 to 73% over 22 min at a flow rate of 0.2 mL min−1. Retention times of (−)-JA (5) and (+)-JA (6) were 18.2 and 18.9 min, respectively. The source gas temperature was set to 300°C at a flow rate of 12 L min−1. The nebulizer gas pressure was set to 40 psi. Sheath gas temperature was 350°C at a flow rate of 12 L min−1. Capillary and nozzle voltages were set to 3000 and 500 V, respectively. Analysis was conducted in negative ion mode and quantification was performed by multiple reaction monitoring. The precursor-to-product transition was monitored for m/z 209/59.
Growth inhibition assay of rice
The growth inhibition assay was carried out according to the micro-drop method at 30°C under continuous white light using 5-day-old wild-type rice seedling, as previously described [Citation7]. One μL aliquot of 0, 0.2, 2, 5, 10, or 20 nmol of JA or JA-Ile dissolved in 50% (v/v) acetone aq was applied to the surface of the coleoptile. After 4-day incubation, the lengths of the second leaf sheaths were measured. Five to six plants were used for each sample.
Comparison of the metabolic rate of (+)-JA (6) into (+)-JA-Ile with that of (-)-JA (5) into (-)-JA-Ile in rice
The leaf explants (3 cm long) were excised from the fully expanded youngest leaves of 6-week-old rice plants of cpm2, and floated on distilled water in covered Petri dishes at 25°C under white fluorescent light (918 mmol m−2 s−1) for a day. The leaf samples were then floated on 250 μM of (+)-JA (6) or (−)-JA (5) solution in covered Petri dishes at 25°C under white fluorescent light. After 2 h of incubation, the leaf samples (about 30 mg fresh weight) were homogenized and suspended in 2 mL 80% (v/v) methanol aq prior to adding 10 ng each of [13C6]-(+)-JA-Ile and [13C6]-(−)-JA-Ile as internal standards. Five μL aliquots from samples were diluted 100 times with 50% (v/v) methanol aq and then 5 ng of [2H2]-(±)-JA was added as an internal standard to the diluted solution, which was subjected to LC-ESI-MS/MS using a Sumichiral OA-7500 column for chiral analysis of JA as described in the subsection 2.8. The rest of the leaf extracts were purified using a Bond Elut C18 (100 mg, 3 mL; Agilent Technologies, Santa Clara, CA, USA) and subjected to LC-ESI-MS/MS as described previously [Citation10] for the quantification of (+)-JA-Ile and (−)-JA-Ile.
Results and discussion
Preparation of optically active (−)-JA (5) and (+)-JA (6)
As shown in , racemic MeJA (1) was hydrolyzed to give racemic JA (2), which was then coupled with (S)-4-isopropyl-2-oxazolidinone to give a mixture of diastereomeric isopropyloxazolidinone derivatives [Citation11]. The mixture was chromatographed on a silica gel column to easily separate 3 and 4. Compounds 3 and 4 were hydrolyzed with aq. lithium hydroperoxide [Citation12] and yielded optically active 5 and 6, respectively. The optical purities of 5 ([α]D = −88.2 (25°C, c = 0.820, methanol), Lit. −81.4 (23°C, c = 0.44, methanol)) and 6 ([α]D = +85.8 (25°C, c = 0.782, methanol), Lit. +84.7 (23°C, c = 0.50, methanol)) were higher than the previously reported values [Citation13]. Upon negative ion mode LC-ESI-MS/MS analysis using a chiral column, m/z 209/59 transitions were observed in 5 and 6 as single peaks with different retention times (), indicating that 5 and 6 were optically pure and suitable for evaluation of their biological activity. In addition, we also derivatized JAs by using 4-phenyl-2-oxazolidinone and 4-benzyl-2-oxazolidinone. The product yields and silica gel-TLC separations of these derivatives were comparable to those of isopropyloxazolidinone derivatives (data not shown). These derivatives may be used to prepare optically active JAs although further investigation was not attempted.
Figure 1. Chiral analysis of optically-active jasmonates.
Each optically active jasmonate (200 pg) was subjected to combined liquid chromatography and electrospray ionization tandem mass spectrometry (LC-ESI-MS/MS) with a chiral column. The precursor-to-product transition was monitored for m/z 209/59.
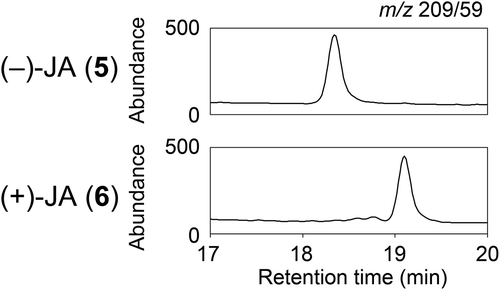
To date several methods have been reported for the optical resolution of racemic jasmonates. However, most of these methods involve rather laborious procedures such as a preparative HPLC separation of diastereomeric ketals with (−)-2,3-butandiol [Citation6], separation of diastereomeric mandelyl esters by repeated silica gel chromatography followed by recrystallization [Citation13], and lipase-catalyzed resolution of a racemic JA derivative [Citation14]. A patent has been obtained for simple optical resolution using fractional crystallization of diastereomeric salts with optically active 1-p-tolylethylamine, but the optical purities of the products were insufficient [Citation15]. In contrast, our current method to prepare optically active JAs () has advantages such as simplicity and ease over the earlier methods. It is especially advantageous that the 4-substituted oxazolidinone diastereomers prepared from racemic JA (2) are easily separable by conventional chromatography and hence this method can be applied for a relatively large-scale preparation.
Rice growth inhibitory activities of (+)-JA (6), (−)-JA (5), and their Ile conjugates
We next assayed the inhibitory effects of (+)-JA (6) and (−)-JA (5) on the elongation of the rice second leaf sheath. The exogenous application of (−)-JA (5) caused the growth inhibition of the second leaf sheath in a dose-dependent manner. (+)-JA (6) was also inhibitory, however, its activity was significantly lower than that of (−)-JA (5) ()). This result parallels with the earlier bioassay results obtained for methyl esters of (+)-MeJA and (−)-MeJA by Yamane et al. [Citation6].
Figure 2. Inhibitory effects of jasmonic acid (JA) and jasmonoyl-l-isoleucine (JA-Ile) on the growth of the second leaf sheath of rice seedlings (Oryza sativa L. “Nihonmasari”).
Rice seedlings (5-day old) were treated with 0, 0.2, 2, 5, 10, or 20 nmol of JA (a) or JA-Ile (b). After 4-day incubation, the lengths of the second leaf sheaths were measured. Values indicate the relative length of the second leaf sheath of rice seedlings treated with each isomer of JA (a) or JA-Ile (b) (n = 5 or 6). Values are normalized to the length of the second leaf sheath of rice seedlings without JA or JA-Ile treatment. Bars indicate standard errors of the means. Statistically different data groups are indicated by different letters (P < 0.05 by one-way ANOVA with a Tukey-Kramer’s post hoc test).
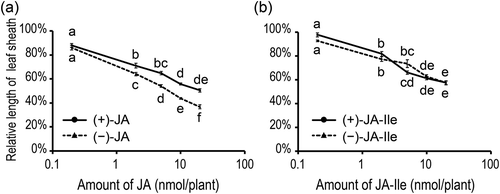
In contrast, the inhibitory activities of (+)-JA-Ile and (−)-JA-Ile in the rice test were indicated to be at the same levels ()) in line with the earlier observation by Jikumaru et al. [Citation7]. In Arabidopsis roots, (+)-JA-Ile has been reported to be more inhibitory than (−)-JA-Ile [Citation5], suggesting that action mechanisms of jasmonates may differ with plant species or tissues.
Metabolic rates of (+)-JA and (−)-JA into respective Ile conjugates in rice
JA becomes bioactive after conversion into JA-Ile in Arabidopsis and rice [Citation16–Citation19]. (+)-JA is unnatural and is converted into putative bioactive (+)-JA-Ile in Arabidopsis [Citation8]. To investigate why the growth inhibitory activity of (+)-JA is lower than that of (−)-JA in rice although (+)-JA-Ile and (−)-JA-Ile have equivalent inhibitory activities, we compared the metabolic rate of (+)-JA into (+)-JA-Ile with that of (−)-JA into (−)-JA-Ile in the JA-deficient rice mutant cpm2 [Citation9]. We first confirmed that JA and JA-Ile were undetectable in leaf explants of cpm2. Leaf explants (3-cm long) of the cpm2 mutant were then treated with 250 μM of (+)-JA (5) or (−)-JA (6) for 2 h prior to extracting with 80% methanol. The extracts were spiked with [2H2]-JA and [13C6]-JA-Ile as internal standards, and subjected to LC-ESI-MS/MS analysis. As shown in , the (+)-JA (6)-treated leaf explants contained 335.5 nmol g−1 fresh weight (FW) of (+)-JA (6) and 0.23 nmol g−1 FW of (+)-JA-Ile whereas the (−)-JA (5)-treated leaf explants contained 217.0 nmol g−1 FW of (−)-JA (5) and 3.2 nmol g−1 FW of (−)-JA-Ile. These results indicated that the ratio of (+)-JA-Ile to (+)-JA was about 20 times lower than that of (−)-JA-Ile to (−)-JA. This finding indicates that (+)-JA (6) was metabolized to (+)-JA-Ile far less efficiently than the conversion of (−)-JA (5) to (−)-JA-Ile in rice leaves and hence (+)-JA (6) may have lower inhibitory activity in rice tissues compared with (−)-JA (5).
Figure 3. Bioconversion of jasmonic acid (JA) to jasmonoyl-l-isoleucine (JA-Ile) in rice leaves.
Values indicate the amount of JA and JA-Ile in cpm2 leaf explants treated with 250 μM (−)-JA or (+)-JA (n = 3); bars indicate standard errors of the means. The inset shows enlargement of the JA-Ile accumulation. The cpm2 mutant was JA-deficient and neither JA nor JA-Ile was detected in the control tissues. Statistical analysis was conducted using a Welch’s test. Statistically different data are indicated by an asterisk (*, P < 0.05). n.s.: not significant.
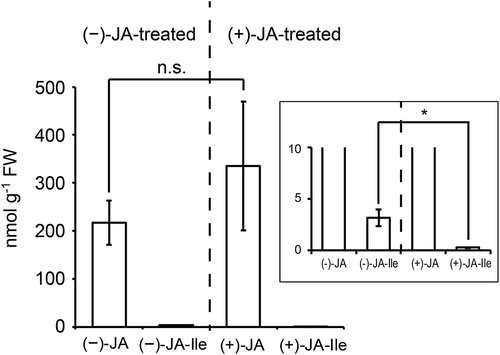
Conclusions
In this study, we succeeded in the facile optical resolution of racemic JA and were able to obtain optically pure (+)-JA (6) and (−)-JA (5) on a relatively large scale. Using such (+)-JA (6) and (−)-JA (5), we confirmed that (+)-JA (6) is considerably inhibitory in the rice second sheath elongation but its activity is significantly lower than that of (−)-JA (5). It was also confirmed that (+)-JA-Ile and (−)-JA-Ile have equivalent activities in the rice test. Furthermore we found that the metabolic rate of (+)-JA (6) into (+)-JA-Ile was about 20 times lower than that of (−)-JA (5) into (−)-JA-Ile in rice, and have proposed that this lower metabolic rate of (+)-JA (6) may be a primary reason why (+)-JA (6) is less active than (−)-JA (5) in rice.
Authors contribution
Koji Miyamoto, Tomoharu Matsumoto and Kenichi Uchida carried out the experiments. Takao Yokota, Emi Yumoto and Tomoko Sakazawa performed LC-ESI-MS/MS analyses. Koji Miyamoto, Hisakazu Yamane and Kenichi Uchida designed the project and prepared the manuscript.
Acknowledgments
We are grateful to Prof. Moritoshi Iino (Osaka City University) for providing seeds of the cpm2 mutant.
Disclosure statement
No potential conflict of interest was reported by the authors.
Additional information
Funding
References
- Turner JG, Ellis C, Devoto A. The jasmonate signal pathway. Plant Cell. 2002;14:153–164.
- Farmer EE, Alméras E, Krishnamurthy V. Jasmonates and related oxylipins in plant responses to pathogenesis and herbivory. Curr Opin Plant Biol. 2003;6:372–378.
- Miersch O, Meyer A, Vorkefeld S, et al. Occurrence of (+)-7-iso-jasmonic acid in Vicia faba L. and its biological activity. J Plant Growth Regul. 1986;5:91–100.
- Schulze B, Lauchli R, Sonwa MM, et al. Profiling of structurally labile oxylipins in plants by in situ derivatization with pentafluorobenzyl hydroxylamine. Anal Biochem. 2006;348:269–283.
- Fonseca S, Chini A, Hamberg M, et al. (+)-7-iso-Jasmonoyl-l-isoleucine is the endogenous bioactive jasmonate. Nat Chem Biol. 2009;5:344–350.
- Yamane H, Takahashi N, Ueda U-I, et al. Resolution of (±)-methyl jasmonate by high performance liquid chromatography and the inhibitory effect of (+)-enantiomer on the growth of rice seedlings. Agric Biol Chem. 1981;45:1709–1711.
- Jikumaru Y, Asami T, Seto H, et al. Preparation and biological activity of molecular probes to identify and analyze jasmonic acid-binding proteins. Biosci Biotechnol Biochem. 2004;68:1461–1466.
- Guranowski A, Miersch O, Staswick PE, et al. Substrate specificity and products of side-reactions catalyzed by jasmonate: aminoacid synthetase (JAR1). FEBS Lett. 2007;581:815–820.
- Riemann M, Haga K, Shimizu T, et al. Identification of rice allene oxide cyclase mutants and the function of jasmonate for defence against Magnaporthe oryzae. Plant J. 2013;74:226–238.
- Miyamoto K, Enda I, Okada T, et al. Jasmonoyl-l-isoleucine is required for the production of a flavonoid phytoalexin but not diterpenoid phytoalexins in ultraviolet-irradiated rice leaves. Biosci Biotechnol Biochem. 2016;80:1934–1938.
- Evans DA, Bartroli J, Shih TL. Enantioselective aldol condensations. 2. Erythro-selective chiral aldol condensations via boron enolates. J Amer Chem Soc. 1981;103:2127–2129.
- Evans DA, Britton TC, Ellman JA. Contrasteric carboximide hydrolysis with lithium hydroperoxide. Tetrahedron Lett. 1987;28:6141–6144.
- Kramell R, Schneider G, Schmidt J, et al. Optical resolution of racemic jasmonic acid by separation of diastereomeric mandelyl esters. Z Naturforsch B. 1990;45:377–381.
- Kiyota H, Higashi E, Koike T, et al. Lipase-catalyzed preparation of both enantiomers of methyl jasmonate. Tetrahedron-Asymmetr. 2001;12:1035–1038.
- Nohira H, Shiyou Y, Nippon Zeon Co. Ltd., Optical resolution of jasmonic acid and dihydrojasmonic acid. JP 1991-261743. 1991 Nov 21.
- Staswick PE, Tiryaki I. The oxylipin signal jasmonic acid is activated by an enzyme that conjugates it to isoleucine in Arabidopsis. Plant Cell. 2004;16:2117–2127.
- Riemann M, Riemann M, Takano M. Rice JASMONATE RESISTANT 1 is involved in phytochrome and jasmonate signaling. Plant Cell Environ. 2008;31:783–792.
- Shimizu T, Miyamoto K, Miyamoto K, et al. OsJAR1 contributes mainly to biosynthesis of the stress-induced jasmonoyl-isoleucine involved in defense responses in rice. Biosci Biotechnol Biochem. 2013;77(7):1556–1564.
- Chini A, Fonseca S, Fernandez G, et al. The JAZ family of repressors is the missing link in jasmonate signalling. Nature. 2007;448:666–671.