ABSTRACT
Adenosine kinase (ADO1)-deficient mutants can be obtained from cordycepin-resistant strains, and the disruption of ADO1 causes S-adenosylmethionine (SAM) accumulation. To breed a high-SAM-accumulating yeast strain without genetic manipulation for industrial purposes, we bred a cordycepin-resistant strain using sake yeast kyokai No. 9 as the parent strain with a mutation in adenosine kinase (ADO1) and acquired high-SAM-accumulating strain. In the bred strain (NY9-10), a single mutation (T258I) was present in the ADO1, and this mutation site is an ATP binding site and is highly conserved during evolution. Moreover, it was suggested that high accumulation of SAM and cordycepin resistance in NY9-10 was due to functional deficiency of ADO1 by this mutation. This strain is not a genetically-modified organism and can be employed for use in the food and medicine industry such as mass production and sake making.
Graphical Abstract
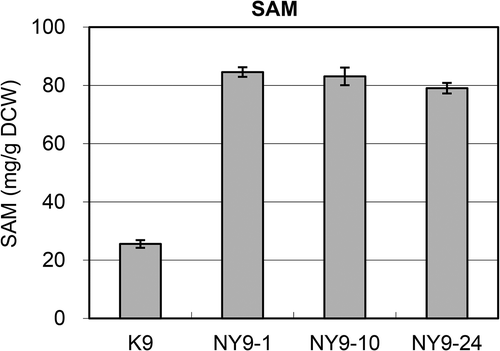
We bred a cordycepin-resistant strain using sake yeast with a mutation in the ADO1 gene and obtained high-SAM-accumulating strains.
The nutrient S-adenosylmethionine (SAM) is synthesized by transfer of the adenosyl moiety of adenosine triphosphate (ATP) to the sulfur atom of methionine. SAM is a key regulatory compound in various biological reactions and functions as a major donor of methyl groups in transmethylation. Shiozaki et al. reported intracellular SAM accumulation in various microorganisms and found that yeasts, especially sake yeast, accumulated more SAM than molds, basidiomycetes, bacteria, actinomycetes, and lactic acid bacteria [Citation1]. We previously reported that the sake yeast YHR032W/ERC1 haplotype contributed to SAM accumulation in sake yeast strains and led to enhanced stress resistance and longevity [Citation2,Citation3]. However, the physiological significance and exact mechanism underlying SAM accumulation in yeasts, especially sake yeast, remain unclear.
In our previous work, to breed novel sake yeast strains, we screened for methionine-resistant sake yeast strains and identified ADO1, which encodes adenosine kinase, as one of the factors involved in high-SAM accumulation [Citation4]. However, because this strain is a genetically-modified organism, industrial use continues to be difficult. Therefore, in the present study, the ado1 mutant (SAM accumulation strain) was bred from sake yeast by a mutation treatment (ultraviolet irradiation) that is not based on genetic recombination. Thus, we successfully acquired a high-SAM-accumulating strain suitable for use in the food and medicine industry.
It has been previously reported that one of cordycepin-resistant strains of Saccharomyces cerevisiae was adenosine kinase-deficient mutant [Citation5], and the disruption of ADO1 affects the utilization of SAM as a purine source [Citation6]. Furthermore, we previously reported that the disruption of ADO1 caused SAM accumulation [Citation4]. Accordingly, we focused on cordycepin resistance indicated by the ADO1 disrupted strain to breed a new high-SAM-accumulating strain. Cordycepin (3ʹ-deoxyadenosine), which is a component unique to Cordyceps sinensis that is used as a nourishing tonic, induces immature termination upon RNA synthesis as an inhibitor of RNA polymerase and protein kinase. The deletion of ADO1 results in resistance to the toxic adenosine analogue cordycepin [Citation6]. Therefore, it was assumed that a new high-SAM-accumulating yeast strain for industrial use could be bred by acquiring a cordycepin-resistant strain not based on genetic manipulation from sake yeast. In fact, we performed breeding using diploid sake yeast kyokai No. 9 (K9) and No. 7 (K7). However, in this report, we describe the result of breeding using K9.
Materials and methods
Strains and plasmids
The K7H893Δado1 strain (haploid sake yeast; MAT a Δado1::kanMX4) used in this study was as described previously [Citation4]. To construct the pAUR101-K7ADO1 plasmids, the ADO1 gene was amplified by high-fidelity polymerase chain reaction (PCR) using KOD-Plus-Neo DNA polymerase (Toyobo Co., Ltd., Osaka, Japan) and genomic DNA of K7 as templates with the following primers: pADO-infusionF2 (5′-GCAGGTCGACTCTAGTTCGTCAGTTGGAGCACCATGTGTC-3′) and pADO-infusionR (5′-ACCCGGGGATCCTCTAGTGCCATTAATGCCCCAGTATGAGATGC-3′). The nucleotide sequence of ADO1 gene in K7 was identical to that in K9. To construct the pAUR101-NY9-10ADO1 plasmids, the ADO1 gene was amplified by high-fidelity PCR using KOD-Plus-Neo DNA polymerase and genomic DNA of NY9-10 as templates with the following primers: pADO-infusionF2 and pADO-infusionR. The vector pAUR101 is an E. coli-yeast shuttle vector for chromosomal integration. pAUR101 was linearized by cutting at a single restriction enzyme site (Stu I) on the AUR1-C gene and was efficiently integrated in the aur1 gene region on the host yeast chromosome by homologous recombination. Each amplified ADO1 gene was cloned into the Xba I site of the pAUR101 plasmid using an In-Fusion HD Cloning Kit (Takara Bio USA, Inc., CA, USA). Positive transformants in the NY9-10 and K7H893Δado1 background were selected on plates of YPD medium containing Aureobasidin A (1.0 and 0.5 μg/mL, respectively), which consisted of 1% yeast extract, 2% peptone, 2% D-glucose, 2% agar, and Aureobasidin A (Takara Bio USA, Inc., CA, USA).
Culture media
Yeasts were cultured on YPD, YPD containing cordycepin (FUJIFILM Wako Pure Chem. Corp., Tokyo, Japan), and O medium (glucose 5%, peptone 1%, yeast extract 0.5%, KH2PO4 0.4%, K2HPO4 0.2%, MgSO4K7H2O 0.05%, and l-methionine 0.15%).
Breeding of cordycepin-resistant sake yeast strains
The K9 strain was treated with ultraviolet (UV) light on a clean bench (approximately 10% viability) and subcultured in YPD medium containing cordycepin (600 μg/mL). The medium used for passage culture was replaced with fresh YPD medium containing cordycepin after every passage culture. The culture time from primary to tertiary passage culture is three days; fourth to sixth passage culture is one day at 30ºC. We then plated yeast suspension on YPD plate and obtained 25 cordycepin-resistant sake yeast strains.
Determination of the SAM and S-adenosylhomocysteine (SAH) content
The SAM and SAH content were determined by capillary electrophoresis [Citation4]. Cultivation was started by inoculation of YPD or O medium to achieve a starting optical density at 660 nm (OD660) of 0.1. Yeast strains were grown at 30°C with shaking. The cells were harvested, washed twice with MilliQ grade water (Merck Millipore, Darmstadt, Germany), suspended in 1 mL of 10% perchloric acid solution, extracted for 1 h at 30°C, centrifuged, and filtered using Ekicrodisc 3 pore size (0.45 mm; Nippon Genetics Co., Ltd., Tokyo, Japan). The supernatant was subjected to capillary electrophoresis using high-performance capillary electrophoresis standard capillaries (75 mm inner diameter (id), 72 cm light 2/PK, Agilent Technologies, Inc., CA, USA) in the Agilent Capillary Electrophoresis System G1600A (Agilent Technologies, Inc.). The electrophoresis buffer comprised 40 mM sodium phosphate with pH 2.5.
DNA sequence analysis
Yeast genomic DNA was extracted using a Dr. GenTLE (from yeast) high-recovery kit (Takara Bio, Inc., Shiga, Japan). The sequence analysis was performed with the following primers: ADO1U45F (5′-GCAGGGTAGAACCAAAGATAACAGCAAAAGAAAGAGCAAGCAATT-3′), ADO1D45R (5′- GAACACGTAGAGTATATTTTATTTCTATTATATTGTAAGAAGAAT-3′).
Pyrithiamine resistance test
Yeast strains were spotted on YNB containing pyrithiamine medium (0, 3.75, 7.5, and 15 μM, Sigma, St. Louis, MO, USA). Spotted yeast strains were grown for 3 days at 30°C.
Cordycepin resistance test
Ten-fold serial dilutions of indicated strains were spotted on YNB medium supplemented with the indicated concentration of cordycepin (0 and 400 μg/mL, FUJIFILM Wako Pure Chem. Corp., Tokyo, Japan). The spotted yeast strains were grown for 3 days at 30ºC.
Determination of the homocysteine (Hcy) content
The Hcy content of the strains was determined using the Homocysteine high-performance liquid chromatography (HPLC) Kit (Immundiagnostik AG, Bensheim, Germany) [Citation4]. Yeast strains were cultivated at 30°C in YPD medium (starting OD660, 0.1). The cells were harvested, washed, and freeze-dried. Lyophilized yeast samples (20 mg) were suspended in 8 mM hydrochloric acid and disrupted by vortexing with 0.5 mm glass beads (5 times × 30 s). After centrifugation, the supernatant was collected. An aliquot of supernatant was subjected to fluorescence labeling and analyzed by HPLC.
Small-scale sake making test
A small-scale sake making test with three mashing steps was carried out as previously described [Citation7] using 400 g total rice. Precultured yeast (1.7 × 109 cells) was added to a mixture of 114 mL water, 240 μL 90% lactic acid, and 20 g dried koji (Tokushima Seikiku Co., Osaka, Japan), and incubated overnight at 15°C. The next day, 15 mL water and 50 g pre-gelatinized rice (Tokushima Seikiku Co., Osaka, Japan) were added (Soe). Two days later, 187 mL water, 20 g dried koji, and 110 g pre-gelatinized rice were added and the mash was fermented at 9°C (Naka). The following day, 316 mL water, 40 g dried koji, and 160 g pre-gelatinized rice were added and the mash was fermented at 7°C (Tome). The fermentation temperature was increased by 1.0 °C/day up to a maximum temperature of 15°C and then maintained for 4 days. Next, the temperature was then decreased by 1.0 °C/day to 9°C. Further, 19 days after Tome, 160 mL of mash was centrifuged and the supernatant was analyzed as sake. Analysis of total CO2 output (g), nihonshu-do, ethanol concentration (%), acidity, amino acidity, and methylene blue (MB) dyeing rate (%) in sake making were examined as previously described [Citation8].
Results and discussion
Cordycepin resistance in sake yeast
We initially examined whether ADO1-disrupted sake yeast strains exhibited cordycepin resistance. Cell growth of the haploid sake yeast (K7H893) strain was inhibited with an increase in cordycepin concentration (0–200 μg/mL); however, the ADO1-disrupted strain (K7H893Δado1) exhibited cordycepin resistance (200 μg/mL). Therefore, the use of cordycepin was confirmed as an effective strategy for breeding high-SAM-accumulating strains (ADO1 mutants).
Breeding of cordycepin-resistant sake yeast strains accumulating high levels of S-adenosylmethionine
Next, we evaluated the effect of cordycepin concentration on growth in the diploid sake yeast K9. Cell growth in K9 was remarkably delayed at 120–200 μg/mL cordycepin and completely inhibited at ≥500 μg/mL cordycepin during three-day culture. Accordingly, to obtain a cordycepin-resistant strain derived from K9, we selected a cordycepin concentration of 600 μg/mL. By mutation treatment using UV, cell growth was not observed during the primary and secondary passage culture; however, slight cell growth was observed after the tertiary passage culture. Furthermore, subculture was repeated three times, and we plated the bacterial suspension on YPD plate and obtained 25 cordycepin-resistant sake yeast strains (). As breeding was performed in a liquid medium, it was expected that yeast cells exhibiting good cordycepin resistance and proliferation were preferentially selected.
Next, we examined the SAM content in the obtained cordycepin-resistant strain. All single colonies (NY9-1–25) obtained from K9 had a SAM content higher than that of the parent strain after incubation at 30°C in YPD medium for 24 h. Particularly, from the point of view of the SAM content among them, three strains (NY9-1, 10, and 24) showed ≥10.0 mg per 1 g of dry cell weight (/g DCW), which was more than 20 times that of the corresponding parent strain. We then determined the SAM content in bred strains cultured in O medium, which is known as a high-SAM accumulation medium. After incubation at 30°C in O medium for 24 h, the SAM content in NY9-1 and −10, which are the strains derived from K9, was ≥80 mg/g DCW; the SAM content exceeded 8% of DCW, which was approximately four times greater than that observed in the parent strain (25.6 mg/g DCW). Because breeding did not involve genetic modification, this strain could have industrial applications (Patent No. P5641192). Therefore, we then focused on the NY9-10 strain, which accumulated the highest level of SAM among 25 isolates in the YPD and O media ().
Function of Ado1p in NY9-10
To elucidate the SAM accumulation mechanism and other properties of the bred strain (high-SAM accumulation strain), we examined the sequence of its ADO1 gene. Adenosine kinase catalyzes the transfer of phosphoryl group from ATP to adenosine to yield adenosine monophosphate (AMP) and adenosine diphosphate. The adenosine kinase of budding yeast encoded by ADO1 has the kinase catalytic domain, active center (aspartate: D293), 17 substrate binding site (asparagine: N10, leucine: L12, D14, alanine: A34, glycine: G63, G64, A65, N68, cysteine 121, L132, threonine: T134, L136, and phenylalanine: F165, F196, N289, G290, and D293) and 13 ATP binding site (T258, G260, valine: V261, T264, V277, proline 279, and isoleucine: I285, T288, A291, G292, F295, A320, and I324) ((a)). In NY9-10, threonine 258 was substituted by isoleucine in the homozygous diploid ADO1 gene compared with K9 ((a)). This mutation site (T258) is an ATP binding site and is highly conserved during evolution ((a, b)). Therefore, decrease or inactivation of adenosine kinase activity owing to this mutation is a potential cause of high-SAM accumulation in the bred strain (NY9).
Figure 2. Structure of Ado1p. (a) Comparison between sake yeast strains K9 Ado1p (K9Ado1p) and NY9-10 Ado1p (NY9-10Ado1p). Rectangles with light shading, filled circles, arrows, and triangles represent the adenosine kinase domain, the positions of active center, substrate binding site, and ATP binding site, respectively. Open circles indicate the positions of single amino acid substitutions. The nucleotide and amino acid sequences surrounding the mutation in NY9-10 (T258I) are also shown. (b) Amino acid sequences of adenosine kinase surrounding the mutation in NY9-10 is aligned with Saccharomyces cerevisiae Sc S288C (UniProtKB accession no. P47143), Sc K7 (SYGD accession no. SYK7_040281), Arabidopsis thaliana At (Q9SF85), Mus musculus Mm (P55264), Rattus norvegicus Rt (Q64640), and Homo sapiens Hs (P55263). The nucleotide sequences of Ado1p in K9 and NY9-10 were examined in this study. Identities and similarities are highlighted in black and gray boxes, respectively.
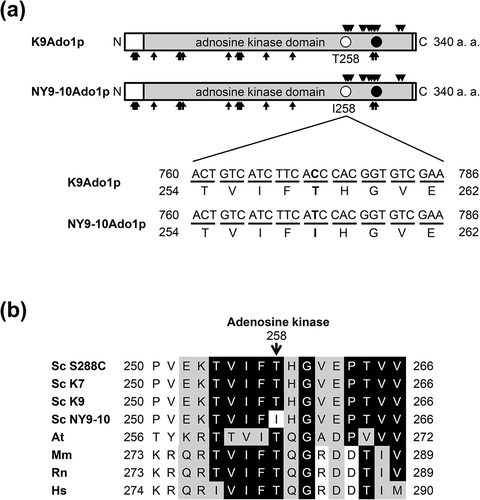
To verify this possibility, we constructed the plasmid pAUR101-K7ADO1 by subcloning ADO1 gene of sake yeast K7 and pAUR101-NY9-10ADO1 by subcloning ADO1 mutant gene of the bred strain NY9-10, and integrated these plasmids into the K7H893Δado1 strain. We then determined the SAM content and examined cordycepin resistance using transformants (K7H893Δado1/K7ADO1 or NY9-10ADO1) integrated these plasmids. The nucleotide sequence of ADO1 gene in K7 was identical to that in K9 (data not shown). As shown in (a) and , the SAM content and cordycepin resistance in the transformant integrated into the plasmid pAUR101-K7ADO1 in K7H893Δado1 decreased to nearly the same level relative to K7H893, whereas the SAM content and cordycepin resistance in transformant integrated into the plasmid pAUR101-NY9-10ADO1 in K7H893Δado1 was unchanged relative to K7H893Δado1. These results indicated that the loss-of-function of Ado1p in NY9-10 was responsible for this mutation (T258I).
Figure 3. The SAM content and cordycepin resistance using transformants integrated various ADO1 genes into the K7H893Δado1 and NY9-10 strains. (a and c) Culture was initiated by inoculation of YPD medium to obtain a starting optical density at 660 nm of approximately 0.1. The transformants were cultivated for 24 h at 30°C in O medium, and determined the SAM content. Values are presented as mean ± standard deviation for three independent experiments; *P < 0.01 (t test, two-tailed, parametric unequal variance). (b and d) Ten-fold serial dilutions of the indicated strains were spotted on YNB medium supplemented with the indicated concentration of cordycepin and incubated at 30ºC for 2 days.
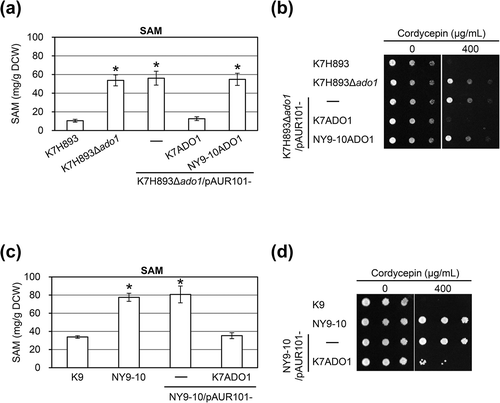
Based on the above results, we next integrated pAUR101-K7ADO1 into the NY9-10 strain and examined the SAM content and cordyceptin resistance of the transofrmant NY9-10/pAUR101-K7ADO1. The SAM content in the transformant NY9-10/pAUR101-K7ADO1 (having two copies of mutated Ado1p-T258I and one copy of wild-type Ado1p) decreased to nearly the same level relative to K9 ((c)). However, cordycepin resistance in the transformant NY9-10/pAUR101-K7ADO1 was partially suppressed relative to K9 ((d)).
Next, we examined a pyrithiamine resistance in bred strains. It was previously reported that cordycepin-resistant strains can be obtained by acquiring mutants of adenosine kinase-deficient or thiamine transporters exhibiting pyrithiamine resistance [Citation5,Citation9]. As a result, the cordycepin-resistant strain acquired in this study did not exhibit pyrithiamine resistance, indicating that the phenotype of the bred cordycepin-resistant strains was not due to mutation of pyrithiamine transporters.
These results suggested that high-SAM accumulation and cordycepin resistance in NY9-10 is due to the functional deficiency of ADO1. However, cordycepin resistance in NY9-10 may be due to the influence of the dosage effect by semi-dominant mutation (T258I) in NY9-10. Alternatively, cordycepin resistance in NY9-10 may be not only due to the functional deficiency of ADO1 because this bred strain had various mutations due to UV treatment. The mechanism responsible for these phenomena remains unclear.
The SAM and various components related to methionine metabolism during cell growth in NY9-10
To precisely determine the SAM content during cell growth, we determined the SAM content of K9 and NY9-10 at 30°C in YPD medium. As shown in (a), in K9 and NY9-10 strains, the maximum SAM content occurred during the exponential phase (6 h at 30°C), and then dramatically decreased. Furthermore, the SAM content was markedly higher in NY9-10 than in K9. In addition, various components related to methionine metabolism, such as SAH and Hcy, were determined in sake yeast K9 and the bred strain NY9-10. The mechanism through which SAH continues to increase with cultivation time is unknown. However, the SAH and Hcy contents in NY9-10 increased compared to those in K9 ((b,c)). These results suggest that SAM accumulation due to the functional deficiency of ADO1 in the NY9-10 strain could be due to various mechanisms contributing to hyperactivation of the methionine biosynthesis pathway. Next, to confirm the possibility of industrial use of NY9-10, we determined the SAM content during cell growth at 30°C in O medium. In YPD medium, the SAM content in NY9-10 was higher than that in K9 (especially at 6–12 h) and then slowly decreased. As shown in , both strains entered the stationary phase at 18–24 h and cell growth in NY9-10 was higher than that in K9. Interestingly, the SAM content was higher in NY9-10 than K9, and the SAM content increased during the stationary phase in both strains because O medium contains a large amount of methionine. Accordingly, we concluded that the NY9-10 strain is suitable for industrial production of SAM.
Figure 4. SAM content during cell growth in YPD medium in the K9 and NY9-10 strains.
Cell extracts were obtained at the indicated time points. The optical density at 660 nm (OD660, opened, dashed lines) and the (a) SAM, (b) SAH, and (c) Hcy content (closed, solid lines) were determined. Symbols: triangles, K9; circles, NY9-10. Values are presented as mean ± standard deviation for three independent experiments.
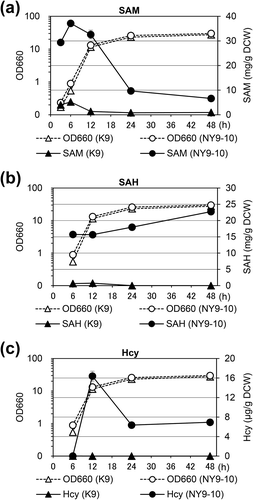
Figure 5. SAM content during cell growth in O medium in the K9 and NY9-10 strains.
Cell extracts were obtained at the indicated time points. The optical density at 660 nm (OD660, opened, dashed lines) and the SAM content (closed, solid lines) were determined. Symbols: triangles, K9; circles, NY9-10. Values are presented as mean ± standard deviation for three independent experiments.
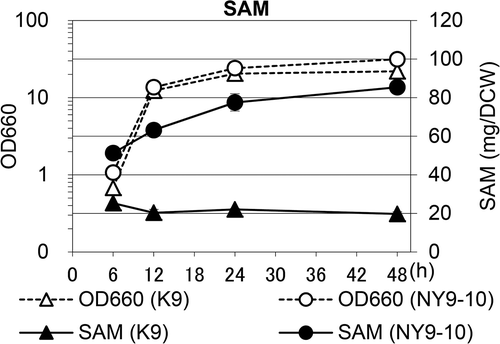
Small-scale sake making test
Finally, to confirm whether NY9-10 is suitable for sake making, we performed a small-scale sake making test (400 g total rice) using the K9 and NY9-10 strains. After 19 days of fermentation culture, 160 mL of mash was centrifuged and separated into solid and liquid parts. The supernatant (liquid part) was analyzed as sake, and we analyzed total CO2 output (g), nihonshu-do, ethanol concentration (%), acidity, amino acidity, and MB dyeing rate (%). The above results and the SAM content in the solid and liquid parts of the fermentation mash (moromi) (μg/g), ratio of solid part to moromi (%), and total SAM content in moromi (μg/g) are summarized in . The NY9-10 strain was fermented to an extent that is suitable for ordinary sake making, although the concentration of produced ethanol was slightly low. Moreover, the total SAM content of moromi was slightly higher in NY9-10 than in K9. Because methionine is supplied from rice for sake making, we predicted that the difference in SAM was small compared with the result obtained in YPD medium. Further studies are required in order to reveal the mechanism of SAM accumulation in the making of sake. These results indicate that the NY9-10 strain is also suitable for sake making, as it contains the highly accumulated nutrient component SAM. The correlation between alcoholic fermentation and SAM accumulation remains unclear in this study, thus warranting further study. In NY9-10, because the AMP level essential for the activation of phosphofructokinase (EC 2.7.1.11) in glycolysis pathway is thought to be low due to functional deficiency of the ADO1 gene, we speculate that the reason for slightly reduced alcoholic fermentation in NY9-10 compared to K9 is the reduced AMP level.
Table 1. Sake making characteristics and the SAM content of moromi in the K9 and NY9-10 strains.
Conclusions
In the present study, by selecting cordycepin-resistant sake yeast strains, we successfully bred yeast strains that highly accumulated SAM. Because the resulting strains are not genetically-modified organisms, they can be employed for industrial purposes. Thus, we proposed and tested the use of cordycepin-resistant strains in sake making. In such cases, the brewing by-products (e.g., sake cake) contain high levels of SAM, which may add value to the brewing by-products.
Author contributions
H. I and M. K. designed the study. N. Y. performed almost all the experiments. T. M., S. Y., and N. N. supported the experiments. M. K. wrote the draft of the manuscript and revised the final manuscript. M. M., T. F., and H. I. discussed the results and contributed to the improvement of the manuscript. All authors have approved the final manuscript.
Acknowledgments
We thank all the members of the Applications Research Division of the National Research Institute of Brewing for their constant guidance, support, and helpful discussions.
Disclosure statement
No potential conflict of interest was reported by the authors.
References
- Shiozaki S, Shimizu S, Yamada H. Unusual intracellular accumulation of S-adenosyl-l-methionine by microorganisms. Agric Biol Chem. 1984;48:2293–2300.
- Kanai M, Kawata T, Yoshida Y, et al. Sake yeast YHR032W/ERC1 haplotype contributes to high S-adenosylmethionine accumulation in sake yeast strains. J Biosci Bioeng. 2017;123:8–14.
- Ogawa T, Tsubakiyama R, Kanai M, et al. Stimulating S-adenosyl-l-methionine synthesis extends lifespan via activation of AMPK. Proc Natl Acad Sci USA. 2016;113:11913–11918.
- Kanai M, Masuda M, Takaoka Y, et al. Adenosine kinase-deficient mutant of Saccharomyces cerevisiae accumulates S-adenosylmethionine because of an enhanced methionine biosynthesis pathway. Appl Microbiol Biotechnol. 2013;97:1183–1190.
- Iwashima A, Ogata M, Nosaka K, et al. Adenosine kinase-deficient mutant of Saccharomyces cerevisiae. FEMS Microbiol Lett. 1995;127:23–28.
- Lecoq K, Belloc I, Desgranges C, et al. Role of adenosine kinase in Saccharomyces cerevisiae: identification of the ADO1 gene and study of the mutant phenotypes. Yeast. 2001;18:335–342.
- Nanba K, Obata T, Kayashima S, et al. The setting of the small sake brewing. J Soc Brew Jpn. 1978;73:295e300, (in Japanese).
- Katou T, Kitagaki H, Akao T, et al. Brewing characteristics of haploid strains isolated from sake yeast Kyokai No. 7. Yeast. 2008;25:799–807.
- Singleton CK. Identification and characterization of the thiamine transporter gene of Saccharomyces cerevisiae. Gene. 1997;199:111–121.