ABSTRACT
In order to increase secondary metabolite production in filamentous fungi, a transcription factor gene in the biosynthetic gene cluster and global regulator genes such as laeA are considered plausible as targets for overexpression by genetic modification. In this study, we examined these overexpression effect in fungal sp. No. 14919 that produces FR901512, an HMG-CoA reductase inhibitor. Resultantly, the productivity was improved at 1.7–1.8 fold by overexpressing frlE, a transcription factor gene in the biosynthetic gene cluster, whereas productivity did not change by overexpression of laeA and veA. Furthermore, we searched for extra transcription factors affecting the productivity by transcriptome analysis between wild-type strain and highly productive UV mutants. After verifying productivity decrease by overexpression, Drf1, a novel transcription factor encoded by drf1 was identified as the negative regulator. Because each frlE product (FrlE) and Drf1 worked on the same cluster in positive and negative regulatory manners, their network was analyzed.
Graphical Abstract
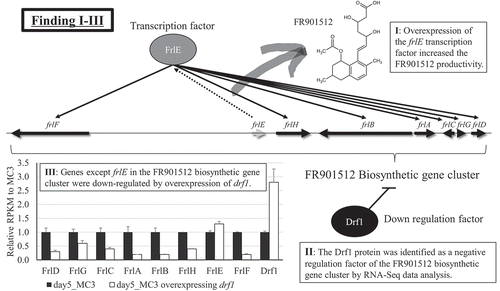
By focusing on transcription factors in fungal sp. No. 14919 produces FR901512, we identified Drf1, negative regulation factor of the FR901512 biosynthetic gene cluster.
Microorganisms produce various kinds of secondary metabolites, some of which, such as avermectin[Citation1], statin[Citation2], and tacrolimus (FK506)[Citation3], are utilized as pharmaceutical agents and raw materials. However, the productivities of these secondary metabolites by microorganisms are very low in general. Thus, for industrial use, it is indispensable to increase the productivities of these metabolites. The productivities have been increased mainly by random mutagenesis with ultraviolet (UV) irradiation, like in the case of FR901379[Citation4], and mutagenic chemical treatment[Citation5]. Although the mechanism of the productivity increase caused by random mutagenesis is unknown, the method is generally used. In fact, strain stocks for industrial use were constructed in our company by UV mutagenesis[Citation4]. With repeated UV mutagenesis, the productivity of a useful secondary metabolite was enhanced in a stepwise fashion, however screening of the mutagenized strain at every step of mutagenesis required much labor. If key genes that affects the productivity were identified, high-productive strains could be constructed more efficiently by genetic engineering in a much shorter period.
Fungal sp. No. 14919 is a filamentous fungus that produces a 3-hydroxy-3-methylglutaryl-coenzyme A (HMG-CoA) reductase inhibitor named FR901512[Citation6]. FR901512 strongly inhibits cholesterol synthesis in the human hepatoma cell line Hep G2 as well as in rats and beagle dogs[Citation7]. The IC50 value of FR901512 for cholesterol synthesis is only 1.0 nM in Hep G2 cells[Citation6], which exceeds the reported IC50 values of 24 nM for lovastatin and 34 nM for simvastatin under the same conditions[Citation8]. FR901512 is a secondary metabolite synthesized by polyketide synthase (PKS) and has a structure similar to that of the HMG-CoA reductase inhibitor lovastatin [Citation9] and ML-236B [Citation2] which are synthesized by PKS in the Aspergillus terreus [Citation10] and Penicillium ctrinum[Citation11], respectively. Lovastatin and ML-236B possess a hexahydro naphthalene nucleus bound to 2-methylbutyrate, whereas FR901512 possesses a tetralin nucleus with acetate (Figure S1).
The genomic sequence of fungal sp. No. 14919 was determined in our previous study[Citation12]. The internal transcribed spacer sequences shared 99% identity with those of Xylaria grammica in Sordariomycetes growing on deadwood. In addition, in the previous genomic point mutation analysis of a series of FR901512 highly producing mutants obtained by screening via repeated UV mutagenesis, the SREBP protein was found as a key factor to regulate the productivity[Citation13]. By the knockout of SREBP, productivity of FR901512 was revealed to be improved.
In this study, we focused on transcription factors and searched for them that affect FR901512 productivity of fungal sp. No. 14919. As the most plausible one, a transcription factor in the biosynthetic gene cluster was considered[Citation14]. Besides, there are several transcription factors known as the global regulators of secondary metabolite biosynthesis genes in filamentous fungi [Citation15,Citation16]. The transcription factors LaeA and VeA are widely known as them. We examined their involvement in FR901512 productivity by the individual overexpression. Furthermore, based on the RNA-Seq data analysis of a series of the highly FR901512 productive UV mutant strains obtained so far, the other transcription factors affecting the productivity were searched. As a result, a novel transcription factor was found as a negative regulator of the biosynthetic gene cluster.
Materials and methods
Fungal strains
The wild-type fungal sp. No. 14,919 strain MC3 was deposited in the American Type Culture Collection (ATCC) as FERM BP-3752. The genome sequence was registered at the DNA Data Bank of Japan (DDBJ) as BDMC01000001-BDMC01000104 [Citation12] and was used for reference of RNA-Seq data analysis and primer design in this study. MC3 and the two derivative mutant strains (Nos. 1019 and 1407) superimposed the UV mutagenesis from MC3 were used for preparation of gene overexpression strains. Also, MC3 and the four derivative UV-mutagenized strains (Nos. 425, 1019, 1225, and 1407) were used for preparation of RNA for RNA-Seq.
Plasmid construction, transformation, and productivity evaluation
To determine homologue of LaeA and VeA in fungal sp. No. 14919 genome, LaeA (ADL63139.1) and VeA (ADX36108.1) amino acid sequence of P. citrinum were used for BlastP search. The plasmid for overexpressing frlE (ANO14919_110,000), laeA (ANO14919_121910), veA (ANO14919_116240) and drf1 (ANO14919_086630) were constructed. These plasmids derived from pUC19 and cloned the coding sequence (CDS) region and 300 bp downstream region, together with the predicted promoter region of the domestic glyceraldehyde-3-phosphate dehydrogenase (GAPDH, ANO14919_ 044360)and the bleomycin resistance gene (bleR) (Figure S2). All plasmids were constructed using the In-Fusion® HD Cloning Kit (Clontech, CA, USA). PCR was performed using KOD-Plus-Neo DNA polymerase (Toyobo, Osaka, Japan). The constructed plasmids were sequenced by using ABI3730 capillary sequencer (Applied Biosystems, MA, USA). Protoplast preparation, transformation, FR901512 productivity evaluation by liquid culture and LC-MS analysis were performed as described previously[Citation13]. After transformation, insertion of a DNA construct to the chromosome of a transformant was confirmed by direct PCR as suspending mycelia in PCR reaction solution (Figure S2). The primer sets used for plasmid construction, sequencing, and direct PCR are listed in Table S1. FR901512 productivity was evaluated on day 5 sample.
Quantitative reverse transcription PCR analysis
RNA was isolated from the cultures on day 3 or day 5. Purified RNA (200 ng) was reverse-transcribed using a high-capacity RNA-to-cDNA kit (Applied Biosystems). Quantitative reverse transcription PCR (qRT-PCR) was performed using a StepOnePlus™ Real-Time PCR System (Applied Biosystems) and Fast SYBR® Green Master Mix (Applied Biosystems) according to the manufacturer’s instructions. The actin gene was used as an endogenous reference gene. The primer sets are listed in Table S1.
RNA-Seq analysis
Transcriptional data comparison was conducted by using our previous RNA-Seq data; registered in DDBJ as DRX083424-DRX083429 (MC3 strain), DRX083430-DRX083435 (No. 425 strain), DRX083436-DRX083441 (No. 1019 strain), DRX083454-DRX083459 (No. 1225 strain) and DRX083466-DRX083471 (No. 1407 strain). In this study, additional RNA-Seq data were taken in the same protocols in RNA isolation, RNA-Seq sample preparation, RNA-Seq by MiSeq (Illumina, CA, USA), and RNA-seq data treatment as described previously[Citation13]. RNA was isolated from the cultures on day 3 or day 5. The RNA-Seq data taken in this study were registered in DDBJ as DRX140762-DRX140767 (MC3 overexpressing frlE), DRX140768-DRX140773 (No. 1019 strain overexpressing frlE), DRX140774-DRX140779 (No. 1407 strain overexpressing frlE), and DRX140780-DRX140785 (MC3 overexpressing drf1).
Results
Overexpression effect of transcription factor genes frlE, laeA, and veA on FR901512 productivity
The transcription factor FrlE (ANO14919-110000) present in the putative biosynthetic gene cluster of FR901512 has the GAL4-like Zn2Cys6 binuclear cluster DNA-binding domain, as with LovE in the A. terreus lovastatin biosynthetic gene cluster and MlcR in P. citrinum ML-236B biosynthetic gene cluster[Citation13]. They have 27% and 29% homology to FrlE, respectively (Figure S3). As a result of overexpressing frlE under the endogenous GAPDH promoter, FR901512 productivity per cell was increased at 1.7 and 1.8 fold from wild-type MC3 strain and UV mutant No. 1019 strain, respectively (). On the other hand, productivity improvement effect was not obtained in No. 1407 strain that productivity has already increased at 9.5 fold from MC3 and at 3.1 fold from No. 1019. In addition, productivity was not improved by overexpressing laeA and veA in MC3, No. 1019, and No. 1407 strains. Expression levels of overexpressed genes were confirmed by qRT-PCR for these recombinant strains (, S4). Among all overexpression strains, only frlE overexpressing No. 1407 strain did not show increased effect in its expression level by the overexpression ()). The other gene overexpression predominantly improved its expression level relative to the control, and thus it was confirmed that expression was overexpressed as intended (). In addition, when expression of laeA and veA were overexpressed in MC3 and No. 1019 strain, the expression of frlE and frlB that encodes PKS tended to be affected ().
Figure 1. Productivity of FR901512 with overexpressing genes of transcription factor, FrlE, and global regulators, LaeA and VeA.
Productivity is shown as the amount of FR901512 produced per dry cell weight of each overexpression mutant relative to the MC3 strain. Three replicate experiments were performed, and the values of the means and standard deviations are presented (**, p < 0.01 [Student’s t test]).
![Figure 1. Productivity of FR901512 with overexpressing genes of transcription factor, FrlE, and global regulators, LaeA and VeA.Productivity is shown as the amount of FR901512 produced per dry cell weight of each overexpression mutant relative to the MC3 strain. Three replicate experiments were performed, and the values of the means and standard deviations are presented (**, p < 0.01 [Student’s t test]).](/cms/asset/2ae24c7e-6772-49f6-8c58-e1618d3855bb/tbbb_a_1584519_f0001_b.gif)
Figure 2. Results of qRT-PCR analysis. Relative expression levels of genes of (a) frlE, (b) laeA, (c) veA, and (d) polyketide synthase gene frlB on day 5 are shown.
Three strains (MC3, Nos. 1019 and 1407) described as “Orig.” were used as hosts to overexpress the genes encoding FrlE, LaeA, and VeA. In respective strains, expression level of each gene was compensated by that of actin gene, and then relative expression level was calculated. Three replicate experiments were performed, and the values of the means and standard deviations are presented. As another version of this in different description, the relative expression levels of respective genes in each mutant to “Orig.” of the MC3 strain are shown in Figure S4.
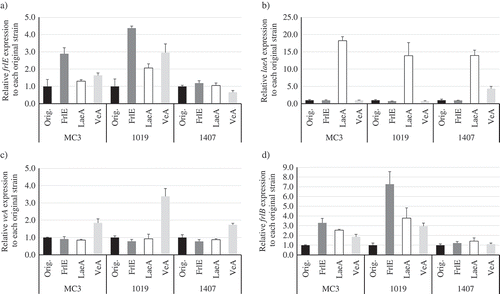
Search for extra transcription factors affecting the FR901512 productivity
Then, the expression levels of frlE were compared between MC3, Nos. 425, 1019, 1225 and 1407 strains. The Nos. 425, 1019, 1225 and 1407 strains were obtained by repeated UV mutagenesis of MC3 and had increased FR901512 productivity from MC3 at 2.5, 4.5, 9.5 and 15.2 fold, respectively[Citation13]. The expression was approximately doubled in only No. 1407 strain ()), and similar trends were found in FR901512 biosynthesis genes presumed to be under frlE control. Representatively, the expression amount of frlB was shown in ). Focusing on this phenomenon, we examined why the expression of frlE was improved in No. 1407 strain. First, among genes of fungal sp. No. 14919, the genes having transcription factor motifs were extracted. Subsequently, correlation analysis with frlE expression level was performed based on reads per kilobase of exon per million mapped fragments (RPKM) of day 3 and day 5 of MC3, Nos. 425, 1019, 1225 and No. 1407 strains (). Among the genes that had correlation to frlE in an expression pattern, validation was carried out for the top correlation ANO14919_084720 and the second ANO14919_086630 with a negative correlation by constructing recombinant strains. As a result, even when the ANO14919-084720 was overexpressed in MC3 strain, there was no change in productivity (data not shown). When ANO14919-086630 was overexpressed, the productivity of FR901512 remarkably decreased to 10% of the native MC3 strain without the overexpression ().
Table 1. Transcription factors correlated with the expression level of frlE (top five genes).
Figure 3. Results of RNA-Seq data analysis. RPKMs of a) frlE and b) frlB in the wild-type MC3 and the derivative four UV mutant (Nos. 425, 1019, 1225 and 1407) strains are shown.
Three replicate experiments were performed, and the values of the means and standard deviations are presented.
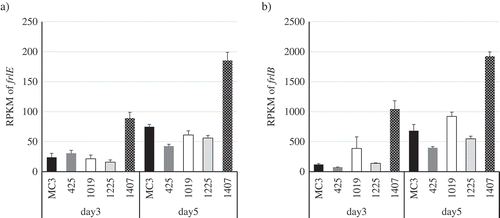
Figure 4. Productivity of FR901512 with overexpressing drf1 gene encoding Drf1.
The wild-type strain MC3 described as “Orig.” was used as a host for overexpression. Three replicate experiments were performed, and the values of the means and standard deviations are presented (**, p < 0.01 [Student’s t test]).
![Figure 4. Productivity of FR901512 with overexpressing drf1 gene encoding Drf1.The wild-type strain MC3 described as “Orig.” was used as a host for overexpression. Three replicate experiments were performed, and the values of the means and standard deviations are presented (**, p < 0.01 [Student’s t test]).](/cms/asset/291f4707-1fcb-4459-8ab3-5fcbe61579d3/tbbb_a_1584519_f0004_b.gif)
Since ANO14919-086630 negatively regulates the genes related to FR901512 biosynthesis and decreases the productivity, it was named drf1 (down regulation factor 1 of FR901512 biosynthetic genes). As a result of homology search on drf1 by Blastp, homologous genes were found in Aspergillus oryzae and A. terreus (Table S2, Figure S5). As for filamentous fungi that produce HMG-CoA reductase inhibitors with similar structures, the homologue gene ATEG-10025 (XP-001209327.1) was found in A. terreus producing lovastatin, whereas no homologue gene was found in P. citrinum producing ML-236B. RNA-Seq analysis was carried out on strains overexpressing frlE in MC3, No. 1019 and No. 1407 strains, and strains overexpressing drf1 in MC3 strain (). When drf1 was overexpressed, the expression level of frlB was reduced to 18.5% and other biosynthetic genes also had decreased expression ()). On the other hand, there was no significant change in frlE expression, but rather increased ()). To investigate the influence of drf1 overexpression to the expression of other genes, genes were extracted under the following conditions; 1) The gene that expression level decreased to less than 0.8 fold due to overexpression of drf1 and 2) the expression level was improved 1.2 fold or more between MC3 and No. 1407 strains, and between No. 1225 and No. 1407 strains. Since the expression level of drf1 was significantly decreased only in No. 1407 strain, the condition 2) denoted above was applied for refinement of extracted genes for more certainty. Both conditions corresponded to 63 genes including FR901512 biosynthesis gene (Table S3). The genes that were affected by drf1 seemed to have definitely been extracted by the conditions, however there was no bias in their annotation, and thus functional classification of them could not be done. Further, it was supposed that drf1 may negatively control not only the FR901512 biosynthesis genes but also other secondary metabolism-related genes. Therefore, the secondary metabolite biosynthesis genes (PKS, NRPS, terpene etc.) in the genome of fungal sp. No. 14919 were searched by antiSMASH Fungal version (https://fungismash.secondarymetabolites.org/#!/start). Then, the selected secondary metabolite biosynthesis genes (Table S4) were collated to the list of extracted genes presumed to be under drf1 control (Table S3). However, except for ANO14919_109980 encoding FR901512 biosynthetic PKS, they were not only included in the list but their expression was also fluctuated independently of drf1 expression (Table S4). In addition, the effect of drf1 overexpression was also confirmed preliminarily at the metabolite level using LC-MS, however no definite shift of secondary metabolites in variety and amount except FR901512 could be found (data not shown), which was identical to the results of transcriptional analysis aforementioned.
Figure 5. Results of RNA-Seq data analysis. RPKMs of (a) frlE, (b) frlB, and (c) drf1 are shown. Three strains (MC3, Nos. 1019 and 1407) described as “Orig.” were used as hosts to overexpress the genes encoding FrlE, LaeA, and VeA. (d) Effect of drf1 overexpression on the expression of genes in FR901512 biosynthetic gene cluster are shown. The genes shown encode FrlD, Transesterase; FrlG, Thioesterase; FrlC, Enoyl reductase; FrlA, P450 monooxygenase; FrlB, Polyketide synthase; FrlH, HMG-CoA reductase; FrlE, Transcription factor; and FrlF, Polyketide synthase. Three replicate experiments were performed, and the values of the means and standard deviations are presented.
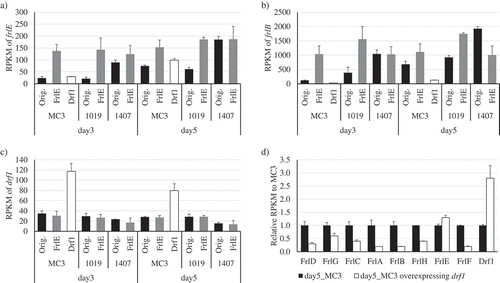
Discussion
In this study, we focused on transcription factors that affect productivity of FR901512, and there were three findings. Firstly, overexpression of the frlE transcription factor increased the FR901512 productivity. Secondly, the Drf1 protein was identified as a negative regulation factor of the FR901512 biosynthetic gene cluster by RNA-Seq data analysis. Thirdly, the genes except frlE in the FR901512 biosynthetic gene cluster was down-regulated by overexpression of drf1.
In our previous study, we also verified that sequences of the genes focused in this study (FR901512 biosynthetic genes containing frlE, laeA (ANO14919_121910), veA (ANO14919_116240) and drf1 (ANO14919_086630)) were correct. No mutation was generated in these genes including the 1,000 bp-long upstream and downstream regions throughout UV mutagenesis from MC3 strain to No. 1407 strain[Citation13]. Thus, the FR901512 productivity increase observed in this study is proven not to be due to their DNA sequence shift.
As examples of overexpression of transcription factors in the secondary metabolic biosynthetic gene clusters in filamentous fungi, several reports regarding aflatoxin production in Aspergillus flavus [Citation17] and monacolin K production in Monascus pilosus [Citation18] are there. Similarly, in this fungus, the productivity of FR901512 was improved by the overexpression of the transcription factor gene frlE that was located in the biosynthetic gene cluster. As for the overexpression of frlE in No. 1407 strain, although insertion of the DNA construct for overexpression was confirmed (Figure S2), expression level of frlE gene itself did not increase ()), and thus productivity did not change (). From the results of the RNA-Seq of MC3 and all UV mutant strains, the expression level of the biosynthesis genes including frlE is about 2 fold higher in only No. 1407 strain than the other strains (). The expression level seemed to have already reached the threshold saturation value, and therefore overexpression of frlE in No. 1407 strain could not be attained.
Differently from frlE, productivity of FR901512 was not improved by overexpression of either laeA or veA homologue gene. It has been reported that LaeA epigenetically controls genes at the chromatin level in secondary metabolite production of Aspergillus sp[Citation15]. By overexpression of laeA, a transcription factor lovE in the lovastatin biosynthetic gene cluster is highly expressed in A. terreus ATCC 20542 strain, and the biosynthesis genes are also induced[Citation15]. It is known that LaeA forms a complex with VeA and VelB in the nucleus and exerts its function[Citation16]. Although LaeA is considered to be a methylation enzyme (demethylation, euchromatinization) due to its SAM binding domain, details of molecular mechanism of LaeA have not been elucidated. Also in P. citrinum, it is reported that overexpression of laeA improves the productivity of ML-236B[Citation19]. Furthermore, in M. pilosus which produces monacolin K, there is a report that secondary expression of metabolites including monacolin K increased when laeA was overexpressed[Citation20]. However, in this study, fungal sp. No. 14919 showed no improvement in the FR901512 productivity by the laeA overexpression. In the previous study, LaeA2 of P. citrinum, a splicing variant of LaeA1 generated by a spontaneous mutation, that has additional 15 residues of C-terminal only showed effect on improving the ML-236B productivity[Citation21]. Thus, we compared amino acid sequences of the LaeA homologue of fungal sp. No. 14919 with LaeA-α, -β of A. terreus [Citation22] and LaeA1, A2 of P. citrinum. Resultantly, the LaeA homologue in fungal sp. No. 14919 retained the 15 residues-added C-terminal corresponding to LaeA2, as well as the SAM binding domain (Figure S6)[Citation15]. Therefore, the LaeA homologue was suggested to have the same effect on improving the productivity of secondary metabolite FR901512, however it did not. Since expression of frlE and frlB increased up to 3 fold at maximum when laeA or veA were overexpressed in MC3 and No. 1019 strains (), it remains unclear why no improvement in productivity of FR901512 was observed by their overexpression. Analysis of the actions of these global regulators in this fungus, such as confirming whether the productivity of FR901512 disappears when deleted, may lead to further understanding of secondary metabolic production.
We have been conducting productivity improvement verification of FR901512 by overexpression of global regulator based on known reports, but in addition to these, key genes that increase productivity of FR901512 were searched. Although the expression level of drf1 did not change significantly in MC3, Nos. 425, 1019 and 1225 strains, it is reduced by 36 to 45% compared with other strains in No. 1407 strain, and a negative correlation with frlE expression level was seen (Figure S7). Overexpression of drf1 suppressed the expression of the biosynthetic genes of FR901512 (), and hence it was expected that the biosynthetic genes will be more highly expressed if drf1 is deleted. In fungal sp. No. 14919, site-specific deletion strain could be constructed by using CRISPR-Cas9 system [Citation13,Citation23], and thus we tried to make it but failed to obtain a deletion strain of drf1. If drf1 is an essential gene for the fungus, it would be impossible to acquire the deletion strain due to lethal effect. Interestingly, although this factor was found in correlation with frlE, frlE was not down-regulated when drf1 was overexpressed, while seven genes of frlA, frlB, frlC, frlD, frlG, frlH and frlF under the control of FrlE were significantly negatively regulated ()). It is desirable to analyze the unique control mechanism of Drf1 in detail such by analyzing whether the binding domain of FrlE competes with DNA recognition sequence of Drf1. In addition, it is very intriguing to verify the effect if drf1 is overexpressed in No. 1407 strain where expression level of drf1 decreased and frlE expression level increased.
As a transcription factor having high homology with Drf1 found in this paper, ARD1 of Candida albicans SC5314 can be mentioned. It is involved in signaling of acetic acid-induced programmed cell death[Citation24]. On the other hand, in filamentous fungi, it was not possible to find an example of analyzing Drf1 homologues, including A. oryzae in which homologues existed. In this study, we looked at the effect by overexpression of drf1. However, it is desirable that the effect by lower expression is also looked by replacing the original promoter with weaker one hereafter, which would lead to more detailed characterization of Drf1 function. Also, it is considered very interesting to look at the effect of drf1 on A. terreus by heterologous expression. If further examination progresses, such as whether there are other PKS responding to Drf1, it seems that network understanding for secondary metabolic production will advance more than ever.
In this study, it was confirmed that the productivity of FR901512 was improved depending on the expression level of transcription factor in its biosynthetic gene cluster. In addition, the global regulators LaeA and VeA, which have been proven to improve the productivity of secondary metabolites in other filamentous fungi, did not contribute to FR901512 productivity in fungal sp. No. 14919. Furthermore, by RNA-Seq data analysis of UV mutants, we found a novel transcription factor Drf1 that negatively regulates the FR901512 biosynthetic genes. Finding transcription factors regulating expression of secondary metabolism biosynthetic genes in such a way will provide knowledge useful for efficient improvement of productivity of other secondary metabolites in microorganisms by genetic engineering.
Author contributions
Itoh and Miura performed the experiments. Takeda performed computational analysis. Itoh and Tamano wrote the manuscript. Matsui, Machida and Shibata thoroughly discussed and supervised the project.
Supplementary_revision.pdf
Download PDF (977.1 KB)Disclosure statement
No potential conflict of interest was reported by the authors.
Supplementary material
Supplemental data for the article can be accessed here.
Additional information
Funding
References
- Burg RW, Miller BM, Baker EE, et al. Avermectins, new family of potent anthelmintic agents: producing organism and fermentation. Antimicrob Agents Chemother. 1979;15:361–367.
- Endo A. Compactin (ML-236B) and related compounds as potential cholesterol-lowering agents that inhibit HMG-CoA reductase. J Med Chem. 1985;28:401–405.
- Goto T, Kino T, Hatanaka H, et al. Discovery of FK-506, a novel immunosuppressant isolated from Streptomyces tsukubaensis. Transplant Proc. 1987;19:4–8.
- Kanda M, Tsuboi M, Sakamoto K, et al. Improvement of FR901379 production by mutant selection and medium optimization. J Biosci Bioeng. 2009;107:530–534.
- Iwakuma H, Koyama Y, Miyachi A, et al. Generation of a glucose de-repressed mutant of Trichoderma reesei using disparity mutagenesis. Biosci Biotechnol Biochem. 2016;80:486–492.
- Hatori H, Sato B, Sato I, et al. FR901512, a novel HMG-CoA reductase inhibitor produced by an agonomycetous fungus No. 14919. I. Taxonomy of the producing organism, fermentation, isolation and physico-chemical properties. J Antibiot. 2004;57:264–270.
- Hatori H, Sato B, Sato I, et al. FR901512, a novel HMG-CoA reductase inhibitor produced by an agonomycetous fungus No. 14919. II. Biological profiles. J Antibiot. 2004;57:390–393.
- Cohen LH, van Vliet A, Roodenburg L, et al. Pravastatin inhibited the cholesterol synthesis in human hepatoma cell line Hep G2 less than simvastatin and lovastatin, which is reflected in the upregulation of 3-hydroxy-3-methylglutaryl coenzyme A reductase and squalene synthase. Biochem Pharmacol. 1993;45:2203–2208.
- Alberts AW, Chen J, Kuron G, et al. Mevinolin: a highly potent competitive inhibitor of hydroxymethylglutaryl-coenzyme A reductase and a cholesterol-lowering agent. Proc Natl Acad Sci U S A. 1980;77:3957–3961.
- Hendrickson L, Davis CR, Roach C, et al. Lovastatin biosynthesis in Aspergillus terreus: characterization of blocked mutants, enzyme activities and a multifunctional polyketide synthase gene. Chem Biol. 1999;6:429–439.
- Abe Y, Suzuki T, Ono C, et al. Molecular cloning and characterization of an ML-236B (compactin) biosynthetic gene cluster in Penicillium citrinum. Mol Genet Genomics. 2002;267(5):636–646.
- Itoh H, Matsui M, Kumagai T, et al. Genome sequence of the fungal strain 14919 producing 3-hydroxy-3-methylglutaryl-coenzyme A reductase inhibitor FR901512. Genome Announc. 2017;5:e00129–17.
- Itoh H, Miura A, Matsui M, et al. Knockout of the SREBP system increases production of the polyketide FR901512 in filamentous fungal sp. No. 14919 and lovastatin in Aspergillus terreus ATCC20542. Appl Microbiol Biotechnol. 2018;102(3):1393–1405.
- Matsui M, Yokoyama T, Nemoto K, et al. Identification of a putative FR901469 biosynthesis gene cluster in fungal sp. No. 11243 and enhancement of the productivity by overexpressing the transcription factor gene frbF. J Biosci Bioeng. 2017;123(2):147–153.
- Bok JW, Keller NP. LaeA, a regulator of secondary metabolism in Aspergillus spp. Eukaryot Cell. 2004;3(2):527–535.
- Bayram O, Krappmann S, Ni M, et al. VelB/VeA/LaeA complex coordinates light signal with fungal development and secondary metabolism. Science. 2008;320(5882):1504–1506.
- Flaherty JE, Payne GA. Overexpression of aflR leads to upregulation of pathway gene transcription and increased aflatoxin production in Aspergillus flavus. Appl Environ Microbiol. 1997;63(10):3995–4000.
- Chen YP, Yuan GF, Hsieh SY, et al. Identification of the mokH gene encoding transcription factor for the upregulation of monacolin K biosynthesis in Monascus pilosus. J Agric Food Chem. 2010;58(1):287–293.
- Zheng Y, Cao S, Huang Y, et al. Overexpression of LaeA enhances mevastatin production and reduces sporulation of Penicillium citrinum. Wei Sheng Wu Xue Bao. 2014;54(12):1438–1445.
- Lee SS, Lee JH, Lee I. Strain improvement by overexpression of the laeA gene in Monascus pilosus for the production of monascus-fermented rice. Microbiol Biotechnol. 2013;23(7):959–965.
- Baba S, Kinoshita H, Nihira T. Identification and characterization of Penicillium citrinum VeA and LaeA as global regulators for ML-236B production. Curr Genet. 2012;58(1):1–11.
- Palonen EK, Raina S, Brandt A, et al. Transcriptomic complexity of Aspergillus terreus velvet gene family under the influence of Butyrolactone I. Microorganisms. 2017;5(1):pii: E12.
- Arazoe T, Miyoshi K, Yamato T, et al. Tailor-made CRISPR/Cas system for highly efficient targeted gene replacement in the rice blast fungus. Biotechnol Bioeng. 2015;112:2543–2549.
- Laera L, Guaragnella N, Ždralević M, et al. The transcription factors ADR1 or CAT8 are required for RTG pathway activation and evasion from yeast acetic acid-induced programmed cell death in raffinose. Microb Cell. 2016;3(12):621–631.