ABSTRACT
It has been reported that airborne methyl jasmonate (MeJA) was metabolized into jasmonic acid (JA) and jasmonoyl isoleucine (JA-L-Ile). In this report, jasmonoyl valine (JA-L-Val), 12-hydroxy JA (12OHJA), and 12-glucosyloxy JA (12OGlcJA) were identified as metabolites originating from airborne MeJA using tomato (Solanum lycopersicum). Furthermore, the preferable conversion of (-)-MeJA (natural form) into 12OHJA, 12OGlcJA, JA-L-Ile, and JA-L-Val was observed.
KEYWORDS:
Plants produce a wide variety of volatile substances during their life cycle. Among them, certain volatile substances play pivotal roles in response to various environmental stresses. Green leaf volatiles, which are widely produced in the plant kingdom, are able to repel or attract herbivores and their predators and have the biological potential to induce or prime the plant defense system against herbivores and pathogens. In some cases, green leaf volatiles possess direct toxic effects versus bacteria and fungi. For example, exogenous application of (3Z) hexn-3-al shows protective effects for the plant against Botrytis cinerea [Citation1]. Methyl jasmonate (MeJA) is a well-known biologically active volatile compound [Citation2]. Interestingly, Koda et al. showed that airborne MeJA was able to induce potato tuber using potato single nodes [Citation3],and Tamogami et al. [Citation4] showed that airborne MeJA was metabolized into jasmonic acid (JA) and jasmonoyl isoleucine (JA-L-Ile). However, the hydroxy or glucosyl JA-related metabolite derived from airborne MeJA has not been reported. In this paper, we report other metabolites derived from airborne MeJA, as well as the preferable conversion of (-)-MeJA (natural form), compared with (+)-MeJA (unnatural form), into 12-hydroxy jasmonic acid (12OHJA), 12-O-glucosyloxyjasmonic acid (12OGlcJA), JA-L-Ile, and JA-L-valine (JA-L-Val) using tomato (Solanum lycopersicum) as an experimental plant.
As candidates for hydroxy or glucosyl JA-related metabolites, 12OHJA and 12OGlcJA were selected because these compounds were detected in many plants [Citation5]. Furthermore, JA-L-Val was also selected because of its biological activity to bind COI1 proteins similar to JA-L-Ile [Citation6]. To investigate the difference of metabolite and metabolism of the airborne (-)- and (+)-MeJA, (-)- and (+)-JA were prepared from commercially available MeJA according to the reports of Kiyota et al. [Citation7], respectively. The obtained (-)- and (+)-JA were converted into (-)-MeJA (natural form) and (+)-MeJA (unnatural form), respectively, using excess amount of CH2N2 in MeOH according to the usual manner, and deuteriums were introduced into the synthesized MeJAs using CH3OD and NaOCH3 according to a reported method [Citation8] to give [2H2-2, 2H2-5, 2H1-7] (-)- and (+)-MeJA (MeJA-d5).
In case of analyzing JA-L-Ile synthesized from (-)-JA prepared according to the reported method [Citation7] and L-Ile (Figure 2S, A in the Supplemental Material), two peaks were observed in ultra performance liquid chromatography tandem mass (UPLC MS/MS) chromatograph, in which the retention time of the earlier one was thought to be derived from cis form of (-)-JA-L-Ile, namely (3R, 7S) JA-L-Ile, and latter one to be trans form of (-)-JA-L-Ile, namely (3R, 7R) JA-L-Ile, which was generally accepted that trans isomer of JA was a dominant form of the compound. In case of analyzing JA-L-Ile synthesized from (±)-JA and L-Ile (Figure 2S, B in the Supplemental Material), two peaks were observed in UPLC MS/MS chromatograph, in which the retention time of the later peak had good accordance with that of trans (-)-JA-L-Ile synthesized from (-)-JA. The almost same tendency was observed in the case of analyzing JA-L-Val (Figure 3S in the Supplemental Material).
Tomato plants (S. lycopersicum cv Kyoryoku-beijyu) that grew in a growth chamber under the conditions such as 14 h (light), 10 h (dark), and 22°C for 21 days were placed in a semi-closed container with filter paper loaded (-)- or (+)-MeJA-d5 (1 mg) as shown in Figure 1S in the Supplemental Material. After 24 h, the upper three leaves of the plants were harvested and extracted. To survey the presence of the predicted deuterium-labeled compounds with the naturally occurring JA and its metabolites, UPLC MS/MS analysis was performed according to a reported method [Citation9]. Since deuterium at the C-7 position of MeJA-d5 is susceptible to epimerization by enolization of the ketone [Citation10], we analyzed not only the metabolites derived from MeJA-d5 but also those originating from [[2H2-2, 2H2-5] MeJA (MeJA-d4).
When exposed to (-)-MeJA-d5 (natural form), the plants accumulated trans (-)-JA-L-Ile-d4/d5 (natural form) and trans (-)-JA-L-Val-d4/d5 (natural form) in their leaves (). In case of (-)-JA-L-Ile, the minor peak appeared at earlier retention time than that of the major peak was determined to be derived from cis form of (-)-JA-L-Ile due to good coincidence of the retention time with that of cis (-)-JA-L-Ile in Figure 2S, A in the Supplemental Material. Furthermore, the corresponded minor peak was not observed in the sample derived from the non-treated plant (Figure 4S in the Supplemental Material). However, the minor peaks in the chromatograph analyzing (-)-JA-L-Val were thought to be derived from unidentified compounds because their retention times were not coincided with those of cis form of JA amino conjugate nor (+)-JA amino conjugate.
Figure 1. Metabolism of airborne MeJA into JA-L-Ile and L-Val. A: Monitoring standards of (±)-JA-L-Ile (left panel) and (±)-JA-L-Val (right panel). B: Monitoring (-)-JA-L-Ile (left panel) and (-)-JA-L-Val (left panel) using the plants exposed to (-)-MeJA-d5. C: Monitoring (+)-JA-L-Ile (left panel) and (+)-JA-L-Val (left panel) using the plants exposed to (+)-MeJA-d5. (a) The peak was monitored by selecting m/z 322.03 as the pseudo-molecular ion and m/z 129.68 as the transition ion. (b) The peak was monitored by selecting m/z 308.03 as the pseudo-molecular ion and m/z 115.58 as the transition ion. (c) The peak was monitored by selecting m/z 327.03 as the pseudo-molecular ion and m/z 129.68 as the transition ion. (d) The peak was monitored by selecting m/z 313.03 as the pseudo-molecular ion and m/z 115.58 as the transition ion. (e) The peak was monitored by selecting m/z 326.03 as the pseudo-molecular ion and m/z 129.68 as the transition ion. (f) The peak was monitored by selecting m/z 312.03 as the pseudo-molecular ion and m/z 115.58 as the transition ion.
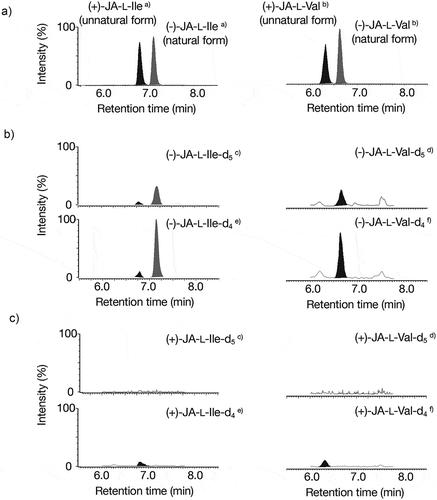
In contrast, the accumulation of (+)-JA-L-Ile-d4/d5 (unnatural form) and (+)-JA-L-Val-d4/d5 (unnatural form) was below the detection level or quite low when the plants were exposed to (+)-MeJA-d5 (unnatural form) (). Peak area ratios for (-)-JA-L-Ile-d5/(-)-JA-L-Ile and (-)-JA-L-Ile-d4/(-)-JA-L-Ile () were quite higher than those of (+)-JA-L-Ile-d5/(-)-JA-L-Ile and (+)-JA-L-Ile-d4/(-)-JA-L-Ile (). Thus, we observed that airborne (-)-MeJA was metabolized into (-)-JA-L-Ile and (-)-JA-L-Val with higher efficiency than those of airborne (+)-MeJA, although the metabolism of airborne (±)-MeJA into JA-L-Ile has been reported [Citation4]. In contrast, when focusing on the metabolism of airborne MeJA into hydroxy and glucosyl derivatives, we found that airborne (-)- and (+)-MeJA-d5 were both metabolized to 12OHJA-d4/d5, 12OGlcJA-d4/d5, and JA-d4/d5 ( and ). Since each mixture of (-)- and (+)-JA, −12OHJA, and −12OGlcJA were not able to be separable with the column used in the UPLC MS/MS analysis in this study, the (-)- and (+)-derivatives were not distinguished. Because peak area ratios of 12OHJA-d5/12OHJA and 12OHJA-d4/12OHJA derived from (-)-MeJA-d5 () were relatively higher than those of 12OHJA-d5/12OHJA and 12OHJA-d4/12OHJA derived from (+)-MeJA-d5 (), it was able to say that airborne (-)-MeJA was metabolized to 12OHJA with higher efficiency than those of airborne (+)-MeJA. Similar to this, (-)-MeJA was metabolized to 12OGlcJA with higher efficiency than those of airborne (+)-MeJA.Interestingly, it was found that accumulation levels of JA-d4/d5 derived from airborne (+)-MeJA were higher than those from airborne (-)-MeJA (). This observation implies that conversion of airborne (+)-MeJA into (+)-JA had a higher rate than (-)-MeJA or airborne (-)-MeJA to stimulate JA metabolism. To determine the real picture, further studies should be performed. In conclusion, we found that 12OHJA, 12OGlcJA, and JA-L-Val were metabolites of airborne MeJA in this study.
Table 1. Peak area ratio of exogenous/endogenous JA and JA derivatives.a
Figure 2. Metabolism of airborne MeJA into JA, 12OHJA, and 12OGlcJA. A: Monitoring JA-related compounds. B: Monitoring 12OHJA-related compounds. C: Monitoring 12OGlcJA-related compounds. (a) The peaks filled with black color had good agreement with the retention times with that of each authentic sample. (b) The peak was monitored by selecting m/z 214.00 as the pseudo-molecular ion and m/z 60.71 as the transition ion. (c) The peak was monitored by selecting m/z 213.00 as the pseudo-molecular ion and m/z 60.71 as the transition ion. (d) The peak was monitored by selecting m/z 229.97 as the pseudo-molecular ion and m/z 60.71 as the transition ion. (e) The peak was monitored by selecting m/z 228.97 as the pseudo-molecular ion and m/z 60.71 as the transition ion. (f) The peak was monitored by selecting m/z 392.03 as the pseudo-molecular ion and m/z 210.60 as the transition ion. (g) The peak was monitored by selecting m/z 391.03 as the pseudo-molecular ion and m/z 209.60 as the transition ion.
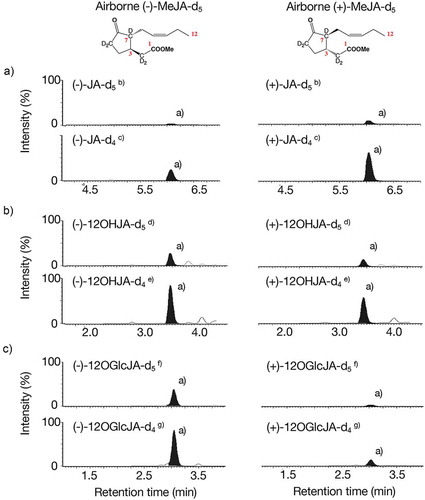
Author Contributions
KO, KM, and RM performed the experiments. KO and HM designed the study and wrote paper. KT and YT helped to draft the manuscript.
04_Supplemental_Data_03.pdf
Download PDF (397.9 KB)Acknowledgments
The MeJA used as a starting material for the synthesis of the deuterium-labeled compounds was kindly provided by the ZEON Corporation (Tokyo, Japan). We acknowledge the assistance of Dr Eri Fukushi (Research Faculty of Agriculture, Hokkaido University) in obtaining the spectroscopy data. We used the UPLC MS/MS systems at the Research Faculty of Agriculture, Hokkaido University.
Disclosure statement
No potential conflict of interest was reported by the authors.
Supplementary material
Supplemental data for this article can be accessed here.
References
- Matsui K. Green leaf volatiles: hydroperoxide lyase pathway of oxylipin metabolism. Curr Opin Plant Biol. 2006;9:274–280.
- Wasternack C. Jasmonates: an update on biosynthesis, signal transduction and action in plant stress response, growth and development. Ann Bot. 2007;100:681–697.
- Koda Y, Kikuta Y. Effects of jasmonates on in vitro tuberization in several potato cultivars that differ greatly in maturity. Plant Prod Sci. 2001;4:66–70.
- Tamogami S, Rakwal R, Agrawal GK. Interplant communication: airborne methyl jasmonate is essentially converted into JA and JA-Ile activating jasmonate signaling pathway and VOCs emission. Biochem Biophys Res Commun. 2008;376:723–727.
- Miersch O, Neumerkel J, Dippe M, et al. Hydroxylated jasmonates are commonly occurring metabolites of jasmonic acid and contribute to a partial switch-off in jasmonate signaling. New Phytol. 2008;177:114–127.
- Katsir L, Schilmiller A, Staswick PE, et al. COI1 is a critical component of a receptor for jasmonate and the bacterial virulence factor coronatine. Proc Natl Acad Sci U S A. 2008;105:7100–7105.
- Kiyota H, Higashi E, Koike T, et al. Lipase-catalyzed preparation of both enantiomers of methyl jasmonate. Tetrahedron Asymmetr. 2001;12:1035–1038.
- Galka PW, Ambrose SJ, Ross ARS, et al. Syntheses of deuterated jasmonates for mass spectrometry and metabolism studies. J Labelled Comp Radiopharm. 2005;48:797–809.
- Matsuura H, Aoi A, Satou C, et al. Simultaneous UPLC MS/MS analysis of endogenous jasmonic acid, salicylic acid, and their related compounds. Plant Growth Regul. 2009;57:293–301.
- Holbrook L, Tung P, Ward K, et al. Importance of the chiral centers of jasmonic acid in the responses of plants (activities and antagonism between natural and synthetic analogs). Plant Physiol. 1997;114:419–428.