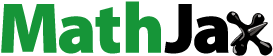
ABSTRACT
In Experiment 1, the effects of calcium maltobionate (MBCa) on calcium and magnesium absorption were examined using male rats. Four diets were designed in which 25%, 50%, and 100% of calcium carbonate (CaCO3, Control) were substituted with MBCa and were designated as MBCa-25, MBCa-50, and MBCa-100, respectively. The cecal concentration of short-chain fatty acids was significantly higher in groups MBCa-50 and MBCa-100; however, pH of cecal contents did not significantly differ among the groups. Retention rates of calcium and magnesium were significantly higher in all MBCa groups as compared to the Control. In Experiment 2, the efficiency of calcium absorption was compared using everted sacs of jejunum and ileum with CaCO3 and MBCa as calcium sources. More calcium from MBCa was absorbed as the concentration of calcium increased in comparison to CaCO3. It was concluded that MBCa is a better calcium source than CaCO3 in terms of both calcium retention and absorption.
Abbreviations: ANOVA: analysis of variance; Ca: Calcium; CaCO3: calcium carbonate; ICP-OES: Inductivity coupled plasma optical emission spectrometer; Mg: magnesium; MBCa: calcium maltobionate; OCPC: o-cresolphthalein complexone; SCFAs: short-chain fatty acids; SE: standard error; TRPM6: transient receptor potential melastatin 6.
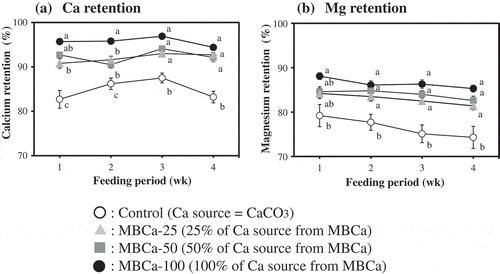
Calcium carbonate was substituted with calcium maltobionate (MBCa) at 25, 50 and 100% based on calcium equivalency. Absorption of Ca (a) and Mg (b) was significantly higher in MB-Ca diet groups as compared to the control group.
Calcium (Ca) is the fifth most abundant element in the human body, and in the order of descending abundance, carbon, hydrogen, oxygen, and nitrogen are the other four most common elements. Calcium is involved in physiologically important processes and actions such as blood clotting, neuromuscular excitation, and enzymatic activity. So, we need to take adequate Ca from diets. Geriatric-onset osteoporosis accompanying Ca deficiency has become a major public health concern as the average human lifespan increases [Citation1,Citation2], and the number of such patients in Japan is estimated to be 13 million [Citation3]. Total bone loss in the younger generations, in particular, is indicative of inadequate Ca intake in those age groups, and this issue appears to become severer as these generations age [Citation4]. Thus, maintaining proper Ca intake from a younger age is important for extending the healthspan [Citation5].
To address the above situations where Ca intake falls below the recommended level, foods can be enriched with Ca to increase Ca intake. Calcium carbonate (CaCO3) in particular is the most widely used additive in Ca-enriched foods such as snack foods, dairy beverages, bread, and noodles because it is an inexpensive source of calcium.
In general, most of the calcium present in food products is in the form of an insoluble salt and is not absorbed by the body in this form. Rather, this calcium is absorbed primarily in the small intestine after ionization by stomach acid. Calcium salts with higher solubility have been reported to have an absorption-enhancing effect [Citation6–Citation8], and calcium carbonate, which has negligible solubility in water, has poor Ca bioavailability, impeding its effectiveness as an additive for increasing Ca intake.
Maltobionic acid (4-O-α-D-Glucopyranosyl-D-gluconic acid: CAS No. 534–42-9), a molecule in which glucose is α-1,4-bonded to gluconic acid, is an indigestible disaccharide present in honey. Because this compound contains multiple hydroxyl groups and one carboxyl group, it possesses properties characteristic of both saccharides and acids. Maltobionic acid can also form a stable salt with inorganic cations, and when bound to Ca to form maltobionate calcium (MBCa), manifests high solubility in water as compared to existing Ca-containing compounds even in pH above 7.0 [Citation9].
As a result of our research to date, we have reported that the rate of Ca absorption from MBCa is significantly higher than that from CaCO3 and calcium gluconate (C12H22CaO14) according to accumulation experiments on rats [Citation9]. Since maltobionate (MB) has remained in the lumen after Ca absorption, remaining MB might have some effects on other minerals. And some indigestible carbohydrates, which increased Ca absorption, were reported to increase Mg absorption [Citation10]. So, magnesium was also set as the second target mineral in this experiment. The purpose of the present study was to assess the effect of replacing CaCO3 with MBCa in diets fed to rats on the rates of absorption of Ca and Mg because of the superior water solubility of MBCa.
Materials and methods
In vivo assays of calcium maltobionate ingestion (Experiment [Exp.] 1)
Experimental animals
Five-week-old male Wistar rats weighing 120–140 g were obtained from SLC (Shizuoka, Japan). The rats were housed individually in stainless-steel wire-bottomed cages in a room maintained at 23 ± 1°C on an inverted 12-h light–dark cycle (lights on at 06:00 AM). Prior to each feeding experiment, the rats were allowed to adapt to the AIN-76 diet for 7 d. The study protocol was approved by the Gifu University Committee for Animal Research and Welfare and for Proper Use of Laboratory Animals.
Experimental diets
The composition of the different types of diet used in our experiments is described in . One type of diet was prepared by partially altering the composition of the AIN-76 diet to contain only CaCO3 as a Ca source (the control group) and the Ca concentration was 50% of that in the AIN-76 diet. Another type of diet was prepared by replacing a portion of CaCO3 present in the control diet with an amount of MBCa equivalent to 25% of the CaCO3-based Ca content (the MBCa-25 group). In the last two types of diets, substituted amounts of MBCa were equivalent to 50% and 100% of the CaCO3-based Ca content in the control diet (the MBCa-50 group and MBCa-100 group). In addition, the Ca concentration of these diet types was designed to be insufficient for Japanese adults, and all the diets in this study contained 50% less Ca in comparison with the unaltered AIN-76 diet. MBCa was prepared according to the method proposed by Fukami et al. [Citation11]; CaCO3, sucrose, and soybean oil were purchased from Nacalai Tesque Co., Ltd. (Kyoto, Japan); choline bitartrate was bought from Wako Pure Chemical Industries Co., Ltd. (Osaka, Japan); and other ingredients were acquired from Oriental Yeast Co., Ltd. (Tokyo, Japan).
Table 1. Composition of the experimental diets (grams per 100 g of diet).
Methodology for animal experiments
Rats (n = 7) were distributed into groups such that the average body weights of all groups were equal and then raised under the designated study conditions for 28 d. Pair feeding was carried out to ensure the consistency of Ca intake across all groups, and the animals were provided ion exchange–filtered drinking water. The rats were allowed to consume water ad libitum. The rats’ body weights and amounts of diet ingested were measured daily during the experimental period. Fecal and urine samples were collected for times during the experimental period starting from day 3 after initiation of the study diet to day 5 (week 1), from day 10 to day 12 (week 2), from day 17 to day 19 (week 3), and from day 24 to day 26 (week 4). The collected fecal samples were lyophilized and then powderized using a Milser device (IFM-600 DG, Iwatani Sangyo Co., Ltd., Osaka, Japan). The collected urine samples were centrifuged at 1,500 × g and 4°C for 15 min. The processed fecal and urine samples were used for measurement of Ca and Mg concentrations, respectively.
Rats were subjected to anesthesia with pentobarbital sodium (Somnopentyl®; Kyoritsu Pharmaceutical Co., Ltd, Tokyo, Japan) at 9:30 AM 29 d after the start of the experiment. Blood samples were collected from the abdominal aorta by means of a heparinized syringe, and the rats were euthanized by cervical dislocation. The contents of the small intestine (jejunum + ileum) were removed. After measurement of the weight of the unprocessed cecum, its contents were collected, and after a rinse with physiological saline, the weight of cecum tissue alone was measured, and the total weight of cecal contents was calculated from the difference. Next, pH of jejunal, ileual, and cecal contents were measured using a compact pH meter (B-212, Horiba, Kyoto, Japan) after preparation of a fivefold dilution of a portion of the contents with deionized water immediately after collection. The remaining contents of the cecum were analyzed for short-chain fatty acids (SCFAs) and the rate of Ca solubilization. Combined small intestinal contents were also analyzed for the rate of Ca solubilization. The left-femur length was measured with calipers after removal of the periosteum and tendons from the knee joint and detachment of the femur from the pelvic hinge, and then the femur was dehydrated at 110°C for 15 h, after which dry weight was measured.
Quantification of SCFAs in the cecal contents
SCFAs in cecal contents were quantitated by the method of Rémésy and Démigne [Citation12] using Shimadzu GC-14A equipped with a flame ionization detector and a capillary column (CBP 20, 0.25 mm × 25 m; Shimadzu, Tokyo, Japan), with crotonic acid as the internal standard. Acetic acid, propionic acid, n-butyric acid, and crotonic acid were purchased from Nacalai Tesque (Kyoto, Japan).
Measurement of Ca and Mg retention and femoral Ca concentration
Powderized fecal and femur samples (0.1 g each) were weighed out into a Teflon beaker, added to 11 mL of concentrated nitric acid or 10 mL of 70% perchloric acid + 7 mL of 6 M hydrochloric acid, respectively, and then heated on a hot plate at 120°C for 390 min to achieve wet ashing. Urine samples were not subjected to the ashing treatment and were analyzed without any processing. Ca concentration in feces and urine and femoral Ca concentration were determined by the o-cresolphthalein complexone (OCPC) method [Citation13], and Mg was quantified on an ICP-OES device (Optima 7300 DV, Perkin Elmer, MA, USA). In vivo Ca and Mg retention rates were calculated from the quantities of these elements present in excreta by means of the following formulae:
Measurement of the Ca solubilization rate in intestinal and cecal contents
Samples of small intestinal and cecal contents (0.1 g each) were separately weighed out, resuspended in 0.4 mL of ion exchange–filtered water, and then homogenized (HG-30, Hitachi Koki, Tokyo, Japan). The total Ca concentrations in the intestinal and cecal contents were measured by the OCPC method after wet ashing. The rate of Ca solubilization in the supernatant obtained by ultracentrifuging the homogenate (30,000 × g for 20 min at 4°C, himac CS 120 GX II, Hitachi Koki Co., Ltd., Tokyo, Japan) was calculated in accordance with the following formulae:
Assessment of in vitro Ca absorption in the rat small intestine by the everted-sac method (Exp. 2)
The everted-sac technique applied to rats’ small intestines was performed on the basis of a partially modified version of the method proposed by Wilson et al. [Citation14] Six-week-old Wistar ST/Clean male rats (body weight: 160–180 g) were prefed with the AIN-76 standard diet and ion exchange–filtered water only for 5 d and then fasted for 24 h before euthanasia and dissection. After laparotomy under Somnopentyl® anesthesia, 5 cm contiguous sections of jejunal tissue (15 cm from the jejunostomy site) and terminal ileal tissue (15 cm from the ileocecal valve) were, respectively, excised. These intestinal tissue sections were then inverted using a thin rounded glass rod, one end of which was ligated with silk thread. The other end of the sac was ligated after injection with 0.4 mL of a serosal solution (10 mM Tris-HCl buffer pH 7.4, 125 mM NaCl, 4 mM KCl, 10 mM glucose, and 1.25 mM calcium carbonate or calcium maltobionate) and then immersion into 120 mL of prepared 200 mL of a mucosal solution (10 mM Tris-HCl buffer pH 7.4, 125 mM NaCl, 4 mM KCl, 10 mM glucose with 10, 20, or 40 mM calcium carbonate or calcium maltobionate), which had been sufficiently bubbled with a gas mixture (O2/CO2 = 95/5, v/v) beforehand and then stored at 37°C. The time point of everted-sac immersion was designated as the start of absorption, and the sacs were removed 30 min after the start of incubation. After that, the quantity of Ca in the liquid content of the sacs was measured by the OCPC method.
Statistical analysis
Data are expressed as mean ± standard error (SE). In Exp. 1, one-way analysis of variance (ANOVA) was performed with Bartlett’s test for all the four groups, followed by Tukey’s multiple-comparison test. In cases involving unequal variance, a nonparametric (Kruskal–Wallis) test was performed, followed by the Steel–Dwass multiple-comparison test. Alphanumeric characters served as the symbols indicating the presence of a statistically significant difference, and groups not marked with the same symbol were assumed to be significantly different.
In Exp. 2, Factor A was designated to be the difference in Ca sources and Factor B as Ca concentration in results of a two-way ANOVA, and tests were conducted based on this notation. Student’s t test was performed on differences between two groups at each Ca concentration, and an asterisk (*) indicates a significant difference of an MBCa group from the control group. For the multiple-comparison tests among concentrations of Ca arising from the same Ca source, analysis was carried out by the same methods and with the same symbols and notation as in Exp. 1.
The level of statistical significance in all the above tests was defined as a probability of less than 5% (p< 0.05). Microsoft Excel 2010 and BellCurve for Excel (Social Survey Research Information Co., Ltd., Tokyo, Japan) were used to conduct these analyses.
Results
The in vivo assay of calcium maltobionate ingestion (Exp. 1)
Final body weight, weight gain, food intake, and food efficiency ratio
Rats’ final body weight, weight gain, food intake, and food efficiency ratio are described in . No differences were observed among the groups in any measured parameter.
Table 2. Growth parameters,food intake and feed efficiency of rats fed the experimental diets.
Cecal weight, quantity of SCFAs in cecal content
presents the rats’ cecal tissue weight, wet weight of cecal content, and the amounts of SCFAs in cecal content. In the MBCa-100 group, significantly greater cecal tissue weight and cecal content wet weight were observed as compared to the control group. The total amount of SCFAs per cecum was significantly higher too in the MBCa-50 group and MBCa-100 group compared to the control group, and the amounts of SCFAs acetate and propionate per cecum were also significantly higher in the MBCa-50 group and MBCa-100 group.
Table 3. Weight of cecal tissue and cecal contents and amount of short-chain fatty acids in the cecal content of rats fed the experimental diets.
Changes in in vivo Ca and Mg retention rates during the study period
Changes in Ca and Mg retention rates during the experimental periods are depicted in , b), respectively. Significant increases in Ca retention were observed throughout the entire period in MBCa-25, MBCa-50 and MBCa-100 groups compared with the control group. In comparison with the control group, Mg retention rate was significantly higher in MBCa-50 and MBCa-100 groups in week 1, and in MBCa-25, MBCa-50, and MBCa-100 groups after week 2. In addition, the differences in these in vivo retention rates became more pronounced as the proportion of MBCa in the diet increased.
Figure 1. Calcium (a) and magnesium (b) retention rates of rats fed the experimental diets.
Rats were fed the experimental diets (listed in ) for 21 d. Feces were collected on days 3–5 (week 1), days 10–12 (week 2), days 17–19 (week 3), and days 24–26 (week 4) for evaluation of the calcium and magnesium retention rates.○ Control, ▲MBCa-25, ■ MBCa-50, ● MBCa-100Calcium retention rate (%) = [(total calcium intake – calcium excretion in feces and urine)/total calcium intake] × 100Magnesium retention rate (%) = [(total magnesium intake – magnesium excretion in feces and urine)/total magnesium intake] × 100Values are means ± SE (n = 7). For details on the experimental groups, see the legend of .Means in the same week not sharing the same superscript letter are significantly different (p< 0.05).
![Figure 1. Calcium (a) and magnesium (b) retention rates of rats fed the experimental diets.Rats were fed the experimental diets (listed in Table 1) for 21 d. Feces were collected on days 3–5 (week 1), days 10–12 (week 2), days 17–19 (week 3), and days 24–26 (week 4) for evaluation of the calcium and magnesium retention rates.○ Control, ▲MBCa-25, ■ MBCa-50, ● MBCa-100Calcium retention rate (%) = [(total calcium intake – calcium excretion in feces and urine)/total calcium intake] × 100Magnesium retention rate (%) = [(total magnesium intake – magnesium excretion in feces and urine)/total magnesium intake] × 100Values are means ± SE (n = 7). For details on the experimental groups, see the legend of Table 1.Means in the same week not sharing the same superscript letter are significantly different (p< 0.05).](/cms/asset/a5e45b31-db47-4646-814f-d6b7417890ea/tbbb_a_1611411_f0001_b.gif)
Proportions of solubilized Ca in small intestinal and cecal contents and pH in intestinal and cecal contents
shows the proportions of solubilized Ca in small intestinal and cecal contents as well as pH in jejunal, ilial and cecal contents. The Ca solubilization rate in the MBCa-100 group was significantly higher in the contents of both the small intestine and cecum as compared to the control group. Although the difference between the MBCa-25 and MBCa-50 groups was not significant, this proportion was significantly higher than that in the control group. Meanwhile, there was no significant difference in intestinal pH among the groups.
Table 4. Ratios of soluble calcium and pH in the small intestinal and cecal contents of rats fed the experimental diets.
Bone weight, bone length, and Ca concentration in the bone tissue of the left femur
Bone weight, bone length, and Ca concentration of the left femur for each rat group are listed in . Although no differences in bone weight and bone length were observed, Ca concentration in femoral bone tissue was significantly higher in MBCa-50 and MBCa-100 groups compared to the control group.
Table 5. Weight, length, and calcium content of Ca of the left femur of rats fed the experimental diet.
Assessment of in vitro Ca absorption in the rat small intestine by the everted-sac method: (Exp. 2)
, b) present the respective amounts of Ca incorporated into the everted sacs made of sections of the jejunum and ileum. Ca absorption in both the jejunum and ileum was significantly higher in the MBCa groups compared to the control group (CaCO3 only). In addition, in the MBCa groups, significantly greater jejunal Ca absorption was observed (exceeding 10 and 20 mM at 40 mM Ca concentration in the medium) relative to the control, and Ca absorption in the ileum was significantly greater in the MBCa groups too, exceeding 10 mM at 20 and 40 mM Ca concentrations in the medium. Furthermore, at the same Ca concentration, significantly greater Ca absorption was confirmed in both the jejunum and ileum in the MBCa groups compared to the control group (CaCO3 only).
Figure 2. Absorption of calcium by rat everted jejunal (a) and ileal (b) sacs.
Everted sacs of jejunum and ileum were incubated at indicated concentrations of Ca in serosal fluid for 30 min, and mucosal solutions were analyzed for calcium.□ Calcium carbonate, ■ Calcium maltobionateValues are presented as means ± SE (n = 6). Means in the calcium carbonate groups or calcium maltobionate groups not sharing the same superscript letter are significantly different (p< 0.05). *Significantly different from the Calcium carbonate group of respective calcium concentration (p< 0.05).F value (P value) for jejunum: Factor 1: 92.7 (p< 0.001), Factor 2: 36.6 (p< 0.001), interaction: 20.6 (p< 0.001)F value (P value) for ileum: Factor 1: 54.4 (p< 0.001), Factor 2: 11.9 (p< 0.001), interaction: 5.06 (p= 0.013)Factor 1: Calcium source, Factor 2: Ca concentration
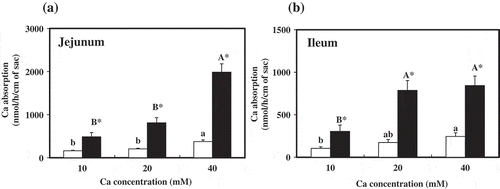
Discussion
Ca and Mg present in food are solubilized by gastric acid, and Ca is primarily absorbed via active transport mediated by binding to proteins (e.g., calbindin D9k and TRPM6) present in the intestinal region spanning the duodenum to the jejunum, whereas Mg is absorbed in the ileum [Citation15]. Nonetheless, gastric acid is neutralized by pancreatic and intestinal fluids as digesta travel to the lower portions of the intestinal tract, and as pH rises, Ca forms nearly insoluble salts with other locally present substances such as phosphate, thereby nearly fully avoiding absorption. Shiga et al. reported that pH of samples of digesta collected throughout rats’ gastrointestinal tract decreased to approximately 3.5 in the stomach but rose to approximately 8.2 by the time the digesta reached the ileum [Citation16]. Ca and Mg absorption in the small intestine substantially depends on the solubility of the compounds containing these elements during transit through the intestines. As average pH of ileal content in the MBCa-100 group was pH 7.71 in this experiment, increased absorption by MB was not simply due to decreased pH not but increased solubility of minerals in the presence of MB even at higher pH than 7.0 [Citation9].
The solubility of CaCO3 added as a supplement into the standard diet given to the control group in Exp. 1 was low because this compound remains an insoluble salt in a basic-pH environment. Thus, in vivo Ca and Mg retention rates were expected to be low. Because of the effective insolubility of CaCO3, the Ca solubilization rate was significantly higher in the groups for which the amount of MBCa incorporated into the diet exceeded 25% (of CaCO3 in the control), as compared to the control group across all periods ()). Furthermore, Ca concentration in the femur was significantly higher in the groups for which the amount of MBCa incorporated into the diet exceeded 50% (of CaCO3 in the control), in comparison with the control group. Therefore, a Ca absorption-enhancing effect was assumed to take place, leading to more efficient absorption of Ca by the intestines and by bone tissue without excretion from the body as a result of replacement (with MBCa) of even a fraction of the CaCO3 contained in the diet. The in vivo Mg retention rate was also significantly higher 2 weeks after initiation of the diet, as a result of the replacement of 25% or more of CaCO3 with MBCa ()). Mg is known to be closely involved in Ca metabolism in processes such as normal bone metabolism and regulation of Ca concentration in the body, and maltobionic acid has been found to enhance not only Ca absorption but Mg absorption as well.
In another study, in an in situ ligated rat jejunal loop absorption test, the Ca component of MBCa was selectively absorbed, and the maltobionic acid component was confirmed to have remained almost entirely unabsorbed [Citation9]. Undigestible carbohydrates such as water-soluble dietary fiber and oligosaccharides are fermented by the gut microflora present in the large intestine, resulting in the production of SCFAs such as acetate, propionate, and n-butyric acid or organic acids such as lactate and succinate; the presence of these compounds has been reported to accelerate intestinal absorption of Ca and Mg by increasing the acidity of the intestinal lumen via an increase in the quantities of solubilized minerals in the intestinal tract [Citation17–Citation19]. In a rat model of gastrectomy in which dissolution of undigestible substances by gastric acid is suppressed, indigestible saccharides such as fructo-oligosaccharides [Citation20], difructose dianhydride III [Citation21,Citation22], and guar gum hydrolysate [Citation23], promote fermentation and solubilization in the large intestine to mitigate decreases in Ca absorption.
No significant decreases in cecal pH were observed as a result of ingestion of MBCa, and in contrast to the typical absorption-promoting effect of fructo-oligosaccharides (and other indigestible oligosaccharides) toward minerals, unabsorbed maltobionic acid after ingestion of MBCa was assumed to remain in the intestinal tract and to continue to maintain the solubility of Ca and Mg ions, thus increasing the efficiency of absorption of these ions throughout the intestinal tract [Citation9]. Our results supporting these conclusions include the finding that the rates of Ca and Mg solubilization in small intestinal and cecal contents were significantly higher compared to the control group after ingestion of MBCa. The finding of higher Ca absorption rates overall suggests that it is necessary to increase intestinal Ca absorption above the normal level to satisfy physiological Ca demand during a mildly Ca-restricted diet.
Exp. 2 evaluated in vitro Ca absorption kinetics in the rat jejunum and ileum by the everted-sac method. Although a peak in intestinal absorption was observed in the CaCO3 control group, in the MBCa groups, intestinal Ca absorption was higher in a concentration-dependent manner, i.e., significantly greater Ca absorption rates were observed as compared to the control group at all Ca concentrations (). These results support the conclusion that the mechanism by which maltobionic acid increases Ca absorption is a shift of Ca to soluble compounds from poorly soluble compounds present in the intestinal tract. The reason why Ca absorption in vitro is poorer as compared to in vivo will be the absence of solubilization step in the stomach in everted sac experiment.
Additionally, significant increases in both cecal tissue weight and weight of cecal content were observed after ingestion of MBCa. This phenomenon is commonly observed after ingestion of indigestible saccharides associated with high levels of intestinal fermentation [Citation24,Citation25]. Maltobionic acid transferred from the small intestine to the large intestine contributes to intestinal fermentation; in our study, significant increases in the amounts of acetate, propionate, and n-butyric acid in the cecum were observed relative to the control. n-Butyric acid is known to exert a growth-promoting effect on enteric epithelial cells, and the unabsorbed maltobionic acid component of MBCa contributes to the maintenance of the enteric environment via promotion of intestinal fermentation.
To summarize the above results, our findings in this study clearly indicate that replacement of even a fraction of poorly absorbable CaCO3 with MBCa in diet can result in a marked absorption-enhancing effect on both Ca and Mg ions as well as increased quantities of Ca in the femur. These results are likely to be the consequence of more efficient Ca and Mg absorption because of the maintenance of Ca and Mg in soluble compounds throughout the intestinal tract owing to ingestion of maltobionic acid. Further studies are needed for a more comprehensive understanding of the Ca absorption-increasing properties of MBCa.
Author contributions
Daiki Suehiro, Ken Fukami, and Takashi Hayakawa designed the study. Daiki Suehiro drafted the manuscript, and Daiki Suehiro and Takashi Hayakawa completed the manuscript. Ken Fukami and Tomoyuki Nakagawa reviewed the manuscript. Misato Okada took part in the analyses. All the authors reviewed and approved the final manuscript.
Disclosure statement
Daiki Suehiro and Ken Fukami are employees of San-ei Sucrochemical Co., Ltd.
References
- Cumming RG, Nevitt MC. Calcium for prevention of osteoporotic fractures in postmenopausal women. J Bone Miner Res. 1997;12:1321–1329.
- Welten DC, Kemper HC, Post GB, et al. A meta-analysis of the effect of calcium intake on bone mass in young and middle aged females and males. J Nutr. 1995;125:2802–2813.
- Yoshimura N, Muraki S, Oka H, et al. Prevalence of knee osteoarthritis, lumbar spondylosis, and osteoporosis in Japanese men and women: the research on osteoarthritis/osteoporosis against disability study. J Bone Miner Metab. 2009;27:620–628.
- Ohta H, Uenishi K, Shiraki M, et al. Recent nutritional trends of calcium and vitamin D in East Asia. Osteoporos Sarcopenia. 2016;2:208–213.
- Nakamura K, Kurahashi N, Ishihara J, et al. Calcium intake and the 10-year incidence of self-reported vertebral fractures in women and men: the Japan public health centre-based prospective study. Br J Nutr. 2009;101:285–294.
- Levenson DI, Bockman RS. A review of calcium preparations. Nutr Rev. 1994;52:221–232.
- Spencer H, Scheck J, Lewin I, et al. Comparative absorption of calcium from calcium gluconate and calcium lactate in man. J Nutr. 1966;89:283–292.
- Hämäläinen MM. Bone repair in calcium-deficient rats: comparison of xylitol+calcium carbonate with calcium carbonate, calcium lactate and calcium citrate on the repletion of calcium. J Nutr. 1994;124:874–881.
- Suehiro D, Okada M, Fukami K, et al. Effect of calcium maltobionate on enhancement of calcium absorption in rats. Luminacoids Res. 2017;21:1–7. (in Japanese).
- Lopez HW, Coudray C, Bellanger J, et al. Intestinal fermentation lessens the inhibitory effects of phytic acid on mineral utilization in rats. J Nutr. 1998;128:1192–1198.
- Fukami K, Kawai K, Takeuchi S, et al. Effect of water content on the glass transition temperature of calcium maltobionate and its application to the characterization of non-arrhenius viscosity behavior. Food Biophysics. 2016;11:410–416.
- Rémésy C, Démigne C. Determination of volatile fatty acids in plasma after ethanolic extraction. Biochem J. 1974;141:85–91.
- Harold V, Connerty MD, Anglis R, et al. Determination of serum calcium by means of orthocresolphthalein complexone. J Clin Pathol. 1966;45:290–296.
- Wilson TH, Wiseman G. The use of sacs of everted small intestine for the study of the transference of substances from the mucosal to the serosal surface. J Physiol. 1954;123:116–125.
- Lameris A, Nevalainen P, Reijnen D, et al. Segmental transport of Ca2⁺ and Mg2⁺ along the gastrointestinal tract. Am J Physiol Gastrointest Liver Physiol. 2015;308:206–216.
- Shiga A, Sasaki T, Horii N. Correlations among pH and Mg, Ca, P, Na, K, Cl- and HCO3- contents of digesta in the gastrointestinal tract of rats. Nihon Juigaku Zasshi. 1987;49:973–979. (in Japanese).
- Yanahira S, Morita M, Aoe S, et al. Effect of lactitol-oligosaccharides on calcium and magnesium absorption in rats. J Nutr Sci Vitaminol. 1997;43:123–132.
- Levrat M, Rémésy C, Démigne C. High propionic acid fermentations and mineral accumulation in the cecum of rats adapted to different levels of inulin. J Nutr. 1991;121:1730–1737.
- Lutz T, Scharrer E. Effect of short-chain fatty acids on calcium absorption by the rat colon. Exp Physiol. 1991;76:615–618.
- Ohta A, Ohtsuki M, Hosono A, et al. Dietary fructooligosaccharides prevent osteopenia after gastrectomy in rats. J Nutr. 1998;128:106–110.
- Shiga K, Nishimukai M, Tomita F, et al. Ingestion of difructose anhydride III, a non-digestible disaccharide, imporoves postgastrectomy osteopenia in rats. Scand J Gastroerol. 2006;30:1165–1173.
- Suzuki T, Hara H, Kasai T, et al. Effects of difructose anhydride III on calcium absorption in small and large intestines of rats. Biosci Biotechnol Biochem. 1998;62:837–841.
- Hara H, Suzuki T, Kasai T, et al. Ingestion of guar gum hydrolysate, a soluble fiber, increases calcium absorption in totally gastrectomized rats. J Nutr. 1999;129:39–45.
- Oku T, Nakamura S. Digestion, absorption, fermentation, and metabolism of functional sugar substitutes and their available energy. Pure Appl Chem. 2002;74:1253–1261.
- Sakata T. Stimulatory effect of short-chain fatty acids on epithelial cell proliferation in the rat intestine: a possible explanation for trophic effects of fermentable fibre, gut microbes and luminal trophic factors. Br J Nutr. 1987;58:95–103.