ABSTRACT
Activation of the adipose lipolytic pathway during lipid metabolism is mediated by protein kinase A (PKA), which responds to β-adrenergic stimulation, leading to increased lipolysis. Soy is well known as a functional food and it is able to affect lipolysis in adipocytes. However, the mechanism by which soy components contribute to the lipolytic pathway remains to be fully elucidated. Here, we show that hydrolyzed soy enhances isoproterenol-stimulated lipolysis and activation of PKA in 3T3-L1 adipocytes. We also found that the expression of β-adrenergic receptors, which coordinate the activation of PKA, is elevated in adipocytes differentiated in the presence of soy hydrolysate. The activity of the soy hydrolysate towards β-adrenergic receptor expression was detected in its hydrophilic fraction. Our results suggest that the soy hydrolysate enhances the PKA pathway through the upregulation of β-adrenergic receptor expression and thereby, increase lipolysis in adipocytes.
GRAPHICAL ABSTRACT

Soy hydrolysate enhances the lipolytic PKA pathway through the upregulation of β-adrenergic receptor expression in adipocytes.
Soy is popular, particularly in Asia, due to its flexibility as a food, and it is widely consumed as a source of vegetable protein and as a component of processed foods. As soy and its hydrolysate contain some components that provide health benefits beyond basic nutrition, soy has also been accepted as functional food [Citation1]. Soy isoflavones, which are secondary metabolites produced during the growth of the soybean plant, are well known as some of the components responsible for the health benefits of soy, and they have been broadly sold as dietary supplements [Citation2]. For instance, the biological activities of genistein, an abundant soy isoflavone, have been reported in metabolic disorders [Citation3].
Lipids are necessary for many normal physiological functions, including energy homeostasis, but they are also linked to numerous pathological processes, such as diabetes and obesity. Obesity occurs as a result of the excess accumulation of fat in insulin-responsive organs, such as adipose tissues and liver. This promotes the development of insulin resistance, a major hallmark of type 2 diabetes [Citation4]. Adipose tissue is a major organ for excess energy storage in the form of triglycerides (TG) [Citation5,Citation6]. In order to control lipid homeostasis, lipid metabolism in adipose tissues is dynamically regulated through the metabolic turnover of lipids in response to the surrounding energy state. This turnover mainly depends on lipolysis and lipogenesis [Citation6]. Therefore, lipolysis, and lipogenesis are tightly coordinated by hormonal signaling, and the disruption of their kinetics within adipose tissue induces lipotoxicity and insulin resistance, which participate in the development and progression of diabetes [Citation7]. Apart from lipid storage, adipose tissue also possesses thermogenic ability conferred by uncoupling protein 1 (UCP1), which contributes to energy homeostasis [Citation8].
There have been reports on the beneficial effects of dietary soy protein, as well as its hydrolysate, in reducing body fat and lowering plasma TG and low-density lipoprotein cholesterol levels in obese subjects [Citation9–Citation11]. These findings suggest the involvement of soy and its components in physiological lipid turnover. A more recent study has shown that hydrolyzed soy proteins stimulate the lipid catabolic pathway, i.e. lipolysis in adipocytes in vitro [Citation12]. However, the mechanisms by which soy and its hydrolysate contribute to lipid metabolism are not yet fully understood.
In the present study, we focused on the effect of soy hydrolysate on the β-adrenergic agonist-stimulated lipolytic pathway and attempted to understand the underlying mechanism mediating the link between soy hydrolysate and lipolysis. We showed that soy hydrolysate indeed enhanced β-adrenergic agonist-stimulated lipolysis in 3T3-L1 adipocytes, by increasing the activation of the protein kinase A (PKA) pathway. Isoproterenol (nonselective β-adrenergic agonist)-induced phosphorylation of PKA target molecules, such as hormone-sensitive lipase (HSL) and perilipin and the PKA-mediated expression of Ucp1, were enhanced in adipocytes differentiated in the presence of soy hydrolysate. We further demonstrated that soy hydrolysate increased the expression of β-adrenergic receptors and this effect was mediated by the hydrophilic components of the hydrolysate. Our results suggest that soy hydrolysate affects lipolysis and functions via the PKA pathway by acting on the expression of β-adrenergic receptors.
Materials and methods
Materials and cell culture
The soy hydrolysate used in this study was Hinute-AM, purchased from Fuji Oil Co. (Tokyo, Japan) [Citation13,Citation14]. A working stock solution (0.1 g/mL) was prepared by dissolving the soy hydrolysate in Milli-Q water (Millipore, Burlington, MA, USA) and then filtering it through a 0.22 μm polyethersulfone filter for sterilization.
Isoproterenol, forskolin, and H89 were purchased from Nacalai Tesque (Kyoto, Japan) or Sigma-Aldrich (St Louis, MO, USA).
3T3-L1 preadipocytes were cultured and differentiated to adipocytes, as described previously [Citation15]. Different concentrations of the soy hydrolysate were added throughout the differentiation period.
Lipid analysis
Oil Red O staining was performed to evaluate TG accumulation, as described previously [Citation16].
To investigate the lipolytic activity of the adipocytes, differentiated 3T3-L1 cells were first starved of serum for 3–5 h, after which isoproterenol was added for 1 h to stimulate lipolytic activity. Non-esterified fatty acid (NEFA) and glycerol levels within the cell culture medium were measured using NEFA C (Wako, Osaka, Japan) and Triglyceride G (Wako) assay kits, respectively.
Western blotting
Cells were washed with PBS and lysed with lysis buffer containing 20 mM Tris-HCl buffer (pH 7.5), 1% (v/v) Triton X-100, 10% (v/v) glycerol, 137 mM NaCl, 2 mM EDTA (pH 8.0), a phosphatase inhibitor cocktail, and a protease inhibitor cocktail (Nacalai Tesque). The lysate was centrifuged at 16,700 g for 10 min and the resulting supernatant was collected. Protein concentration was measured using the DC Protein Assay (Bio-Rad, Hercules, CA, USA). Denatured protein was separated by 10% SDS-PAGE and transferred to a polyvinylidene difluoride transfer membrane. The membrane was then blocked, washed, and incubated with appropriate dilutions of the primary antibodies (anti-phospho-HSL Ser660, #4107; anti-HSL, #4126; anti-perilipin, #9349; and anti-phospho-PKA substrate, #9624 [Cell Signaling, Danvers, MA, USA]). Immunoreactive bands were visualized with Immobilon Western Chemiluminescent Horseradish Peroxidase Substrate (Millipore) using an LAS-4000 mini-imaging system (Fujifilm, Tokyo, Japan).
RNA preparation and quantification of gene expression
To quantify mRNA expression, real-time PCR was performed using SYBR Green chemistry, as described previously [Citation15]. All mRNA expression levels were normalized to the expression levels of a 36b4 internal control. The primer sequences used in this study are listed in .
Table 1. Oligonucleotide primers used for mRNA analysis.
Chromatographic fractionation
The soy hydrolysate was fractionated by reverse-phase HPLC with a 5C18-AR-II octadecylsilyl column (Nacalai Tesque), using a mobile phase of water (solvent A) and acetonitrile (solvent B), with 0.1% v/v formic acid added to both solvents. The program began with 1% solvent B in solvent A, followed by a linear elution gradient from 1% to 100% solvent B in solvent A for 30 min and a maintenance of 100% solvent B for an additional 15 min. HPLC elution was monitored using a diode array detector in the range of 200–400 nm. The flow rate was set at 1.0 mL/min. Fractions eluted from 1 to 5 min formed Frc.1; fractions eluted from 6 to 11 minutes formed Frc.2; fractions eluted from 12 to 20 min formed Frc.3; and finally, fractions eluted from 21 to 45 min formed Frc.4. Each of these eluted fractions was then vacuum freeze-dried and re-dissolved in Milli-Q water which is the same as the injection volume. For β2AR (Adrb2) expression assay, the concentration of soy hydrolysate components within each fraction was made to correspond to the concentration found within the 0.5 mg/mL soy hydrolysate control.
LC/MS analysis
Metabolome analysis using LC/MS was performed as previously described [Citation17,Citation18] with slight modifications. Briefly, LC-MS was performed with a Nexera X2 HPLC system (Shimadzu GLC Ltd., Tokyo, Japan) coupled to a Q Exactive Plus Orbitrap system (Thermo Fisher Scientific Inc., San Jose, CA). These data were acquired with X-Calibur and Compound Discoverer 2.1 (Thermo Fisher Scientific Inc.).
Statistical analysis
The statistical significance of differences between the mean values was analyzed using a one-way ANOVA followed by Tukey’s test or Student’s t-test. Differences were considered significant at P < 0.05.
Results and discussion
Effect of soy hydrolysate on isoproterenol-stimulated lipolysis
Isoproterenol, a nonselective β-adrenergic agonist, stimulates the lipolytic pathway and sequentially increases the release of NEFA and glycerol into the medium from adipocytes [Citation19]. To investigate a possible role of soy hydrolysate (Hinute-AM) in the lipolytic pathway in adipocytes, we measured NEFA and glycerol following treatment with isoproterenol. The amounts of NEFA and glycerol released after isoproterenol stimulation were higher in 3T3-L1 adipocytes differentiated in the presence of the soy hydrolysate than in those differentiated in the absence of the soy hydrolysate (). Meanwhile, the soy hydrolysate did not have a significant effect on the amounts of NEFA and glycerol released under unstimulated conditions. These findings suggest that the soy hydrolysate enhances isoproterenol-stimulated lipolysis in adipocytes.
Figure 1. Soy hydrolysate enhanced the isoproterenol-stimulated lipolytic pathway in 3T3-L1 adipocytes.
(a, b) 3T3-L1 cells were differentiated in the presence of 0 (vehicular control), 0.1, or 0.5 mg/mL soy hydrolysate. Cells were then treated with 10 μM isoproterenol for 1 h before glycerol (a) and NEFA (b) levels in the culture medium were measured. Data are presented as mean ± SEM (error bars), with n = 4 per group. Bars with different letters represent significant differences (P < 0.05) by a one-way ANOVA followed by Tukey’s test. (c) 3T3-L1 cells differentiated in the presence of the soy hydrolysate were treated with 1 or 10 µM isoproterenol for 30 min. Phosphorylation of HSL (p-HSL), protein levels of HSL, phosphorylation of perilipin (p-perilipin), and protein levels of perilipin were determined using anti-phospho HSL Ser660 antibodies, anti-HSL antibodies, anti-phospho-PKA substrate antibodies, and anti-perilipin antibodies, respectively. (d, e) Phosphorylation levels of HSL and perilipin were quantified in cells treated with (+) or without (-) 10 μM isoproterenol for 30 min. The intensity of immunoreactive bands was quantified using ImageJ. The ratio of p-HSL/HSL (d) or p-perilipin/perilipin (e) in unstimulated cells was assigned a relative value of 100. Data are presented as mean ± SEM (error bars), with n = 3–5 per group. (f) 3T3-L1 cells were differentiated with (+) or without (-) 0.5 mg/mL soy hydrolysate. mRNA expression levels of Ucp1 in the cells treated with (+) or without (-) 10 μM isoproterenol for 4 h were determined by real-time PCR. Data are presented as mean ± SEM (error bars), with n = 4 per group. *, P < 0.05 between the non-stimulated vehicular control group and the isoproterenol-stimulated groups. #, P < 0.05.
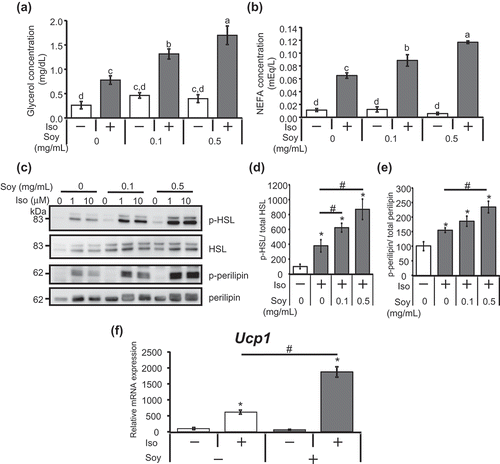
Stimulation of β-adrenergic receptors (βAR) on adipocytes by adrenergic agonists results in the activation of protein kinase A (PKA), which is associated with elevated levels of cAMP [Citation20]. Activated PKA then directly phosphorylates its target proteins, including HSL and perilipin (a protein located on the lipid droplet surface), which are crucial for the activation of lipolysis [Citation21–Citation23]. To determine whether the soy hydrolysate influences the PKA signaling pathway, we examined the phosphorylation levels of HSL and perilipin as indicators of PKA activity. As shown in , the isoproterenol-stimulated phosphorylation of HSL at Ser660, a target site of PKA [Citation21], was increased in adipocytes differentiated in the presence of the soy hydrolysate. Perilipin has multiple PKA consensus sequences and its phosphorylation level can be detected using an anti-phospho-PKA substrate antibody [Citation23,Citation24]. The soy hydrolysate also enhanced the isoproterenol-stimulated phosphorylation of perilipin (). PKA activated by adrenergic agonists has an impact, not only on lipolysis, but also on the expression of genes, including uncoupling protein 1 (Ucp1), which is a fatty-acid anion/H+ symporter and is involved in non-shivering thermogenesis in adipose tissues [Citation25]. Isoproterenol stimulation increases the expression of Ucp1 via PKA and cAMP response element-binding protein (CREB) in 3T3-L1 adipocytes [Citation26]. We found that the incubation of adipocytes with soy hydrolysate significantly elevated the isoproterenol-increased expression of Ucp1 (). These results indicate that the soy hydrolysate enhances the isoproterenol-stimulated activation of the PKA pathway.
Enhancement of the lipolytic pathway by the soy hydrolysate is dependent on PKA
To clarify the contribution of PKA to the enhancement of the lipolytic pathway by the soy hydrolysate during isoproterenol-stimulated lipolysis, we measured NEFA and glycerol in the presence of H89, a PKA inhibitor. As shown in , pretreatment with H89 significantly decreased the release of NEFA and glycerol from adipocytes differentiated in the presence of the soy hydrolysate. Additionally, the effect of the soy hydrolysate on the isoproterenol-stimulated phosphorylation of HSL was depressed by pretreatment with H89 (). These results indicate that the enhancement of isoproterenol-stimulated lipolysis by the soy hydrolysate is dependent on the PKA pathway.
Figure 2. Enhancement of the isoproterenol-stimulated lipolytic pathway by soy hydrolysate in 3T3-L1 adipocytes is dependent on PKA.
(a, b) 3T3-L1 cells were differentiated with (+) or without (-) 0.5 mg/mL soy hydrolysate. The cells were pre-treated with 20 μM H89 for 30 min, prior to 10 μM isoproterenol treatment (1h). Glycerol (a) and NEFA (b) levels in the culture medium were then measured. (c, d) Cells were pre-treated with 20 μM H89 for 30 min before treatment with 10 μM isoproterenol for 30 min. Detection of HSL phosphorylation (c) and quantification of HSL phosphorylation levels (d) were performed, as described in the legend of ). Data are presented as mean ± SEM (error bars), with n = 4 per group for the glycerol and NEFA assays and n = 3 per group for relative HSL phosphorylation measurements. *, P < 0.05 between the non-stimulated vehicular control group and the isoproterenol-stimulated groups. #, P < 0.05.
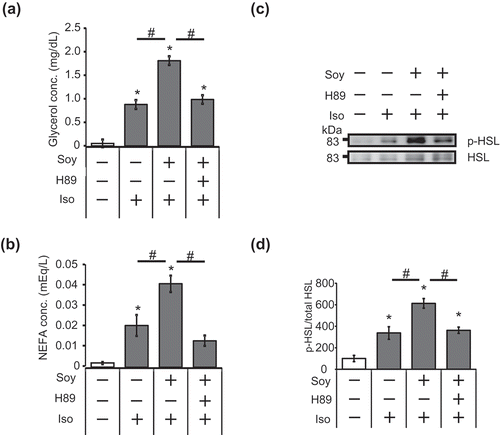
Soy hydrolysate increases the expression of βar
We next examined the mechanism whereby the soy hydrolysate enhances the isoproterenol-stimulated activation of the PKA pathway. In adipocytes, three βAR subtypes (β1AR, β2AR, and β3AR) contribute to increased cAMP production, leading to the activation of PKA [Citation20]. An increase in β2AR (Adrb2) and β3AR (Adrb3) expression is associated with the progression of adipocyte differentiation [Citation27], whereas β1AR expression is not significantly increased during adipocyte differentiation [Citation28]. A previous study has shown that soluble soy peptides facilitate adipocyte differentiation via the up-regulation of peroxisome proliferator-activated receptor γ (PPARγ), an essential regulator of adipocyte differentiation [Citation16]. These findings raise the possibility that the soy hydrolysate may exert its effect on the lipolytic pathway through an increase in βAR expression by facilitating adipocyte differentiation. To explore this possibility, we first confirmed the role of the soy hydrolysate used in our study in adipocyte differentiation. As shown in , the level of TG accumulation in adipocytes incubated with the soy hydrolysate was higher throughout the differentiation period, compared to the differentiated control adipocytes. Furthermore, expression of the adipocyte differentiation markers, Pparγ and adipocyte protein 2 (aP2), was increased in adipocytes differentiated with the soy hydrolysate (). We next investigated whether the soy hydrolysate influences the expression of β2AR and β3AR. β2AR expression was significantly higher in adipocytes differentiated with the soy hydrolysate than in those differentiated without the soy hydrolysate (). The soy hydrolysate also showed a tendency to increase β3AR expression (P = 0.08, ). Forskolin elevates cAMP levels and subsequently, PKA activity, through a βAR-independent manner. If the effect of the soy hydrolysate on the lipolytic pathway was due to an increase in βAR expression, the forskolin-induced activation of PKA would not be affected by the soy hydrolysate. As expected, the soy hydrolysate did not significantly affect HSL phosphorylation following treatment with forskolin (). These findings strongly suggest that the enhanced effect of the soy hydrolysate on the PKA pathway was exerted by the upregulation of β2AR and β3AR expression.
Figure 3. Soy hydrolysate enhanced the lipolytic pathway by increasing βAR expression during adipocyte differentiation.
(a) 3T3-L1 cells were left undifferentiated (undiff.) or were differentiated with (+) or without (-) 0.5 mg/mL soy hydrolysate. After differentiation, the cells were fixed with formalin before being stained with Oil Red O and observed using a microscope. Bar, 200 μm. (b) Oil Red O was extracted from the cells with isopropanol and the absorbance was measured at 490 nm. (c–f) Expression levels of Pparγ (c), aP2 (d), β2AR (Adrb2) (e), and β3AR (Adrb3) (f) were measured. Data are presented as mean ± SEM (error bars), with n = 3 per group for Oil Red O staining and n = 4 per group for mRNA expression. *, P < 0.05 between the undifferentiated group and the differentiated groups. #, P < 0.05. (g, h) 3T3-L1 cells differentiated with (+) or without (-) 0.5 mg/mL soy hydrolysate were treated with 10 μM isoproterenol (Iso) or 20 μM forskolin (For) for 30 min. HSL phosphorylation was detected (g) and quantified (h), as described in the legend of . Data are presented as mean ± SEM (error bars), with at n = 4 per group. *, P < 0.05 between the non-stimulated vehicular control group and the stimulated groups. #, P < 0.05.
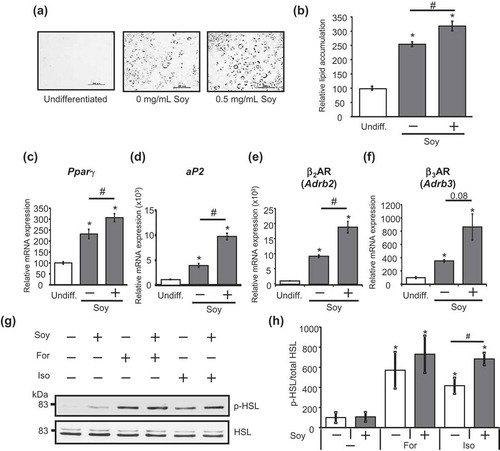
Hydrophilic components of the soy hydrolysate are responsible for its effect on the lipolytic pathway
To gain further insight into the effect of the soy hydrolysate on the increase in βAR expression, we aimed to determine the character of the components in the soy hydrolysate that are responsible for the effect on the lipolytic pathway by fractionation using column chromatography. The soy hydrolysate was fractionated using a C18 column, under reverse-phase HPLC conditions and was separated into four fractions (Frc.1–Frc.4, ). We then determined the effect of each fraction on the expression of βAR. As shown in , treatment with Frc.2 resulted in increased β2AR expression levels. These results suggest that the components of the soy hydrolysate that are responsible for the effects on the lipolytic pathway are relatively hydrophilic substances.
Figure 4. Fraction 2 of soy hydrolysate increased β2AR (Adrb2) expression.
(a) Schematic flow of the fractionation of the soy hydrolysate, which is described in the “Materials and methods” section. (b) Expression levels of β2AR (Adrb2) were measured in undifferentiated adipocytes (Undiff.), in adipocytes differentiated with (+) or without (-) 0.5 mg/mL soy hydrolysate, and in adipocytes differentiated with fractions 1–4 (Frc.1–4). Data are presented as mean ± SEM (error bars), with n = 4 per group. *, P < 0.05 between the vehicular control differentiated group and the other groups.
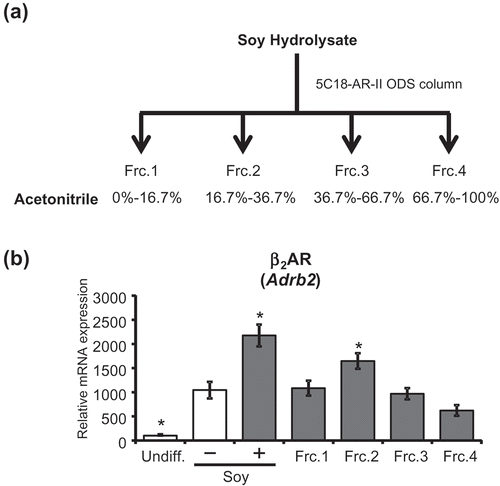
Soy and its hydrolysate are classified as functional foods and they provide health benefits that include the improvement of lipid metabolism in obese subjects [Citation1]. We analyzed the physiological function of soy hydrolysate in the lipolytic pathway in adipocytes. We found that incubating adipocytes with soy hydrolysate, during the process of adipocyte differentiation, enhanced β-adrenergic agonist-stimulated lipolysis through increased PKA activation. The involvement of soy hydrolysate in the lipolytic pathway has also been previously demonstrated in vitro [Citation12,Citation29]. The treatment of mature adipocytes with hydrolyzed soy proteins decreases their TG content, suggesting that hydrolyzed soy proteins directly stimulate the lipolytic pathway [Citation12]. However, as shown in , incubation of adipocytes with soy hydrolysate during the process of adipocyte differentiation conferred no obvious effect on the basal levels of lipolysis. Instead, we found that the soy hydrolysate used in this study elevated the levels of β2AR and β3AR expression, while facilitating adipocyte differentiation () and thereby, contributed to the activation of the PKA pathway stimulated by isoproterenol (). These results suggest that the soy hydrolysate also possesses the ability to indirectly modulate the lipolytic pathway.
Genistein, one of the soy isoflavones, is well known for its health benefits, which include preventing certain cancers, osteoporosis, cardiovascular diseases, and metabolic diseases [Citation30]. Recent studies have indicated the involvement of genistein in adipocyte differentiation; however, these studies remain controversial because different groups have shown opposite effects [Citation31]. The soy hydrolysate used in this study promoted adipocyte differentiation (), which is consistent with a previous study [Citation16], implying that the bioactive substances of soy, including genistein, are most likely present in this hydrolysate. Therefore, these findings raise the possibility that genistein is responsible for the soy hydrolysate-increased activation of the lipolytic pathway. However, LC/MS analysis showed that the relatively hydrophilic fraction, Frc.2, which was responsible for the increased β2AR expression (), contained a very small amount of genistein compared to the relatively hydrophobic fraction, Frc.3 (data not shown). Consequently, the contribution of genistein in the soy hydrolysate to the effects on the lipolytic pathway in adipocytes appears to be minor. On the other hand, it is known that the soy hydrolysate in this study, i.e., Hinute-AM, abound with dipeptides, tripeptides, and free amino acids, and they account for 60% or more of the total nitrogen in Hinute-AM [Citation13,Citation14]. Our LC/MS analysis revealed that the peptides account for 12.2% of annotated compounds in Frc.2, and which consist of five kinds of tripeptides and 62 kinds of dipeptides. The functionality of soy proteins and their peptides in regulating metabolic activity has previously been reported [Citation32], implying that these peptides in the hydrophilic fraction may contribute to the effects on the lipolytic pathway. Further investigations are needed to identify the specific components in the hydrophilic fractions that are involved in the activation of the lipolytic pathway.
Adipose tissue is no longer considered to be a static tissue that stores fat. White adipose tissue (WAT) is a typical adipose tissue for fat storage. It stores energy in the form of TG during periods of excess energy intake, while releasing it in the form of NEFA during fasting conditions [Citation33]. On the other hand, brown adipose tissue (BAT) is specialized for heat production (non-shivering thermogenesis) and dissipates energy in the form of heat, using fatty acids as a substrate, through the uncoupling of oxidative phosphorylation by mitochondrial UCP1 [Citation8,Citation25]. Cold exposure and adrenergic stimulation are well-known factors that increase heat production in BAT. However, these stimuli also induce a brown-like thermogenic phenotype in WAT (generally called “browning” [Citation34],). Notably, we found that the soy hydrolysate in this study enhanced the expression of Ucp1, following treatment with isoproterenol (). The increased thermogenic ability of adipose tissues is expected to protect against obesity and obesity-related metabolic disorders. Moreover, the soy hydrolysate was also reported to have high absorption efficiency in clinical studies [Citation13,Citation14], suggesting that the soy hydrolysate may exert its effect by oral administration. Our finding that the soy hydrolysate affected Ucp1 expression may open up new possibilities for the development of soy component-based therapies for obesity.
Author contribution
W.N., T.K., and T.G. conceived the project. S.-P.N., W.N., H.T., and T.G. designed the experiments. S.-P.N., S.M., and H.N. performed the experiments. S.-P.N., W.N., S.M., H.T., H.-F.J., T.A., T.K., and T.G. discussed the results. S.-P.N. and W.N. wrote the manuscript, and all authors revised the manuscript before submission.
Acknowledgments
We are grateful to T.K. lab members for their technical support and helpful discussions.
Disclosure statement
No potential conflict of interest was reported by the authors.
Additional information
Funding
References
- Xiao CW. Health effects of soy protein and isoflavones in humans. J Nutr. 2008;138:1244S–1249S.
- Setchell KD, Brown NM, Desai P, et al. Bioavailability of pure isoflavones in healthy humans and analysis of commercial soy isoflavone supplements. J Nutr. 2001;131:1362S–1375S.
- Mukund V, Mukund D, Sharma V, et al. Genistein: its role in metabolic diseases and cancer. Crit Rev Oncol Hematol. 2017;119:13–22.
- Pi-Sunyer XF. Clinical guidelines on the identification, evaluation and treatment of overweight and obesity in adults-the evidence report. Obes Res. 1998;6:51S–210S.
- Rosen ED, Spiegelman BM. Adipocytes as regulators of energy balance and glucose homeostasis. Nature. 2006;444:847–853.
- Ducharme NA, Bickel PE. Minireview: lipid droplets in lipogenesis and lipolysis. Endocrinology. 2008;149:942–949.
- Arner P, Bernard S, Salehpour M, et al. Dynamics of human adipose lipid turnover in health and metabolic disease. Nature. 2011;478:110–113.
- Feldmann HM, Golozoubova V, Cannon B, et al. UCP1 ablation induces obesity and abolishes diet-induced thermogenesis in mice exempt from thermal stress by living at thermoneutrality. Cell Metab. 2009;9:203–209.
- Aoyama T, Fukui K, Takamatsu K, et al. Soy protein isolate and its hydrolysate reduce body fat of dietary obese rats and genetically obese mice (yellow KK). Nutrition. 2000;16:349–354.
- Allison DB, Gadbury G, Schwartz LG, et al. A novel soy-based meal replacement formula for weight loss among obese individuals: a randomized controlled clinical trial. Eur J Clin Nutr. 2003;57:514–522.
- Moriyama T, Kishimoto K, Nagai K, et al. Soybean β-conglycinin diet suppresses serum triglyceride levels in normal and genetically obese mice by induction of β-oxidation, downregulation of fatty acid synthase, and inhibition of triglyceride absorption. Biosci Biotechnol Biochem. 2004;68:352–359.
- Tsou MJ, Lin SB, Chao CH, et al. Enhancing the lipolysis-stimulating activity of soy protein using limited hydrolysis with Flavourzyme and ultrafiltration. Food Chem. 2012;134:1564–1570.
- Maebuchi M, Samoto M, Kohno M, et al. Improvement in the intestinal absorption of soy protein by enzymatic digestion to oligopeptide in healthy adult men. Food Sci Technol Res. 2007;13:45–53.
- Kohno M. Soybean protein and peptide as complementation medical food materials for treatment of dyslipidemia and inflammatory disorders. Food Sci Technol Res. 2017;23:773–782.
- Goto T, Lee JY, Teraminami A, et al. Activation of peroxisome proliferator-activated receptor-alpha stimulates both differentiation and fatty acid oxidation in adipocytes. J Lipid Res. 2011;52:873–884.
- Goto T, Mori A, Nagaoka S. Soluble soy protein peptic hydrolysate stimulates adipocyte differentiation in 3T3-L1 cells. Mol Nutr Food Res. 2013;57:1435–1445.
- Takahashi H, Goto T, Yamazaki Y, et al. Metabolomics reveal 1-palmitoyl lysophosphatidylcholine production by peroxisome proliferator-activated receptor α. J Lipid Res. 2015;56:254–265.
- Takahashi H, Sanada K, Nagai H, et al. Over-expression of PPARα in obese mice adipose tissue improves insulin sensitivity. Biochem Biophys Res Commun. 2017;493:108–114.
- Anthonsen MW, Rönnstrand L, Wernstedt C, et al. Identification of novel phosphorylation sites in hormone-sensitive lipase that are phosphorylated in response to isoproterenol and govern activation properties in vitro. J Biol Chem. 1998;273:215–221.
- Carmen GY, Víctor SM. Signalling mechanisms regulating lipolysis. Cell Signal. 2006;18:401–408.
- Holm C. Molecular mechanisms regulating hormone-sensitive lipase and lipolysis. Biochem Soc Trans. 2003;31:1120–1124.
- Miyoshi H, Souza SC, Zhang HH, et al. Perilipin promotes hormone-sensitive lipase-mediated adipocyte lipolysis via phosphorylation-dependent and -independent mechanisms. J Biol Chem. 2006;281:15837–15844.
- Brasaemle DL, Subramanian V, Garcia A, et al. Perilipin A and the control of triacylglycerol metabolism. Mol Cell Biochem. 2009;326:15–21.
- Lu X, Gruia-Gray J, Copeland NG, et al. The murine perilipin gene: the lipid droplet-associated perilipins derive from tissue-specific, mRNA splice variants and define a gene family of ancient origin. Mamm Genome. 2001;12:741–749.
- Bertholet AM, Kirichok Y. UCP1: A transporter for H+ and fatty acid anions. Biochimie. 2017;134:28–34.
- Miller CN, Yang JY, England E, et al. Isoproterenol increases uncoupling, glycolysis, and markers of beiging in mature 3T3-L1 adipocytes. PLoS One. 2015;10:e0138344.
- Monjo M, Pujol E, Roca P. α2- to β3-Adrenoceptor switch in 3T3-L1 preadipocytes and adipocytes: modulation by testosterone, 17β-estradiol, and progesterone. Am J Physiol Endocrinol Metab. 2005;289:E145–E150.
- Guest SJ, Hadcock JR, Watkins DC, et al. β1- and β2-adrenergic receptor expression in differentiating 3T3-L1 cells. Independent regulation at the level of mRNA. J Biol Chem. 1990;265:5370–5375.
- Tsou MJ, Kao FJ, Lu HC, et al. Purification and identification of lipolysis-stimulating peptides derived from enzymatic hydrolysis of soy protein. Food Chem. 2013;138:1454–1460.
- Messina MJ. Legumes and soybeans: overview of their nutritional profiles and health effects. Am J Clin Nutr. 1999;70:439s–450s.
- Chirumbolo S. Genistein as a nature-derived PPAR agonist in adipogenesis and weight gain. Eur J Nutr. 2015;54:489–491.
- Torres N, Torre-Villalvazo I, Tovar AR. Regulation of lipid metabolism by soy protein and its implication in diseases mediated by lipid disorders. J Nutr Biochem. 2006;17:365–373.
- Ahima RS, Flier JS. Adipose tissue as an endocrine organ. Trends Endocrinol Metab. 2000;11:327–332.
- Bartelt A, Heeren J. Adipose tissue browning and metabolic health. Nat Rev Endocrinol. 2014;10:24–36.