ABSTRACT
Abscisic acid (ABA) is one of the plant hormones that regulates physiological functions in various organisms, including plants, sponges, and humans. The biosynthetic machinery in plants is firmly established, while that in fungi is still unclear. Here, we elucidated the functions of the four biosynthetic genes, bcABA1-bcABA4, found in Botrytis cinerea by performing biotransformation experiments and in vitro enzymatic reactions with putative biosynthetic intermediates. The first-committed step is the cyclization of farnesyl diphosphate to give α-ionylideneethane catalyzed by a novel sesquiterpene synthase, BcABA3, which exhibits low amino acid sequence identities with sesquiterpene synthases. Subsequently, two cytochrome P450s, BcABA1 and BcABA2, mediate oxidative modifications of the cyclized product to afford 1ʹ,4ʹ-trans-dihydroxy-α-ionylideneacetic acid, which undergoes alcohol oxidation to furnish ABA. Our results demonstrated that production of ABA does not depend on the nucleotide sequence of bcABA genes. The present study set the stage to investigate the role of ABA in infections.
Graphical abstract
Biosynthetic pathway of a plant hormone abscisic acid produced by phytopathogenic fungi was elucidated through biotransformation experiments.
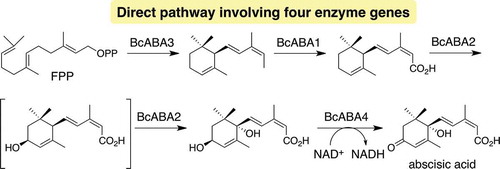
Abscisic acid (ABA, 1) is a sesquiterpene phytohormone that regulates physiological functions in plants and a wide range of organisms from bacteria to humans. For example, 1 mediates adaptive responses towards abiotic stresses such as drought stress and salt stress in plants [Citation1], while it also regulates temperature-induced oxygen consumption and water filtration in sponges [Citation2]. Recent studies have revealed that 1 serves as an activator of human monocytes and vascular smooth muscle cells [Citation3] and that it is a prosurvival factor for megakaryocytes in a Tpo-independent manner [Citation4]. In addition, phytopathogenic fungi, such as Botrytis cinerea [Citation5] and Cercospora species [Citation6], which cause mold diseases, also produce 1 as a virulence factor [Citation7].
The importance of 1 in several organisms has prompted researchers to elucidate its biosynthetic pathway. In plants, ABA biosynthetic pathway has been firmly established through biochemical and molecular genetic studies () [Citation8]. The most notable feature of this pathway is the construction of C15 carbon skeleton by oxidative cleavage of C40 carotenoids derived from β-carotene, imposing complicated biotransformation involving 12 enzyme genes. On the other hand, biosynthesis of 1 in fungi, defined as a direct pathway, is distinct from that in plants [Citation8]. In case of B. cinerea, farnesyl diphosphate (FPP) is directly converted into α-ionylideneethane (2), which harbors a C15 skeleton [Citation9]. Subsequently, 2 undergoes oxidations at C1, C1ʹ, and C4ʹ positions to give 1 (). Previous labeling experiments using 18O2 revealed that molecular oxygen is installed at these positions [Citation9]. The reduction product of 1, 1ʹ,4ʹ-trans-dihydroxy-α-ionylideneacetic acid (5), was also isolated from B. cinerea, while the diastereomeric 1ʹ,4ʹ-cis-dihydroxy-α-ionylideneacetic acid (7) was not [Citation9]. Intermediates 2 and 5 were demonstrated by conversion of [2-13C]-2 and [2H7]-5 into 1 in B. cinerea [Citation10]. In 2006, a putative biosynthetic gene cluster of 1 was identified in B. cinerea [Citation11]. This gene cluster consisted of four genes; two cytochrome P450s, bcABA1 and bcABA2; one short-chain dehydrogenase/reductase, bcABA4; and one functionally unknown enzyme gene, bcABA3. Subsequent gene inactivation experiments of bcABA1-4 revealed that all mutants lacked the ability to produce 1, suggesting that these genes might be responsible for the biosynthesis of 1. However, detailed function of each enzyme remained unclear because of the following reasons; 1) other putative biosynthetic intermediates except 2 and 5 were isolated, and 2) no feeding experiments using putative intermediates were conducted. Especially, little is known about the cyclization enzyme, although circumstantial evidence has suggested that BcABA3 is a potential candidate to catalyze the cyclization.
Previously, we conducted a functional analysis of BcABA3 and characterized the cyclization activity from FPP to 2 [Citation12]. This successful functional analysis enabled us to examine the biosynthetic pathway from 2 to 1 through biotransformation experiments using putative biosynthetic intermediates and in vitro enzymatic reactions. Here, we described the sequence and functional analysis of bcABA genes and revealed step-by-step oxidative modification processes via α-ionylideneacetic acid (3) and 5.
Materials and methods
General
All reagents commercially supplied were used as received. Column chromatography was carried out on 60 N silica gel (Kanto Chemicals). Optical rotations were recorded on JASCO P-2200 digital polarimeter. 1H-NMR spectra were recorded on Bruker DRX-500 or Bruker AMX-500 spectrometer (500 MHz for 1H-NMR). NMR spectra were recorded in CDCl3 (99.8 atom% enriched, Kanto) or CD3OD (99.8 atom% enriched, TCI). 1H chemical shifts were reported in δ value based on residual chloroform (7.26 ppm) and methanol (3.34 ppm) as a reference. GC-MS and UPLC analyses were conducted with an MS-2010 (Shimadzu) and a Waters ACQUITY UPLC, respectively.
Oligonucleotides for polymerase chain reactions (PCRs) were purchased from Hokkaido System Science Co., Ltd. PCRs were performed with a BioRad S1000 thermal cycler. Analysis of the samples during protein purification was performed using SDS-polyacrylamide gel electrophoresis, and the proteins were visualized by using Coomassie Brilliant Blue staining. Protein concentration was determined by the Bradford method with bovine serum albumin as a standard.
Compounds 2, 3, 5, and 7 were synthesized according to the literature procedures [Citation9,Citation13,Citation14].
Strain and culture conditions
Escherichia coli HST08 was used for cloning and following standard recombinant DNA techniques. E. coli BL21-Gold(DE3) was used for protein expression. Botrytis cinerea 306,914 was used for extraction of genomic DNA. A fungal host strain used in this study was A. oryzae NSAR1 [Citation15], a quadruple auxotrophic mutant (niaD−, sC−, ΔargB, adeA−), for fungal expression.
Genomic DNA preparation
Genomic DNA of B. cinerea 306,914 was extracted according to the literature procedure [Citation12].
Construction of A. oryzae expression plasmids
The bcABA2 was amplified from genomic DNA of B. cinerea 306,914 with primer set as shown in Supplemental Table S1. PCR reactions were performed with the KOD-Plus-Neo (TOYOBO). Each PCR product was inserted into the KpnI site of pTAex3 using In-Fusion Advantage PCR cloning kit (Clontech Laboratories) to construct expression plasmid pTAex3-bcABA2. Other plasmids, pTAex3-bcABA1 and pUSA2-bcABA24 were constructed in our previous study [Citation12].
Transformation of Aspergillus oryzae
Transformation of A. oryzae NSAR1 (1.0 × 108 cells) was performed by the protoplast-polyethylene glycol method reported previously to construct following transformants [Citation12]; AO-bcABA1 (plasmids used in the transformation; pTAex3-bcABA1), AO-bcABA2 (pTAex3-bcABA2), and AO-bcABA124 (pTAex3-bcABA1 and pUSA2-bcABA24). AO is an abbreviation of Aspergillus oryzae and AO-bcABAx means a transformant harboring bcABAx gene.
Analysis of the metabolites
Mycelia of AO-bcABA1234 was inoculated into an MPY medium (5 mL) in 20 mL Erlenmeyer flask. The culture was incubated at 30°C for 2 d. The fermentation broth was soaked in acetone (10 mL) for 6 h. After filtration, the filtrate was concentrated in vacuo. The residual water was adjusted to pH 1.0 and then extracted with EtOAc, and the organic layers were concentrated in vacuo. This crude extracts were directly analyzed by a UPLC-MS equipped with an ACQUITY UPLC BEH C18 (ϕ2,1 x 50 mm) at the following conditions (method A): flow rate; 0.7 mL/min, solvent system; acetonitrile in H2O containing 0.1% of formic acid, 0–1 min: 10%; 1–3 min: a linear gradient 10%-95%; 3–5 min: 95%.
cDNA preparation
AO-bcABA124 was grown on MPY medium containing 0.925% (NH4)2SO4, 0.01% adenine for 3–5 d at 30°C. Total RNA was extracted from each dried mycelia using TRIzol® Reagent (Invitrogen) according to the manufacturer’s instructions and then treated with DNase I (Life Technologies) for reverse transcription. Complementary DNA (cDNA) was synthesized with PrimeScriptTM II first strand cDNA synthesis kit (Takara) using the oligo dT primer according to the manufacturer’s instructions. The cDNA was used as a template of the PCR reactions for direct sequencing and subcloning of each STS gene into the pColdI vector.
BcABA4 cloning and expression in Escherichia coli
Intronless bcABA4 was amplified from cDNA with primer set as shown in Supplemental Table S1. PCR reactions were performed with the KOD-Plus-Neo (TOYOBO). Each PCR product was inserted into the NdeI site of pColdI using In-Fusion Advantage PCR cloning kit (Clontech Laboratories) to construct expression plasmid pColdI-bcABA4. This plasmid was introduced into E. coli BL21-Gold(DE3) for overexpression. The transformant was grown at 37°C at an OD600 of ~0.6 in 500 mL flask. After cooling at 4°C, isopropyl β-D-thiogalactopyranoside (0.1 mM) was added to the culture. After incubation at 16°C for 17 h, the cells were harvested by centrifugation at 4000 rpm. Harvested cells were resuspended in disruption buffer (100 mM Tris-HCl (pH 8.0), 20% glycerol, 1 mM DTT) and disrupted by sonication. After centrifugation, the supernatant was applied to a Ni-NTA column to purify the expressed protein.
Biotransformations
Mycelia of the transformant (AO-bcABA1, AO-bcABA2, or AO-bcABA124) were inoculated into 5 mL of MPY (maltose-peptone-yeast extract: 3% maltose, 1% polypeptone, 0.5% yeast extract) medium containing appropriate nutrients (0.925% (NH4)2SO4, 0,15% methionine, 0.01% adenine, 0.06% arginine) in 20 mL Erlenmeyer flask. After 2 d incubation at 30°C, substrate (100 μg, methanol solution) was then administered to the culture broth. After an additional 6 h incubation at 30°C, the mycelia were collected by filtration and soaked in acetone (10 mL). The organic layer was then concentrated in vacuo. The residual water was adjusted to pH 1.0 and then extracted with EtOAc, and the organic layers were concentrated in vacuo. The crude extracts were directly analyzed by a UPLC-MS apparatus according to method A.
Isolation of biosynthetic intermediates
α-ionylideneethane (2); Mycelia of AO-bcABA3 were inoculated into a solid medium containing polished rice (20 g) in 500 mL Erlenmeyer flasks. Each culture was incubated at 30°C for 7 d. After extraction with hexane, the organic layers were washed with brine, dried over anhydrous Na2SO4, and concentrated in vacuo. Purification of the residue by silica gel column chromatography (hexane) gave 2 (4.0 mg from 0.2 kg of rice medium). [α]D20 + 254.6° (c 0.50, CHCl3); FI-HR-MS: calcd. for C15H24 [M]+: 204.1878, found: 204.1884. The 1H-NMR data are in good agreement with the reported data [Citation9].
α-ionylideneacetic acid (3); The crude extracts of the biotransformation experiment of 2 (4.6 mg) using AO-bcABA1 were purified with silica gel column chromatography (hexane/ethyl acetate = 1/5) followed by further purification utilizing HPLC equipped with Wakosil-II 5C18 (ϕ4.6 mm x 250 mm) at the following conditions (λ = 210 nm, flow rate; 1.0 mL/min, solvent system; acetonitrile in H2O containing 0.1% of trifluoroacetic acid, 0–1 min: a linear gradient 90%-100%; 10–20 min: 100%) to afford 3 (1.9 mg). [α]D22 + 179.7° (c 0.16, EtOH). [lit. [α]D21 + 450.3° (c 0.6, EtOH)] [Citation16]. ESI-HR-MS: calcd. for C15H21O2 [M-H]−: 233.1547, found: 233.1549. The 1H-NMR data are in good agreement with the reported data [Citation14].
1ʹ-deoxyABA (6); In this case, fermentation period was changed to 2 d after administration of 3. The crude extracts were purified with silica gel column chromatography (hexane/ethyl acetate = 1/1) followed by further purification utilizing HPLC to give 6 (0.4 mg from 5.0 mg of 3). ESI-HR-MS: calcd. for C15H19O3 [M-H]−: 247.1340, found: 247.1343. The 1H-NMR data are in good agreement with the reported data [Citation17].
Results and discussion
Sequence and functional analyses of bcABA3
In the previous report [Citation12], we initially described the functional analysis of BcABA3 to simplify the discussion although we actually elucidated the function of BcABA124 first. It may be worthwhile to describe how we conducted the experiments for elucidating the function of totally new terpene synthase BcABA3. In the report describing the identification of the ABA gene cluster [Citation11], authors used DNA sequences from three strains of B. cinerea; ABA overproducer ATCC58025 (for gene inactivation), SAS56 (for making a genomic DNA library), and B05.10 (for checking the gene organization). Among these strains, the phytopathogenic strains SAS56 and B05.10 do not produce ABA in axenic culture [Citation18]. This paper did not provide any sequence data of bcABA3 from the three strains. We speculated that all three sequences may be nearly identical because they did not mention their differences.
In the beginning, we considered that slight differences in the bcABA gene sequences from three strains might affect the expression analysis. In addition, we knew many researchers involved in the study of fungal ABA biosynthesis focused on the terpene synthase [Citation19] and mostly failed. Therefore, before going to the detailed analysis of previously uncharacterized BcABA3, we decided to confirm the functions of BcABA124 because their biosynthetic intermediates were predicted experimentally [Citation9,Citation10] and bioconversions of these intermediates were easier to be employed. For functional analysis of bcABA genes, we used B. cinerea MAFF306914 strain, which is genetically relevant to strain B05.10 (99% nucleotide sequence identities with bcABA1-4) [Citation20]. As described later sections, all bcABA124 sequences from an ABA non-producer MAFF306914 (B05.10) are functional. With these experimental data, we are now confident to employ functional analysis of BcABA3.
At first, we focused on the initially deposited sequence bcABA3-S (accession number CAJ87067, 1254 bp) from SAS56 because that from ATCC58025 was not available. The nucleotide sequence of bcABA3-S was prepared by PCR-based removal of an extra base from the longer sequence bcABA3-L (BCIN_08g03880, 1323 bp). A recombinant His6-tagged BcABA3-S expressed in E. coli was obtained as an insoluble form. Solubility was improved when it was expressed as a fusion protein with maltose binding protein. However, no enzymatic activity was detected. Being different from BcABA3-S, His6-tagged BcABA3-L was expressed as a soluble form. In vitro, enzymatic reaction revealed that BcABA3-L mediated cyclization of FPP to yield 2 in the presence of Mg2+ [Citation12]. The nucleotide sequence of bcABA3-L is highly conserved in strains that did not produce 1, B05.10 and T4, suggesting that production of 1 does not depend on the nucleotide sequence of bcABA3, despite SAS56 strain harboring bcABA3-S. It should be emphasized that BcABA3 is a novel terpene synthase, which exhibits low amino acid sequence similarities with those of well-known sesquiterpene synthases, thus limiting the assessment whether the amino acid sequences predicted from the corresponding nucleotide sequences are functional or not.
Heterologous expression of bcABA genes in A. oryzae
Production of 2 by BcABA3 allowed us to speculate that remaining three modification enzymes, BcABA1, BcABA2, and BcABA4, participated in the transformation of 2 to 1. To determine whether the proposed genes are sufficient for the production of 1, we prepared three plasmids, pTAex3-bcABA1, pAdeA2-bcABA3, and pUSA2-bcABA24, which were used for transformations to yield AO-bcABA1234. Each DNA clone, bcABA1, bcABA2, and bcABA4, exhibited more than 99% nucleotide sequence identities with those of the corresponding gene from strains B05.10 and T4 (Figure S1 and ). Semi-synthetic intermediates, such as 2, 3, 5, and 7 [Citation9,Citation13,Citation14], and natural product 1 were used as chromatographic standards. Careful UPLC analysis of the metabolites from AO-bcABA1234 revealed the production of 1 (estimated yield based on the peak area of 1; 8 mg/L in MPY medium, 69 mg/kg of rice medium) as well as small amount of 1ʹ-deoxyABA (6). The structure was determined by NMR analysis described in the following section. Since 6 was not isolated from B. cinerea, it appeared to be a byproduct possibly produced by unexpected oxidation of 1ʹ-hydroxyionylideneacetic acid (4) (). On the other hand, putative biosynthetic intermediates such as 2, 3, 5, and 7 were not detected in the metabolites, suggesting that they might efficiently be converted into 1 in this transformant ().
Table 1. bcABA1-4 genes identified from B. cinerea.
Functional analysis of BcABA4
Previous gene inactivation experiment revealed that ΔbcABA4 accumulated one compound, the molecular weight of which is identical to that of 1ʹ,4ʹ-trans-dihydroxy-α-ionylideneacetic acid (5) [Citation10], indicating that BcABA4 mediates oxidation of 5 into 1 in the last step of ABA biosynthesis (). This proposed oxidation was confirmed by in vitro enzymatic reactions. The cDNA sequence of BcABA4, which was prepared from AO-bcABA1234, was cloned into the expression vector pColdI for protein expression in Escherichia coli BL21-Gold(DE3). The N-terminal His6-tagged protein was successfully expressed in a soluble form. The purified enzymes were subjected to SDS-PAGE analysis to confirm their molecular size and purity (). When the recombinant BcABA4 was incubated with racemic 5 (rac-5) in the presence of NAD+, 1 was produced as a sole product (). In contrast, no product was detected when using NADP+ instead of NAD+, suggesting that BcABA4 oxidizes rac-5 using NAD+. As 7, which is a diastereomeric compound of 5, was also converted into 1 in B. cinerea, rac-7 was used as a substrate instead of rac-5. UPLC analysis demonstrated that 1 was also produced by the action of BcABA4. Time course experiments showed preference of BcABA4 toward natural diastereomer 5 (4.1 mol/mg*min), although BcABA4 also accommodates 7 (0.87 mol/mg*min) in the active site cavity. The reverse reaction of 1 was not detected under the same reaction conditions. These results provided conclusive evidence that 5 is unambiguously a biosynthetic intermediate in B. cinerea.
Functional analysis of modification enzyme genes
The function of remaining P450s, BcABA1 and BcABA2, was then examined by biotransformation experiments using three AO-transformants, AO-bcABA1, AO-bcABA2, and AO-bcABA124. These transformants were prepared by introducing expression plasmids, pTAex3-bcABA1, pTAex3-bcABA2, and pUSA2-bcABA24, into the wild type strain, A. oryzae NSAR1.
Initially, racemic 2 (rac-2) was incubated with AO-bcABA124. UPLC analysis of the metabolites showed production of 1 as well as 6, confirming that biotransformation is effective to analyze the function of BcABA1 and BcABA2 (Figure S2). However, during the functional analysis of BcABA1, we found that AO-bcABA1 produced optically active 6 when incubated with rac-2. The CD spectrum was in good agreement with the reported data of (1ʹR)-(+)-6 [Citation21] (Figure S3), demonstrating that a stereochemistry of the C1ʹ side chain of 6 is the same as that of 1. This optical resolution of rac-2 occurs during the oxidative modifications catalyzed by BcABA1. However, to avoid complicated discussions caused by kinetic optical resolution, we just focused on determining the biosynthetic pathway. For this purpose, optically active 2 was used as a substrate for biotransformation experiments. It was isolated from AO-bcABA3, which produces 2 in solid medium (isolation yield: 20 mg/kg of rice). UPLC analysis of the metabolites revealed that AO-bcABA124 converted 2 into 1 and a small amount of 6 and that AO-bcABA1 converted 2 into two products, 3 and 6 (). On the other hand, both AO-WT and AO-bcABA2 yielded no product from 2 (). HR-MS analysis revealed the molecular formula of 6 as C15H20O3. The 1H-NMR spectrum was identical to that of 1ʹ-deoxyABA reported in the previous paper [Citation17]. Typical signals are as follows: four vinyl protons (δH 7.70, 6.10, 5.93, and 5.72), one methine proton (δH 2.83), two allylic methyl protons (δH 2.03 and 1.95), one methylene proton (δH 2.48 and 2.06), and two methyl protons (δH 1.06 and 1.00). Multiplicities at H5 (δH 6.10, dd, J = 15.8, 9.35 Hz) showed the presence of methine proton (δH 2.83, d, J = 9.35 Hz) at C1ʹ position. The degree of oxidation suggested that 6 is an oxidative product of 3, indicating that carboxylic acid formation on the terminal methyl group of 2 precedes the ketone formation leading to 6.
Figure 2. UPLC profiles. (a) authentic samples, and metabolites produced by (b) AO-WT with 2, (c) AO-bcABA1 with 2, (d) AO-bcABA2 with 2, (e) AO-bcABA124 with 2, (f) AO-WT with 3, (g) AO-bcABA1 with 3, (h) AO-bcABA2 with 3, and (i) AO-bcABA124 with 3.
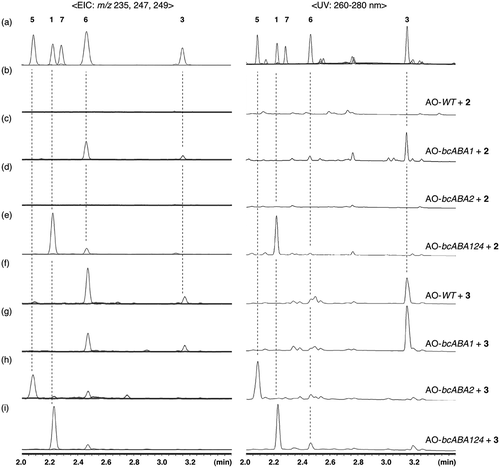
To further elucidate the late-stage oxidations, similar biotransformation experiments were conducted with optically active 3, which was prepared from large-scale biotransformation of 2 with AO-bcABA1. We identified 1 as a major product when 3 was incubated with AO-bcABA124, confirming that 3 is a biosynthetic intermediate (). Diol 5 was observed as a major product among the metabolites obtained from AO-bcABA2 whereas the diastereomeric compound 7 was not. This metabolite profile is consistent with that of B. cinerea. Accumulation of 6 was observed even during the biotransformation experiment of 3 using AO-WT, which indicated that allylic oxidation of 3 is catalyzed by inherent enzymes in A. oryzae and that 6 is a shunt product. This was further validated by the fact that no conversion of 6 was observed using AO-bcABA124 (Figure S4).
Biosynthetic pathway of abscisic acid in B. cinerea
The oxidative modifications of 2 leading to 1 in B. cinerea are summarized in . They can be divided into three steps: step 1) allylic oxidations of the terminal methyl group of 2 to give 3, step 2) allylic oxidations at C1ʹ and C4ʹ positions of 3 to yield 5, and step 3) an alcohol oxidation of 5 to furnish 1. The former two are catalyzed by the two cytochrome P450s, BcABA1 and BcABA2, respectively. Among them, BcABA2 catalyzing two rounds of allylic oxidations is remarkable in that the face selectivity is different during the first- and second-step oxidation (first step: β-face of C4ʹ, second step: α-face of C1ʹ). The intermediate 3 also undergoes allylic oxidations catalyzed by the action of inherent enzymes in A. oryzae. However, such unexpected oxidation could not affect the production of 1 when the downstream oxidation enzyme genes, bcABA2 and bcABA4, were co-expressed with bcABA1, because the rapid conversion of 3 into 5 suppressed the side reaction. Similar phenomena were reported in the reconstitution studies of pyripyropenes (side reaction: hydrolysis) [Citation22], paxilline (hydrolysis) [Citation23], and solanapyrone (oxidation) [Citation24]. Alcohol oxidation of 5 catalyzed by BcABA4 is also interesting in that BcABA4 accommodates diastereomeric compounds, 5 and 7, although the conversion rate of 5 is much higher than that of 7.
ABA biosynthesis in fungi
ABA was widely distributed in phytopathogenic fungi including Cercospora species [Citation6], Rhizopus species [Citation25], and Fusarium species [Citation25]. Labeling experiments using Cercospora species, such as C. rosicola [Citation26–Citation28], C. cruenta [Citation28,Citation29], and C. pini-densiflorae [Citation30], revealed that the biosynthetic pathway of 1 depends on the species, although all fungi commonly applied the C15 direct pathway. For example, in C. rosicola, 6 is considered as an intermediate instead of 5, suggesting that the order of oxidation from 2 to 1 is different from the biosynthetic pathway in B. cinerea. On the other hand, in C. cruenta, γ-ionylideneethane is likely a biosynthetic intermediate, although subsequent oxidation processes are identical to those in B. cinerea. These differences in the biosynthetic pathways can be explained by assuming that functionally relevant ABA proteins can be evolved by a minute change in the active site residues of BcABA proteins. Therefore, once the corresponding enzymes are found in the genomes of C. rosicola and C. cruenta, we can provide conclusive evidences on this hypothesis through well-established biotransformation experiments.
Author contribution
J.T., T.K., A.M., and H.O. designed research; J.T., and T.K. performed research; A.M., J.T., T.K., T.O., C.L., A.M., and H.O. analyzed data; and A.M. and H.O. wrote the paper.
19ABA_BBB_SI_20190425R_.docx
Download MS Word (4.6 MB)Disclosure statement
No potential conflict of interest was reported by the authors.
Additional information
Funding
References
- Nambara E, Marion-Poll A. Abscisic acid biosynthesis and catabolism. Annu Rev Plant Biol. 2005;56:165–185.
- Zocchi E, Basile G, Cerrano C, et al. ABA- and cADPR-mediated effects on respiration and filtration downstream of the temperature-signaling cascade in sponges. J Cell Sci. 2003;116:629–636.
- Magnone M, Bruzzone S, Guida L, et al. Abscisic acid released by human monocytes activates monocytes and vascular smooth muscle cell responses involved in atherogenesis. J Org Chem. 2009;284:17808–17818.
- Malara A, Fresia C, Di Buduo CA, et al. The plant hormone abscisic acid is a prosurvival factor in human and murine megakaryocytes. J Org Chem. 2017;292:3239–3251.
- Marumo S, Kitayama M, Komori E, et al. Microbial production of abscisic acid by Botrytis cinerea. Agric Biol Chem. 1982;46:1967–1968.
- Assante G, Merlini L, Nasini G. (+)-Abscisic acid, a metabolite of the fungus Cercospora rosicola. Experientia. 1977;33:1556–1557.
- Lievens L, Pollier J, Goossens A, et al. Abscisic acid as pathogen effector and immune regulator. Front Plant Sci. 2017;8:587.
- Oritani T, Kiyota H. Biosynthesis and metabolism of abscisic acid and related compounds. Nat Prod Rep. 2003;20:414–425.
- Inomata M, Hirai N, Yoshida R, et al. The biosynthetic pathway to abscisic acid via ionylideneethane in the fungus Botrytis cinerea. Phytochemistry. 2004;65:2667–2678.
- Hirai N, Okamoto M, Koshimizu K. The 1ʹ,4ʹ-trans-diol of abscisic acid, a possible precursor of abscisic acid in Botrytis cinerea. Phytochemistry. 1986;25:1865–1868.
- Siewers V, Kokkelink L, Smedsgaard J, et al. Identification of an abscisic acid gene cluster in the grey mold Botrytis cinerea. Appl Environ Microbiol. 2006;72:4619–4626.
- Takino J, Kozaki T, Sato Y, et al. Unveiling biosynthesis of the phytohormone abscisic acid in fungi: unprecedented mechanism of core scaffold formation catalyzed by an unusual sesquiterpene synthase. J Am Chem Soc. 2018;140:12392–12395.
- Norman SM, Poling SM, Maier VP, et al. Ionylideneacetic acids and abscisic acid biosynthesis by Cercospora rosicola. Agric Biol Chem. 1985;49:2317–2324.
- Hirai N, Yoshida R, Todoroki Y, et al. Biosynthesis of abscisic acid by the non-mevalonate pathway in plants, and by the mevalonate pathway in fungi. Biosci Biotechnol Biochem. 2000;64:1448–1458.
- Jin FJ, Maruyama J, Juvvadi PR, et al. Development of a novel quadruple auxotrophic host transformation system by argB gene disruption using adeA gene and exploiting adenine auxotrophy in Aspergillus oryzae. FEMS Microbiol Lett. 2004;239:79–85.
- Yamashita K, Nagano E, Oritani T. Optical resolution and biological activities of α-ionylideneacetic acid. Agric Biol Chem. 1980;44:1441–1442.
- Hirai N, Iwami K, Horiuchi M, et al. Electrolytic reduction of abscisic acid methyl ester and its free acid. Phytochemistry. 2012;80:89–98.
- Okamoto M, Hirai N, Koshimizu K. Biosynthesis of abscisic acid. Mem Coll Agric Kyoto Univ. 1988;132:79–115.
- Izquierdo-Bueno I, González-Rodríguez VE, Simon A, et al. Biosynthesis of abscisic acid in fungi: identification of a sesquiterpene cyclase as the key enzyme in Botrytis cinerea. Environ Microbiol. 2018;20:2469–2482.
- Staats M, van Jan JA. Genome update of Botrytis cinerea Strains B05.10 and T4. Eukaryot Cell. 2012;11:1413–1414.
- Todoroki Y Studies on highly potent analogues of abscisic acid [PhD thesis]. 1996. NDLBibID: 000000295512. doi:10.11501/3110611.
- Itoh T, Tokunaga K, Matsuda Y, et al. Reconstitution of a fungalmeroterpenoid biosynthesis reveals the involvement of a novelfamily of terpene cyclases. Nat Chem. 2010;2:858–864.
- Tagami K, Liu C, Minami A, et al. Reconstitution of biosynthetic machinery for indole-diterpene paxilline in Aspergillusoryzae. J Am Chem Soc. 2013;135:1260–1263.
- Fujii R, Ugai T, Ichinose H, et al. Reconstitution of biosynthetic machinery of fungal polyketides: unexpectedoxidations of biosynthetic intermediates by expression host. Biosci Biotechnol Biochem. 2016;80:426–431.
- Wu J, Shi Z. Isolation of abscisic acid producing strains of phytopathogens. Phytochemistry. 1998;49:89–90.
- Neill SJ, Horgan R, Walton DC, et al. The metabolism of α-ionylidene compounds by Cercospora rosicola. Phytochemistry. 1987;26:2515–2519.
- Bannett RD, Norman SM, Maier VP. Intermediate steps in the biosynthesis of abscisic acid from farnesyl pyrophosphate in Cercospora rosicola. Phytochemistry. 1990;29:3473–3477.
- Yamamoto H, Inomata M, Tsuchiya S, et al. Metabolism of chiral ionylideneacetic acids on the abscisic acid biosynthetic pathway in Cercospora. Biosci Biotechnol Biochem. 2000;64:2644–2650.
- Inomata M, Hirai N, Yoshida R, et al. Biosynthesis of abscisic acid by the direct pathway via ionylideneethane in a fungus, Cercospora cruenta. Biosci Biotechnol Biochem. 2004;68:2571–2580.
- Okamoto M, Hirai N. Biosynthesis of abscisic acid from α-ionylideneethanol in Cercospora pini-densiflorae. Phytochemistry. 1988;27:3465–3469.