ABSTRACT
Depressive disorders are partly caused by chronic inflammation through the kynurenine (KYN) pathway. Preventive intervention using anti-inflammatory reagents may be beneficial for alleviating the risk of depression. In this study, we focused on the Japanese local citrus plant, Citrus tumida hort. ex Tanaka (C. tumida; CT), which contains flavonoids such as hesperidin that have anti-inflammatory actions. The dietary intake of 5% immature peels of CT fruits slightly increased stress resilience in a subchronic and mild social defeat (sCSDS) model in mice. Moreover, the dietary intake of 0.1% hesperidin significantly increased stress resilience and suppressed KYN levels in the hippocampus and prefrontal cortex in these mice. In addition, KYN levels in the hippocampus and prefrontal cortex were significantly correlated with the susceptibility to stress. In conclusion, these results suggest that dietary hesperidin increases stress resilience by suppressing the augmentation of KYN signaling under sCSDS.
Graphical abstract

Dietary intake of hesperidin increased stress resilience in the subchronic and social defeat stress model of mice.
Depression is a common illness worldwide, affecting more than 300 million people [Citation1]. In the near future, the number of depression patients will increase not only in developed countries but also in developing countries [Citation2]. Therapeutic drugs such as anti-depressants reduce several deleterious symptoms of depression; however, most have severe side effects [Citation3]. Furthermore, some patients with depression do not show significant improvement in response to any medical treatment. As such, alternative therapeutic approaches are warranted [Citation4].
Previously, we discovered that the purity of the diet affects social behaviors in mice subjected to subchronic and mild social defeat stress (sCSDS). We demonstrated that several ingredients in non-purified commercial diets containing various natural food materials may increase stress resilience in sCSDS mice [Citation5,Citation6]. In addition, some researchers have focused on alternative medicines including functional foods [Citation7] and traditional Chinese medicine (Kampo) [Citation8] for the prevention and treatment of depression. Administration of Kampo medicines containing the peel of Citrus unshiu suppressed social behavioral deficits in mice under chronic social defeat stress [Citation8]. Furthermore, glucoraphanin, a precursor of sulforaphane found in broccoli sprouts, increased stress resilience in mice exposed to repeated social defeat stress [Citation9]. Taken together, these observations suggest that several ingredients of foods and farm products affect sensitivity to psychosocial stress.
Inflammation in the central nervous system is a critical pathogenic mechanism underlying depression [Citation10]. Several inflammatory molecules are implicated in depression, such as interleukin-6 (IL-6), which is critically involved in depression pathogenesis [Citation10]. There has been a recent focus on the relationship between the kynurenine (KYN) pathway and depression [Citation11]. KYN is one of the downstream metabolites of the tryptophan (Trp) pathway [Citation12], and has neurotoxic, excitotoxic, cytotoxic, and neuroinflammatory effects [Citation13].
One of our goals was to identify foods and/or dietary ingredients with anti-inflammatory properties that may be developed as preventive therapeutics for depression, and thus identify functional foods with a potential for enhancing stress resilience. We initially focused on a local citrus plant, Citrus tumida hort. ex Tanaka (C. tumida; CT), cultivated around Mt. Tsukuba in Ibaraki Prefecture, Japan [Citation14]. In our recent studies, dietary supplementation with immature peels of CT was found to suppress body weight gain and fat accumulation in an acute mouse model of obesity [Citation15]. CT contains many flavonoids with potential health benefits [Citation16]. In particular, the immature peel of CT has abundant flavonoids such as hesperidin, nobiletin, and tangeretin, which demonstrate anti-inflammatory actions [Citation16]. Therefore, in our first experiment, we examined whether the dietary intake of immature peels of CT affected resilience to psychosocial stress in sCSDS mice. Next, we focused on the flavonoids contained in the immature peels of CT. Among them, hesperidin is a citrus flavonoid [Citation17] with many health benefits including anti-inflammatory, anti-oxidant, anti-obesity, anti-anxiety, and neuroprotective effects [Citation18]. Furthermore, hesperidin showed antidepressant-like actions in an acute depression model in rodents exposed to forced swim and tail suspension [Citation19–Citation22]. Therefore, in our second experiment, we examined whether the dietary intake of hesperidin affected resilience to psychosocial stress in sCSDS mice. In addition, we analyzed IL-6, IL-1β, and corticosterone levels in the blood and KYN levels in the blood and brain in these animals.
Materials and methods
Experiment 1
Animals
All procedures of animal experiments were reviewed and approved by the Animal Care and Use Committee of Ibaraki University (Ibaraki, Japan), and the guidelines conformed to those put forth by the Ministry of Education, Culture, Sports, Science and Technology, Japan (Notification, No. 71).
Animal experiments were performed as previously reported [Citation23]. This sCSDS model can only be induced in male mice [Citation23,Citation24]; hence, only male mice were tested in this study. Male C57BL/6JJmsSlc (B6) mice (7 weeks of age; weighing 21–23 g; intruders) and male Slc:ICR (ICR) mice (retired, older than 6 months of age; residents) were purchased from SLC Japan (Shizuoka, Japan). Food and water were available ad libitum. Animals were maintained at constant temperature (23 ± 1°C) and under a 12-hour light-dark cycle (lights on at 7:00 am). For acclimation, all mice were housed individually in the above conditions for 1 week.
Preparation of immature CT peel powder
Immature CT was harvested at an orchard in the southwestern foothill of Mt. Tsukuba, Ibaraki prefecture, Japan, in October 2015. Peels containing both orange flavedo and white albedo were manually collected and freeze-dried using a freeze-dryer (FDU-1110, TOKYO RIKAKIKAI, Tokyo, Japan). The dried peels were powdered by a centrifugal mill (ZM-1, Retsch Technology GmbH, Haan, Germany). Peel powder was stored in a drying shelf at room temperature (23–26°C) until use. The nutritional components of immature CT peel powder were analyzed by the Japan Food Research Laboratories (Tokyo, Japan) (Supplementary Table 1).
Experimental design
The experimental design is shown in . To elucidate whether the dietary intake of immature CT peel increased resilience in sCSDS mice, B6 mice (n = 54) were divided into two body weight-matched groups that received either semi-purified diet (AIN-93G diet, Oriental Yeast, Tokyo, Japan; ) (n = 27) or AIN-93G containing 5% (w/w) immature CT peel powder (n = 27). All mice were initially fed these diets for 3 weeks before sCSDS exposure. After the sCSDS paradigm, all mice were subjected to the social interaction (SI) test as previously reported [Citation23]. In this study, we defined these two groups as “sCSDS” and “sCSDS + CT”, respectively.
Table 1. Dietary ingredients and food composition list of semi-purified diet (AIN-93G).
sCSDS model
An sCSDS paradigm [Citation23] based on the standard protocol for chronic social defeat in mice [Citation24] was performed from 9:00 am to 11:00 am. Briefly, the duration of physical contact was set at 5 min after the first attack bite on day 1, and was then reduced by 0.5 min per day from days 2 to 10. From days 1 to 10, subject mice (B6) were exposed to a different ICR aggressor mouse each day. After physical contact, test mice were moved into the neighboring compartment with another aggressor for 24 h in a two-compartment social defeat (SD) cage (220 mm × 320 mm × 135 mm; Natsume Seisakusho, Tokyo, Japan).
Body weight, food intake, and water intake
Body weight and food and water consumption were measured daily. Body weight gain was calculated by subtracting the original body weight from the body weight of relevant the day. Food and water intake was calculated by subtracting the weights of food and water from weights of those on the previous day, respectively.
Social interaction (SI) test
The SI test was performed on Day 11 as described previously [Citation23]. SI scores (ICR mouse presence/ICR mouse absent) were calculated as 100 × (interaction time, ICR mouse present)/(interaction time, ICR mouse absent) [Citation25].
Statistical analysis
Statistical analysis for body weight gain, and food and water intakes were performed using Student’s t-tests. The SI score was tested using unpaired two-tailed Student’s t-tests. Data were analyzed with Excel Toukei 2006 for Windows (Social Survey Research Information Co. Ltd., Tokyo, Japan). To detect differences in resilience rates between groups of mice fed different diets, a 2 × 2 contingency table with Fisher’s exact test was generated using R software (www.R-project.org). The statistical significance threshold was set at p = 0.05.
Experiment 2
Animals
Details on the animals are as described in section Experiment 1.
Experimental design
The experimental design is shown in . To elucidate whether the dietary intake of hesperidin increased resilience in sCSDS mice, B6 mice (n = 40) were divided into three body-weight-matched groups: control group fed AIN-93G without sCSDS (n = 8), sCSDS group fed AIN-93G and subjected to sCSDS (n = 15), and sCSDS + Hes group fed AIN-93G containing 0.1% (100 mg/kg) hesperidin (Sigma, MO, USA) (w/w) and subjected to sCSDS (n = 17). Mice were initially fed the respective diets for 3 weeks before sCSDS. The sCSDS paradigm and SI test were performed as described previously [Citation23].
IL-6, IL-1β, and corticosterone measurements
At the end of experimentation, mice were fasted for 3 hours and then anesthetized with 4.5% isoflurane; following this, anesthesia was maintained with 3% isoflurane before blood samples were collected in tubes via the inferior vena cava. Plasma was separated from blood by centrifugation at 1,000 x g for 5 min at 4°C for biochemical assays. The levels of plasma IL-6 and IL-1β were measured using an ELISA kit (Enzo Life Sciences, Inc, NY, USA) according to the manufacturer’s protocol. Plasma corticosterone levels were measured using an EIA kit (Yanaihara Institute Inc, Shizuoka, Japan) according to the manufacturer’s protocol. The absorbance in the ELISA and EIA was measured using a microplate reader (FlexStation 3, Molecular Devices, LLC, Sunnyvale, USA).
KYN and trp measurements
Brain tissues were homogenized at an approximately 1:5 wet weight-to-volume ratio in 0.1 mol/L HCl. A 100 μL aliquot of the homogenate was acidified with 20 μL of 30% trichloroacetic acid. After centrifugation (15 min, 15,000 x g, 4°C), an aliquot of the supernatant was used for high-performance liquid chromatography (HPLC) examination of KYN and Trp. To determine plasma KYN levels, 100-μL of plasma was acidified with 20 μL of 30% trichloroacetic acid. After centrifugation (15 min, 15,000 x g, 4°C), an aliquot of the supernatant was used for the evaluation of KYN and Trp levels. The concentration of KYN in plasma was identified by HPLC using diode array detection (SPD-M20A, Shimadzu) at a wavelength of 365 nm. Free Trp in the brain and plasma was evaluated by HPLC using diode array detection (SPD-M20A, Shimadzu) at a wavelength of 280 nm.
Statistical analysis
Statistical testing for all data was performed using one-way analysis of variance (ANOVA) followed by Bonferroni’s post-hoc test. Data were analyzed with Excel Toukei 2006 for Windows (Social Survey Research Information Co. Ltd., Tokyo, Japan). To detect differences in resilience rates between groups of mice fed different diets, a 2 × 2 contingency table with Fisher’s exact test was generated using R software (www.R-project.org). The statistical significance threshold was set at p = 0.05.
Results
Experiment 1
Body weight gain, food intake, and water intake
The body weight gain of the sCSDS + CT group tended to be lower than that of the sCSDS group, although the difference was not statistically significant (sCSDS, 5.5 ± 0.3 g vs. sCSDS + CT, 4.9 ± 0.3 g; p = 0.0687). There was no significant difference in the total food intake (sCSDS, 104.0 ± 2.6 g vs. sCSDS + CT, 104.4 ± 2.4 g; p = 0.4551) and total water intake between the two groups (sCSDS, 149.2 ± 5.4 g vs. sCSDS + CT, 151.5 ± 6.1 g; p = 0.3875).
SI test
We conducted the SI test to evaluate whether dietary supplementation with CT affected stress resilience in sCSDS mice. SI scores and their distributions are plotted in . The SI score of the sCSDS + CT group tended to be higher than that of the sCSDS group, although the difference was not statistically significant (sCSDS, 72.7 ± 9.2% vs. sCSDS + CT, 96.1 ± 14.2%; p = 0.0867). The sCSDS group consisted of 21 stress-susceptible and 6 stress-resilient mice (resilience proportion = 22.2%; ), whereas the sCSDS + CT group consisted of 16 susceptible and 11 resilient mice (resilience proportion = 40.7%; ). There was no significant difference in the proportion of resilient mice between the two groups (p = 0.2409). Moreover, we analyzed the time spent in the interaction zone and the corner zone during SI tests (). There was no significant difference in the time spent in the interaction zone (s) with an ICR mouse (p = 0.1460), time spent in the corner zone with an ICR mouse (p = 0.6775), total distance travelled with an ICR mouse (p = 0.7860), time spent in the interaction zone (s) without an ICR mouse (p = 0.4832), time spent in the corner zone without an ICR mouse (p = 0.2387), or total distance travelled without an ICR mouse (p = 0.1441).
Table 2. Summary of number. of susceptible and resilient mice and resilient rate in each group.
Table 3. Social interaction test.
Experiment 2
Body weight gain, food intake, and water intake
There was no significant difference in body weight gain among groups (F2, 39 = 0.174, p = 0.8410; Control, 5.7 ± 0.4 g vs. sCSDS, 5.7 ± 0.4 g, p = 1.0000; Control vs. sCSDS ± Hes, 5.9 ± 0.3 g, p = 1.0000; sCSDS vs. sCSDS + Hes, p = 1.0000). However, there was a significant difference in the total food intake, with the sCSDS group showing significantly lower total food intake than the control and sCSDS + Hes groups (F2, 39 = 7.848, p = 0.0014; Control, 122.3 ± 2.5 g vs. sCSDS, 107.4 ± 2.9 g, p = 0.0096; Control vs. sCSDS + Hes, 120.9 + 2.9 g, p = 1.0000; sCSDS vs. sCSDS + Hes, p = 0.0035). There was also a significant difference in the total water intake, with the sCSDS and sCSDS + Hes groups showing significantly greater total water intake than the control group (F2, 39 = 20.090, p < 0.0001; Control, 104.9 ± 6.1 g vs. sCSDS, 164.7 ± 5.2 g, p < 0.0001; Control vs. sCSDS + Hes 158.4 ± 6.4 g, p < 0.0001; sCSDS vs. sCSDS + Hes, p = 1.0000).
SI test
To evaluate the effects of dietary supplementation with hesperidin on social avoidance behavior in sCSDS mice, SI scores and their distributions were plotted (). There was a significant difference in the SI score (F2, 39 = 8.636, p = 0.0008; Control, 124.8 ± 13.0% vs. sCSDS, 82.5 ± 10.2%, p = 0.0817; Control vs. sCSDS + Hes 143.7 ± 11.2%, p = 0.8996; sCSDS vs. sCSDS + Hes, p = 0.0006). Although the score in sCSDS group was lower than that in the control group, the difference was not statistically significant. However, the score in the sCSDS + Hes group was significantly higher than that in the sCSDS group.
Figure 3. Social interaction scores in the control (n = 8), sCSDS (n = 15), and sCSDS + Hes (n = 17) groups. The bar represents the mean ± SEM. Individual values are represented by circles. p values are for Bonferroni’s post-hoc test. †p < 0.1, ***p < 0.001. sCSDS, subchronic and mild social defeat stress.
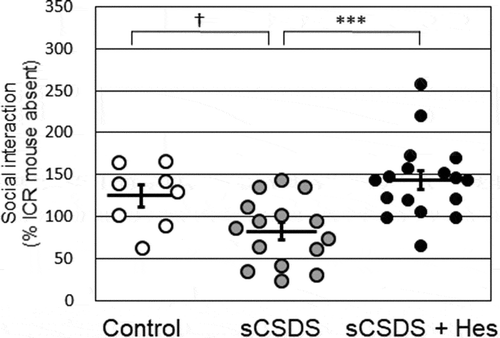
The control group consisted of 2 stress-susceptible and 6 stress-resilient mice (resilience proportion = 75.0%; ), whereas the sCSDS group consisted of 10 stress-susceptible and 5 resilient mice (resilience proportion = 33.3%; ) and the sCSDS + Hes group consisted of 3 stress-susceptible and 14 stress-resilient mice (resilience proportion = 82.4%; ). There was a significant difference in the proportion of resilient mice between the sCSDS and sCSDS + Hes groups (p = 0.0145), and the proportion of resilient mice in the control group tended to differ from that in the sCSDS group (p = 0.0894). However, there was no significant difference in the proportion of resilient mice between the control and sCSDS + Hes groups (p = 1.0000).
Table 4. Summary of number. of susceptible and resilient mice and resilient rate in each group.
To evaluate social behaviors, we analyzed the time spent in the interaction zone and the corner zone during SI tests (). There was a significant difference in the time spent in the interaction zone (s) with an ICR mouse, with the value being higher in the sCSDS + Hes group than in the sCSDS group (F2, 39 = 9.677, p = 0.0004; Control vs. sCSDS, p = 0.2719; Control vs. sCSDS + Hes, p = 0.2134; sCSDS vs. sCSDS + Hes, p = 0.0003). There was no significant difference in the time spent in the corner zone with an ICR mouse (F2, 39 = 1.705, p = 0.1958; Control vs. sCSDS, p = 0.6465; Control vs. sCSDS + Hes, p = 1.0000; sCSDS vs. sCSDS + Hes, p = 0.2599), total distance travelled with an ICR mouse (F2, 39 = 2.225, p = 0.1223; Control vs. sCSDS, p = 0.1994; Control vs. sCSDS + Hes, p = 1.0000; sCSDS vs. sCSDS + Hes, p = 0.3178), time spent in the interaction zone (s) without an ICR mouse (F2, 39 = 0.850, p = 0.4356; Control vs. sCSDS, p = 1.0000; Control vs. sCSDS + Hes, p = 0.6063; sCSDS vs. sCSDS + Hes, p = 1.0000), time spent in the corner zone without an ICR mouse (F2, 39 = 1.953, p = 0.1561; Control vs. sCSDS, p = 0.1716; Control vs. sCSDS + Hes, p = 0.7918; sCSDS vs. sCSDS + Hes, p = 0.8976), or total distance travelled without an ICR mouse (F2, 39 = 0.023, p = 1.0000; Control vs. sCSDS, p = 1.0000; Control vs. sCSDS + Hes, p = 1.0000; sCSDS vs. sCSDS + Hes, p = 1.0000).
Table 5. Social interaction test.
IL-6, IL-1β, and corticosterone levels in blood plasma
IL-6, IL-1β, and corticosterone levels in blood plasma are shown in . There was a significant difference in IL-6 levels (F2, 39 = 3.435, p = 0.0499; Control vs. sCSDS, p = 0.1114; Control vs. sCSDS + Hes, p = 0.0824; sCSDS vs. sCSDS + Hes, p = 1.0000). Although the levels in the sCSDS + Hes group tended to be higher than those in the control group, the difference was not statistically significant. IL-6 levels in plasma did not show a correlation with SI scores (r = −0.09, p = 0.3394). There was no significant difference in IL-1β levels (F2, 39 = 0.017, p = 0.9831; Control vs. sCSDS, p = 1.0000; Control vs. sCSDS + Hes, p = 1.0000; sCSDS vs. sCSDS + Hes, p = 1.0000). Differences in corticosterone levels were close to being statistically significant in the between-group analysis (F2, 39 = 2.783, p = 0.0837; Control vs. sCSDS, p = 1.0000; Control vs. sCSDS + Hes, p = 0.1135; sCSDS vs. sCSDS + Hes, p = 0.2723). IL-1β and corticosterone levels in plasma did not show correlations with SI scores (IL-1β, r = −0.12, p = 0.2883; corticosterone, r = −0.25, p = 0.1128).
Table 6. Interleukin-6, interleukin-1β, and corticosterone levels in plasma.
KYN, trp, and the ratio of KYN to trp (KYN/trp) levels in the brain and blood plasma
KYN and Trp levels, and the KYN/Trp ratio in the brain and blood plasma, are shown in and . There was a significant difference in KYN levels in the hippocampus (F2, 39 = 13.025, p < 0.0001; Control vs. sCSDS, p = 0.0009; Control vs. sCSDS + Hes, p = 1.0000; sCSDS vs. sCSDS + Hes, p < 0.0001), with the levels in the sCSDS group being significantly higher than those in the control group. The KYN levels were significantly suppressed in the sCSDS + Hes group compared with the sCSDS group. Hippocampal KYN levels showed a negative correlation with SI scores (r = −0.29, p = 0.0346). There was a significant difference in Trp levels in the hippocampus (F2, 39 = 5.934, p = 0.0058; Control vs. sCSDS, p = 0.0331; Control vs. sCSDS + Hes, p = 1.0000; sCSDS vs. sCSDS + Hes, p = 0.0108; ), with the levels in the sCSDS group being significantly higher than those in the control group. The Trp levels were significantly suppressed in the sCSDS + Hes group than in the sCSDS group. Hippocampal Trp levels did not show any correlation with SI scores (r = −0.22, p = 0.0851). There was a significant difference in KYN/Trp levels in the hippocampus (F2, 39 = 3.818, p = 0.0311; Control vs. sCSDS, p = 0.0937; Control vs. sCSDS + Hes, p = 1.0000; sCSDS vs. sCSDS + Hes, p = 0.0610; ). Although the levels in the sCSDS group tended to be higher than those in the control and sCSDS + Hes groups, the differences were not statistically significant. Hippocampal KYN/Trp levels did not show any correlation with SI scores (r = −0.18, p = 0.1311).
Table 7. Kynurenine and tryptophan levels in brain.
Table 8. Kynurenine and tryptophan levels in blood plasma.
There was a significant difference in KYN levels in the prefrontal cortex (F2, 39 = 11.528, p < 0.0001; Control vs. sCSDS, p = 0.0054; Control vs. sCSDS + Hes, p = 1.0000; sCSDS vs. sCSDS + Hes, p = 0.0002; ), with the levels in the sCSDS group being significantly higher than those in the control group. The KYN levels were significantly suppressed in the sCSDS + Hes group compared with the sCSDS group. KYN levels in the prefrontal cortex showed a negative correlation with SI scores (r = −0.33, p = 0.0178). There was no significant difference in Trp levels in the prefrontal cortex (F2, 39 = 0.646, p = 0.5299; Control vs. sCSDS, p = 1.0000; Control vs. sCSDS + Hes, p = 1.0000; sCSDS vs. sCSDS + Hes, p = 0.9819; ). Trp levels in the prefrontal cortex did not show any correlation with SI scores (r = 0.04, p = 0.3989). There was a significant difference in KYN/Trp levels in the prefrontal cortex (F2, 39 = 13.052, p < 0.0001; Control vs. sCSDS, p = 0.0030; Control vs. sCSDS + Hes, p = 1.0000; sCSDS vs. sCSDS + Hes, p < 0.0001; ), with the levels in the sCSDS group being significantly higher than those in the control group. The KYN/Trp levels were significantly suppressed in the sCSDS + Hes group compared with the sCSDS group. KYN/Trp levels in the prefrontal cortex showed a negative correlation with SI scores (r = −0.30, p = 0.0283).
There was no significant difference in KYN levels in plasma (F2, 39 = 2.186, p = 0.1266; Control vs. sCSDS, p = 1.0000; Control vs. sCSDS + Hes, p = 0.6883; sCSDS vs. sCSDS + Hes, p = 0.1463; ). KYN levels in plasma did not show any correlation with SI scores (r = −0.14, p = 0.1967). There was no significant difference in Trp levels in plasma (F2, 39 = 0.132, p = 0.8767; Control vs. sCSDS, p = 1.0000; Control vs. sCSDS + Hes, p = 1.0000; sCSDS vs. sCSDS + Hes, p = 1.0000; ). Trp levels in plasma did not show any correlation with SI scores (r = 0.26, p = 0.0555). There was no significant difference in KYN/Trp levels in plasma (F2, 39 = 1.947, p = 0.1571; Control vs. sCSDS, p = 1.0000; Control vs. sCSDS + Hes, p = 1.0000; sCSDS vs. sCSDS + Hes, p = 0.1725; ). KYN/Trp levels in plasma showed a negative correlation with SI scores (r = −0.30, p = 0.0302).
Discussion
Experiment 1 showed that dietary supplementation with immature peels of CT tended to suppress body weight gain without altering food intake. Similarly, Citrus unshiu peel extract [Citation26], and Shiikuwasa (Citrus depressa Hayata) peel extract [Citation27] suppressed body weight, body fat, blood triglycerides (TGs), and total cholesterol in mouse models of high fat diet-induced obesity. Human studies showed that subjects with a chronic dietary intake of grapefruit [Citation28] and those with a chronic intake of orange juice [Citation29] also showed reduced abdominal girth and blood TG levels. A previous study reported that citrus flavonoids influenced the activity of the AMP-activated protein kinase (AMPK) signalling pathway [Citation30]. Naringin, a major citrus flavonoid, inhibited body weight gain and body fat accumulation via the activation of AMPK and inactivation of acetyl-CoA carboxylase (ACC) in the liver [Citation31]. Furthermore, Shiikuwasa extract (mainly comprising hesperidin, nobiletin, and tangeretin) suppressed the expression of genes encoding fatty acid synthase and ACC in liver, epididymal, and perirenal fat adipocytes [Citation27].
Dietary supplementation with immature peels of CT tended to decrease social avoidance in sCSDS mice (, ). Previously, Citrus aurantium peel extract was reported to suppress anxiety-like behavior via the serotonergic system in the light-dark box test [Citation32]. Citrus flavonoids have anti-inflammatory, antioxidant, and anti-hyperglycemic effects [Citation17]. Several studies reported that hesperidin suppressed neural inflammation in rodents [Citation33,Citation34]. In addition, hesperidin could cross the blood brain barrier (BBB) in an in vitro BBB model co-cultured with ECV304 cells and C6 glioma cells [Citation35]. Hesperetin, the aglycon component of hesperidin [Citation36], is produced by bacterial hydrolysis of the sugar moiety of hesperidin in the digestive tract [Citation18]. In a human feces suspension, 34–58% of hesperidin was hydrolyzed to hesperetin [Citation36]. Hesperetin was also able to cross the BBB in vitro [Citation35]. Dietary hesperidin is digested to hesperetin, which is translocated to the brain [Citation37]. Furthermore, hesperetin has anti-inflammatory [Citation38] and neuroprotective effects [Citation39]. Although the precise amount of hesperidin in the immature peels of CT was unclear, the previous report described that the fresh and immature peel of Citrus depressa Hayata (Shiikuwasa) contains 0.4% hesperidin [Citation40]. The CT diet contained 5% of dried peel and might include about 0.2% of hesperidin (data not shown); however the 0.1% hesperidin diet had more effective for induction of stress resilience in mice. This discrepancy between CT diet and hesperidin diet may be occurred by the different efficiency of flavonoids absorption in the digestive tract. In future, the bioavailability of hesperidin in the CT should be studied.
Therefore, in Experiment 2, we examined the effects of dietary hesperidin on stress resilience and inflammatory markers in sCSDS mice. Supplementation with hesperidin increased the SI score in sCSDS mice (, ). Dietary hesperidin may therefore have the potential to increase resilience to sCSDS. Dietary supplementation with malvidin-3ʹ-O-glucoside contained in grape juice and seed extract increased stress resilience following the inhibition of social defeat stress-induced expression of IL-6 [Citation41]. In addition, dietary supplementation with sulforaphane increased stress resilience via the Nrf2-induced anti-inflammatory pathway [Citation9]. Suppressing brain inflammation may be effective for preventing depression [Citation42], given that IL-6 levels in the blood and brain of depression patients are higher than those of healthy people [Citation43]. Further, a study found that inhibiting peripheral IL-6 induced stress resilience in mice [Citation10]. However, dietary hesperidin failed to decrease plasma IL-6 levels in sCSDS mice; therefore, dietary hesperidin may affect other critical targets that endow mice with resilience to social defeat and are linked to inflammation (). In addition, blood corticosterone levels were not changed by sCSDS. A previous report described that chronic social defeat stress (CSDS) increased blood corticosterone levels in both resilient and susceptible mice [Citation25]. In contrast, another study reported that CSDS did not alter corticosterone levels [Citation44]. Such contradictions may be due to sampling conditions, as blood corticosterone levels are profoundly affected by sampling timing after stress exposure [Citation45].
Next, we focused on the KYN pathway, which is linked to both inflammation and psychiatric disorders [Citation46]. sCSDS increased KYN levels in the hippocampus and prefrontal cortex (). Dietary hesperidin suppressed KYN levels in the hippocampus and prefrontal cortex of sCSDS mice. In addition, we discovered a significant negative correlation between brain KYN levels and SI scores (). Fuerting et al. reported that social defeat stress increased KYN levels in the brain and blood of mice [Citation47]. In our study, sCSDS did not increase blood KYN levels (). This discrepancy may be dependent on the differences of stress paradigms and experimental environments. Furthermore, inhibition of indoleamine 2,3-dioxygenase (IDO 1) suppressed fear behavior in mice exposed to social defeat stress [Citation47]. Similarly, Laumet et al. reported that inhibiting KYN 3-monooxygenase had an anti-depressant-like effect in the spared nerve injury mouse model [Citation11]. In humans, suicide attempters with major depressive disorder had higher levels of blood KYN than did healthy people [Citation48]. In addition, a recent metabolomic study showed that blood KYN levels in depression patients were higher than those in healthy people [Citation49], while Trp and KYN levels in the blood of depression patients were lower than those in healthy people [Citation50]. Furthermore, it was reported that there was a negative correlation between suicidal ideation and blood KYN levels in depression patients [Citation51]. In general, blood Trp levels are profoundly affected by food intake and diet composition, and the methods used to analyze Trp and KYN levels may significantly influence the outcomes. In our study, which involved sCSDS mice, dietary hesperidin increased stress resilience and suppressed brain KYN levels. Future studies should examine whether dietary hesperidin lowers brain KYN and increases resilience. Further, in other rodent models of depression such as forced-swim and tail-suspension models, peripheral injections of hesperidin exerted anti-depressant like actions [Citation19–Citation21]. These hesperidin-induced antidepressant-like actions involved kappa opioid receptor signalling [Citation19], 5-HT1A receptor signalling [Citation20], and the nitric oxide (NO)- cyclic guanosine monophosphate (cGMP) pathway [Citation21]. NO may potentiate KYN metabolism through the activation of microglia and induction of inflammatory cytokines [Citation52]. Therefore, it may be worth focusing on NO-cGMP and KYN pathways to investigate the anti-depressant effects of hesperidin.
A critical limitation of this study is that the preventive effects of hesperidin on depression-like symptoms were only assessed in male mice exposed to social defeat because it is difficult to develop female mice models of social defeat and this model is unpopular. In the future, we will investigate the detailed mechanisms of the preventive functions of hesperidin on depression using depression models in both sexes.
To conclude, this study suggested that dietary supplementation with hesperidin modified the brain KYN pathway and reduced the risk of social deficits in sCSDS mice. In the future, the mechanisms underlying the action of hesperidin and/or hesperetin on stress resilience and the KYN pathway should be assessed.
Author contributions
A. Toyoda and M. Sato designed the studies. M. Sato, A. Okuno, K. Suzuki, N. Ohsawa, E. Inoue, and Y. Miyaguchi performed the experiments. A. Toyoda and M. Sato analyzed the data. A. Toyoda and M. Sato wrote the manuscript.
MS2019.04.17_Supplementary_Table.pdf
Download PDF (40.5 KB)Acknowledgments
We thank H. Shimonishi (Ibaraki University) for valuable technical assistance and Drs. S. Tomonaga (Kyoto University) and T. Goto (Obihro University of Agriculture and Veterinary Medicine) for valuable comments regarding the manuscript. This research was supported in part by Ibaraki University Cooperation between Agriculture and Medical Science (IUCAM) (The MEXT, Japan), Prioritised Research (Ibaraki University, Japan), and the Council for Science, Technology and Innovation (CSTI) under the Cross-ministerial Strategic Innovation Promotion Program (SIP) “Technologies for creating next-generation agriculture, forestry, and fisheries” (Bio-oriented Technology Research Advancement Institution, NARO) (The Cabinet Office, Japan). We would like to thank Editage (www.editage.jp) for English language editing.
Disclosure statement
No potential conflict of interest was reported by the authors.
Supplemental material
Supplemental data for this article can be accessed here.
Additional information
Funding
References
- Depression [Internet]. World Health Organization (WHO) [cited 2019 Feb 7]. Available from: http://www.who.int/mediacentre/factsheets/fs369/en/
- Ferrari AJ, Charlson FJ, Norman RE, et al. Burden of depressive disorders by country, sex, age, and year: findings from the global burden of disease study 2010. PLoS Med. 2013;10:e1001547.
- Stevens JR, Jarrahzadeh T, Brendel RW, et al. Strategies for the prescription of psychotropic drugs with black box warnings. Psychosomatics. 2014;55:123–133.
- El-Hage W, Leman S, Camus V, et al. Mechanisms of antidepressant resistance. Front Pharmacol. 2013;22:146.
- Goto T, Kubota Y, Toyoda A. Effects of diet quality on vulnerability to mild subchronic social defeat stress in mice. Nutr Neurosci. 2016;19:284–289.
- Goto T, Tomonaga S, Toyoda A. Effect of diet quality and psychosocial stress on the metabolic profiles of mice. J Proteome Res. 2017;16:1857–1867.
- Arai S. Studies on functional foods in Japan–state of the art. Biosci Biotechnol Biochem. 1996;60:9–15.
- Ito N, Hirose E, Ishida T, et al. Kososan, a kampo medicine, prevents a social avoidance behavior and attenuates neuroinflammation in socially socially defeated mice. J Neuroinflammation. 2017;14:98.
- Yao W, Zhang JC, Ishima T, et al. Role of Keap1-Nrf2 signaling in depression and dietary intake of glucoraphanin confers stress resilience in mice. Sci Rep. 2016;6:30659.
- Hodes GE, Pfau ML, Leboeuf M, et al. Individual differences in the peripheral immune system promote resilience versus susceptibility to social stress. Proc Natl Acad Sci U S A. 2014;111:16136–16141.
- Laumet G, Zhou W, Dantzer R, et al. Upregulation of neuronal kynurenine 3-monooxygenase mediates depression-like behavior in a mouse model of neuropathic pain. Brain Behav Immun. 2017;66:94–102.
- Cervenka L, Agudelo LZ, Ruas JL. Kynurenines: tryptophan’s metabolites in exercise, inflammation, and mental health. Science. 2017 Jul 28;357(6349):pii: eaaf9794. DOI:10.1126/science.aaf9794
- Wang Q, Liu D, Song P, et al. Tryptophan-kynurenine pathway is dysregulated in inflammation, and immune activation. Front Biosci (Landmark Ed). 2015;20:1116–1143.
- Tanaka T. Species problem in Citrus: a critical study of wild and cultivated units of Citrus, based upon field studies in their native homes (Revisio aurantiacearum). Jsps. 1954;9:139.
- Sato M, Goto T, Inoue E, et al. Dietary intake of immature Citrus tumida hort. ex Tanaka peels suppressed body weight gain and fat accumulation in an acute mouse model of obesity. J Nutr Sci Vitaminol (Tokyo). 2019;65:19–23.
- Kawaii S, Tomono Y, Katase E, et al. Quantitation of flavonoid constituents in citrus fruits. J Agric Food Chem. 1999;47:3565–3571.
- Sharma K, Mahato N, Cho MH, et al. Converting citrus wastes into value-added products: economic and environmently friendly approaches. Nutrition. 2017;34:29–46.
- Roohbakhsh A, Parhiz H, Soltani F, et al. Neuropharmacological properties and pharmacokinetics of the flavonoids hesperidin and hesperetin–a mini-review. Life Sci. 2014;113:1–6.
- Filho CB, Del Fabbro L, de Gomes MG, et al. Kappa-opioid receptors mediate the antidepressant-like activity of hesperidin in the mouse forced swimming test. Eur J Pharmacol. 2013;698:286–291.
- Souza LC, de Gomes MG, Goes AT, et al. Evidence for the involvement of the serotonergic 5-HT(1A) receptors in the antidepressant-like effect caused by hesperidin in mice. Prog Neuropsychopharmacol Biol Psychiatry. 2013;40:103–109.
- Donato F, de Gomes MG, Goes AT, et al. Hesperidin exerts antidepressant-like effects in acute and chronic treatments in mice: possible role of l-arginine-NO-cGMP pathway and BDNF levels. Brain Res Bull. 2014;104:19–26.
- Li CF, Chen SM, Chen XM, et al. ERK-dependent brain-derived neurotrophic factor regulation by hesperidin in mice exposed to chronic mild stress. Brain Res Bull. 2016;124:40–47.
- Goto T, Kubota Y, Tanaka Y, et al. Subchronic and mild social defeat stress accelerates food intake and body weight gain with polydipsia-like features in mice. Behav Brain Res. 2014;270:339–348.
- Golden SA, Covington HE 3rd, Berton O, et al. A standardized protocol for repeated social defeat stress in mice. Nat Protoc. 2011;6:1183–1191.
- Krishnan V, Han MH, Graham DL, et al. Molecular adaptations underlying susceptibility and resistance to social defeat in blain reward regions. Cell. 2007;131:391–404.
- Kim GN, Shin MR, Shin SH, et al. Study of antiobesity effect through inhibition of pancreatic lipase activity of diospyros kaki fruit and Citrus unshiu peel. Biomed Res Int. 2016;2016:1723042.
- Lee YS, Cha BY, Saito K, et al. Effects of a Citrus depressa Hayata (shiikuwasa) extract on obesity in high-fat diet-induced obese mice. Phytomedicine. 2011;18:648–654.
- Dow CA, Going SB, Chow HH, et al. The effects of daily consumption of grapefruit on body weight, lipids, and blood pressure in healthy, overweight adults. Metabolism. 2012;61:1026–1035.
- Coelho RCLA, Hermsdorff HHM, Gomide RS, et al. Orange juice with a high-fat meal prolongs postprandial lipemia in apparently healthy overweight/obese women. Arch Endocrinol Metab. 2017;61:263–268.
- Hawang JT, Kwon DY, Yoon SH. AMP-activated protein kinase: a potential target for the diseases prevention by natural occurring polyphenols. N Biotechnol. 2009;26:17–22.
- Pu P, Gao DM, Mohamed S, et al. Naringin ameliorates metabolic syndrome by activating AMP-activated protein kinase in mice fed a high-fat diet. Arch Biochem Biophys. 2012;518:61–70.
- Costa CA, Cury TC, Cassettari BO, et al. Citrus aurantium L. essential oil exhibits anxiolytic-like activity mediated by 5-HT(1A)-receptors and reduces cholesterol after repeated oral treatment. BMC Complement Altern Med. 2013;13:42.
- Li M, Shao H, Zhang X, et al. Hesperidin alleviates lipopolysaccharide-induced neuroinflammation in mice by promoting the miRNA-132 Pathway. Inflammation. 2016;39:1681–1689.
- Khan MH, Parvez S. Hesperidin ameliorates heavy metal induced toxicity mediated by oxidative stress in brain of wistar rats. J Trace Elem Med Biol. 2015;31:53–60.
- Youdim KA, Dobbie MS, Kuhnle G, et al. Interaction between flavonoids and the blood-brain barrier: in vitro studies. J Neurochem. 2003;85:180–192.
- Amaretti A, Raimondi S, Leonardi A, et al. Hydrolysis of the rutinose-conjugates flavonoids rutin and hesperidin by the gut microbiota and bifidobacteria. Nutrients. 2015;7:2788–2800.
- Takumi H, Mukai R, Ishiduka S, et al. Tissue distribution of hesperetin in rats after a dietary intake. Biosci Biotechnol Biochem. 2011;75:1608–1610.
- Kumar B, Gupta SK, Srinivasan BP, et al. Hesperetin rescues retinal oxidative stress, neuroinflammation and apoptosis in diabetic rats. Microvasc Res. 2013;87:65–74.
- Shimouchi A, Yokota H, Ono S, et al. Neuroprotective effect of water-dispersible hesperetin in retinal ischemia reperfusion injury. Jpn J Ophthalmol. 2016;60:51–61.
- Asikin Y, Taira I, Inafuku - Teramoto S, et al. The composition of volatile aroma components, flavanones, and polymethoxylated flavones in Shiikuwasha (Citrus depressa Hayata) peels of different cultivation lines. J Agric Food Chem. 2012;60:7973–7980.
- Wang J, Hodes GE, Zhang H, et al. Epigenetic modulation of inflammation and synaptic plasticity promotes resilience against stress in mice. Nat Commun. 2018;9:477.
- Woelfer M, Kasties V, Kahlfuss S, et al. The role of depressive subtypes within the neuroinflammation hypothesis of major depressive disorder. Neuroscience 2019 Apr 1;403:93–110. DOI:10.1016/j.neuroscience.2018.03.034
- Haapakoski R, Mathieu J, Ebmeier KP, et al. Cumulative meta-analysis of interleukins 6 and 1β, tumour necrosis factor α and C-reactive protein in patients with major depressive disorder. Brain Behav Immun. 2015;49:206–215.
- Avgustinovich DF, Marenina MK, Zhanaeva SY, et al. Combined effects of social stress and liver fluke infection in a mouse model. Brain Behav Immun. 2016;53:262–272.
- Gadek-Michalska A, Bugajski A, Tadeusz J, et al. Chronic social isolation in adaptation of HPA axis to heterotypic stress. Pharmacol Rep. 2017;69:1213–1223.
- Wang B, Lian YJ, Su WJ, et al. HMGB1 mediates depressive behavior induced by chronic stress through activating the kynurenine pathway. Brain Behav Immun. 2018;72:51–60.
- Fuerting R, Azzinnari D, Bergamini G, et al. Mouse chronic social stress increases blood and brain kynurenine pathway activity and fear behaviour: both effects are reversed by inhibition of indoleamine 2,3-dioxygenase. Brain Behav Immun. 2016;54:59–72.
- Sublette ME, Galfalvy HC, Fuchs D, et al. Plasma kynurenine levels are elevated in suicide attempters with major depressive disorder. Brain Behav Immun. 2011;25:1272–1278.
- Kawamura N, Shinoda K, Sato H, et al. Plasma metabolome analysis of patients with major depressive disorder. Psychiatry Clin Neurosci. 2018;72:349–361.
- Kuwano N, Kato TA, Setoyama D, et al. Tryptophan-kynurenine and lipid related metabolites as blood biomarkers for first-episode drug-naïve patients with major depressive disorder: an exploratory pilot case-control study. J Affect Disord. 2018;231:74–82.
- Setoyama D, Kato TA, Hashimoto R, et al. Plasma metabolites predict severity of depression and suicidal ideation in psychiatric patients-A multicenter pilot analysis. PLoS One. 2016;11:e0165267.
- Harry GJ, Kraft AD. Neuroinflammation and microglia: considerations and approaches for neurotoxicity assessment. Expert Opin Drug Metab Toxicol. 2008;4:1265–1277.