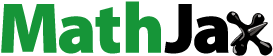
ABSTRACT
Tyrosinase is the key enzyme that controls melanin formation. We found that a hot water extract of the lyophilized fruiting body of the fungus Lyophyllum decastes inhibited tyrosinase from Agaricus bisporus. The extract was fractionated by ODS column chromatography, and an active compound was obtained by purification through successive preparative HPLC using an ODS and a HILIC column. Using spectroscopic data, the compound was identified to be an uncommon amino acid, 6-hydroxytryptophan. 6-Hydroxy-L-tryptophan and 6-hydroxy-D-tryptophan were prepared through a Fenton reaction from L-tryptophan and D-tryptophan, respectively. The active compound was determined to be 6-hydroxy-L-tryptophan by comparison of their circular dichroism spectra and retention time on HPLC analysis of the Nα-(5-fluoro-2,4-dinitrophenyl)-L-leuciamide derivative with those of 6-hydroxy-L-tryptophan and 6-hydroxy-D-tryptophan. A Lineweaver–Burk plot of the enzyme reaction in the presence of 6-hydroxy-L-tryptophan indicated that this compound was a competitive inhibitor. The IC50 values of 6-hydroxy-L-tryptophan was 0.23 mM.
ABSTRACT
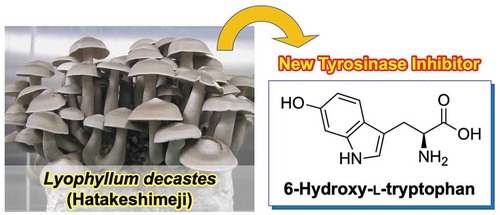
The tyrosinase inhibitor, 6-hydroxy-L-tryptophan, was isolated from the hot water extract of Lyophyllum decastes fruiting body.
The species that produce visible fruiting bodies in the fungi kingdom are regarded as mushrooms, and the majority of them belong to the phylum Basidiomycetes. In natural product chemistry, mushrooms have not been extensively analyzed because their cultivation needs highly technical knowledge and dedicated equipments. However, numerous bioactive compounds, such as medicines, neuroactive substances, plant growth regulators, have been identified from the filamentous fungi[Citation1]. Thus, it is reasonable to assume that mushrooms are unique and promising bioresources that should be investigated further.
Melanin is a pigment that is produced and deposited in the melanocytes present in the stratum basale of the epidermal layer of skin. Because the emergence of mottles and freckles is caused by hypermelanogenesis, the inhibition of melanin deposition is important for maintaining healthy skin. Melanin is biosynthesized from l-tyrosine in mammalian cells. Tyrosinase (EC 1.14.18.1) catalyzes the two steps of the conversion of l-tyrosine to L-dopaquinone via l-3,4-dihydroxyphenylalanine (l-DOPA), and is the rate-limiting enzyme in the melanin biosynthesis pathway[Citation2]. L-Dopaquinone is further metabolized, and finally generates melanin by the polymerization of the intermediate molecules. The main inhibitors of melanin deposition are inhibitors of tyrosinase, such as hydroquinone [Citation3,Citation4], arbutin[Citation5], kojic acid (5-hydroxy-2-hydroxymethyl-4-pyrone)[Citation6], and glutathione[Citation7]. Recently, rhododendrol (4-(4-hydroxyphenyl)-2-butanol), which is an effective inhibitor of tyrosinase and is used as an ingredient in cosmetics, was reported to cause patches of leucoderma on the skin of the face, neck, and hands because of its toxicity towards melanocytes [Citation8–Citation10]. Effective and safe tyrosinase inhibitors are required to develop new medical and cosmetic materials. We screened for the extracts derived from the fruiting bodies and liquid cultures of edible mushroom strains in an attempt to identify novel tyrosinase inhibitors. We found two compounds, 1,3-dihydroisobenzofuran-4,5,7-triol and 5-methoxy-1,3-dihydroisobenzofuran-4,7-diol, from the extract of Neolentinus lepideus (Fr.) Redhead & Ginns (formerly Lentinus lepideus (Fr.) Fr.)[Citation11].
Melanin plays important physiological roles in other organisms such as insects and fungi. In insects, melanin is formed by oxidation of tyrosine via a similar route with that in mammalian cells[Citation12]. The melanization is a substrantial defense reaction of insects because it is involved in wound healing and sequestration of pathogens[Citation13]. In fungi, melanin is mainly formed by polymerization of 1,8-dihydroxynaphthalene that is biosynthesized from the acetate units via pentaketide pathway[Citation14], but some fungi have been shown to produce melanin from L-DOPA[Citation15]. Melanin has been referred to as “fungal armor” due to the ability of the polymer to protect microorganisms against a broad range of toxic insults[Citation16].
Lyophyllum decastes, commonly known as the fried chicken mushroom (hatakeshimeji in Japanese), is an edible species in the family Lyophyllaceae. This mushroom widely distributes in the temperate zone of the northern hemisphere, and typically forms dense mounds of fruiting bodies in grass and litter near trees possibly grown from dead roots and buried wood[Citation17]. Because the mycelium pot cultivation method was established for the production of the fruiting body of L. decastes[Citation18], this mushroom is commercially available in Japan, where production reached 630 tons in 2017 (http://www.maff.go.jp/j/tokei/kouhyou/tokuyo_rinsan/index.html). The extract of L. decastes has been shown to perform some biological activities. Antitumor activities of the polysaccharides obtained from a hot water extract of L. decastes were reported[Citation19]. In addition, the hot water extract of the powder of the fruiting body showed an inhibitory activity on the angiotensin-I-converting enzyme (ACE)[Citation20]. The extract was also effective in suppressing the development of atopic dermatitis-like skin lesions in model mice[Citation21]. and showed hypocholesterolemic action in rats[Citation22]. However, the substances responsible for these biological activities have not been chemically identified, with the exception of the antitumor polysaccharides[Citation19].
In the preliminary experiment, we found that the hot water extract of L. decastes effectively inhibits activity of tyrosinase from Agaricus bisporus. Based on this finding, we attempted to identify the active compound in this mushroom.
Materials and methods
General procedures
1H- and 13C-NMR spectra were recorded using an Avance II instrument (Bruker, Billerica, MA, USA). High-resolution mass spectra were measured using an Exactive mass spectrometer (Thermo Fisher Scientific, Waltham, MA, USA), and ESI MS were recorded by a Quattro Micro API mass spectrometer (Waters, Milford, MA, USA) connected to an Acquity UPLC (Waters). CD and UV spectra were recorded with a J-820 CD spectrophotometer (JASCO Corporation, Tokyo, Japan) and a U-1900 spectrophotometer (Shimadzu, Kyoto, Japan), respectively. Optical rotation was measured using a SEPA-500 polarimeter (Horiba, Kyoto, Japan). Preparative HPLC was performed with a 10A HPLC system (Shimadzu). Enzyme activity was determined with an Infinite 200 PRO microplate reader (TECAN, Männedorf, Switzerland).
Mushroom strain and culture conditions
Lyophyllum decastes TMIC 30,940 stock was provided by The Tottori Mycological Institute, The Japan Kinoko Research Center Foundation (Tottori, Japan). The medium was prepared by mixing cedar sawdust (Rekisha Shoten, Tsuyama, Japan), dried soybean curd refuse (Orient Generalize, Nara, Japan), wheat bran (Masuda Seifunsho, Kobe, Japan), and rice bran (Tottori Kenshoku, Kurayoshi) at the gravimetric ratio of 57:17:11.3:14.7, and tap water was added to this medium to adjust moisture content to 65%. The medium was placed in a polypropylene bag (200 × 450 × 120 mm) to form a medium block (200 × 450 × 120 mm) and autoclaved at 121°C for 60 min. After inoculation of the mushroom, the medium was incubated at 23 ± 1°C under 65 ± 2% humidity for 60 days. Then, the polypropylene bag covering the fruiting body primordia was removed. Then, the medium block was incubated at 16 ± 1°C with 92 ± 1% humidity under 200 lux light (8 h/16 h, light/dark cycles) for approximately 20 days and the formed fruiting bodies were harvested.
Tyrosinase inhibitory activity assay
Tyrosinase activity was determined using the method described by Shimizu et al. [Citation23] and Arung et al. [Citation24] with slight modifications. In brief, a mixture consisting of 59 µL of phosphate buffer (0.1 M, pH 6.5), 47 µL of 2.5 mM l-DOPA (Wako Pure Chemical Industries, Osaka, Japan), and 70 µL of sample solution was preincubated at 25°C for 15 min in a well of a microplate. Next, to initiate the reaction, 24 µL of tyrosinase (12.8 units, extracted from Agaricus bisporus, Sigma-Aldrich, St. Louis, MO, USA) in phosphate buffer (0.1 M, pH 6.5) was added to the mixture. The reaction mixture was incubated at 25°C for 5 min, and absorbance was recorded at 475 nm. The inhibition rate was calculated according to the following equation:
where A is the absorbance at 475 nm for the complete reaction; B and C are the values of the reaction mixtures without tyrosinase and the sample solution, respectively; and D is the value of the reaction mixture without both tyrosinase and the sample solution. Tyrosinase and the sample solution were replaced by phosphate buffer and distilled water, respectively. For comparison, we measured inhibitory activity of 5-hydroxytryptohan (Wako Pure Chemical Industries) and hydroqinone (Wako Pure Chemical Industries).
Purification of active compounds
Lyophilized powder (4 g) of the L. decastes fruiting body was added to distilled water (100 mL) and kept in boiling water for 30 min. The mixture was centrifuged (2,500 × g, 25°C, 10 min) and the supernatant was used as the crude extract of L. decastes. The extract was applied onto an ODS column (Cosmosil 75C18-OPN, 340 g, Nacalai Tesque, Kyoto, Japan) that had been equilibrated with water. Compounds were eluted by mixtures of methanol and water (0%, 20%, 40%, 60%, 80%, and 100% methanol in water, 2 L each). The fractions were concentrated to 100 mL and used for the enzyme assay. The 20% methanol fraction was further (Cosmosil 75C18-OPN, 30 g) fractionated through ODS column chromatography because the inhibitory activity was detected in this fraction. The compounds were eluted with mixtures of methanol and water (0%, 5%, 10%, 20%, 30%, and 100% methanol in water, 240 mL each). The obtained fractions were concentrated to 5 mL and used for enzyme assay. The inhibitory activity was eluted in a 5% methanol fraction. The fraction was subjected to preparative HPLC. The HPLC conditions were as follows: column: Cosmosil 5C18-AR-II (10 mm × 250 mm, Nacalai Tesque); elution: 3% methanol in water; flow rate: 3.0 mL/min; column temperature: 40°C; detection: UV 220 nm. The activity was detected in the fraction corresponding to the peak at 8.3 min. This fraction was further subjected to preparative HPLC with a HILIC column. The HPLC conditions were as follows: column: Cosmosil HILIC (10 mm × 250 mm, Nacalai Tesque); solvent: water (A) and acetonitrile (B); gradient: 80%-60% B/(A + B) within 30 min; column temperature: 40°C; detection: UV 220 nm. The activity was detected in a fraction corresponding to a peak at 15.6 min. This fraction was concentrated to give an active compound (2.1 mg).
Negative ESI MS: m/z 219 [M − H]−; HR ESIMS m/z: 219.0775 [M − H]− (calc. for C11H11O3N2: 220.0770). CD spectrum (10 µg/mL, methanol): Δε 5.0 (227 nm). NMR data are shown in .
Table 1. NMR data for active compound (D2O).
Preparation of 6-hydroxy-L/D-tryptophan
6-Hydroxy-L-tryptophan and 6-hydroxy-D-tryptophan were prepared from L- and D-tryptophan, respectively, by the Fenton reaction according to the method described by Maskos et al. (1992)[Citation25]. L-Tryptophan (300 mg, 1.47 mmol, Wako Pure Chemical Industries) and ferrous EDTA (309 mg, 0.73 mmol, Wako Pure Chemical Industries) were dissolved in a 0.1 M phosphate buffer (pH 6.7, 30 mL), and a solution of 25 mM hydrogen peroxide (85 µL, Santoku Chemical Industries, Tokyo, Japan) was added to the mixture. After stirring for 10 min, 0.1% trifluoroacetic acid in water (10 mL) was added to the mixture. The mixture was applied onto an ODS column (Cosmosil 75C18-OPN, 60 g). Compounds were eluted with 0.1% trifluoroacetic acid in water (750 mL), and then with the mixtures of methanol and 0.1% trifluoroacetic acid in water (5%, 10%, and 20% methanol, 450 mL each). 6-Hydroxy-L-tryptophan was eluted in a 5% methanol fraction. Thus, from this fraction, 6-hydroxy-L-tryptophan was purified by successive preparative HPLC using an ODS column and a HILIC column. The same HPLC conditions for the purification of the natural compound were applied for synthetic 6-hydroxy-L-tryptophan. 6-Hydroxy-D-tryptophan was synthesized by the same procedure.
6-Hydroxy-L-tryptophan (2.9 mg, 0.013 mmol, yield: 0.9%). [α]D − 14.1° (2.0 mg/mL, 18.3°C, methanol), CD spectra: Δε 5.0 (227 nm) (10 µg/mL, methanol), UV spectra (10 µg/mL, methanol), λmax nm (ε): 272.5 (3259), 294.0 (3652). 1H NMR (600 MHz, D2O) δH: 7.56 (1H, d, J = 8.4 Hz, H-4), 7.16 (1H, s, H-2), 6.96 (1H, d, J =1.8 Hz, H-7), 6.77 (1H, dd, J = 8.4, 1.8 Hz, H-5), 4.01 (1H, dd, J = 8.4, 4.8 Hz, H-α), 3.42 (1H, dd, J = 15.6, 4.8 Hz, H-β), 3.23 (1H, dd, J = 15.6, 8.4 Hz, H-β).
6-Hydroxy-D-tryptophan (5.8 mg, 0.026 mmol, yield: 1.8%). [α]D + 14.0° (2.0 mg/mL, 18.6°C, methanol), CD spectra: Δε −5.3 (227 nm) (10 µg/mL, methanol). UV spectrum (10 µg/mL, methanol), λmax nm (ε): 272.5 (3256), 294.0 (3586). 1H NMR (600 MHz, D2O) δH: 7.55 (1H, d, J = 8.4 Hz, H-4), 7.15 (1H, s, H-2), 6.95 (1H, d, J =1.8 Hz, H-7), 6.77 (1H, dd, J = 8.4, 1.8 Hz, H-5), 4.01 (1H, dd, J = 7.8, 4.8 Hz, H-α), 3.41 (1H, dd, J = 15.0, 4.8 Hz, H-β), 3.23 (1H, dd, J = 15.0, 7.8 Hz, H-β).
Derivatization of 6-hydroxytryptophan with Nα-(5-fluoro-2,4-dinitrophenyl)-L-leuciamide
6-Hydroxy-L/D-tryptophan were derivatized with Nα-(5-fluoro-2,4-dinitrophenyl)-L-leuciamide (FDLA, Tokyo Chemical Industry, Tokyo, Japan) to analyze the composition of D- and L- enantiomers[Citation26]. A one percent solution of FDLA in acetone (100 µL) and a 1 M solution of NaHCO3 (20 µL) were added to a 50 mM aqueous solution of 6-hydroxytryptophan (50 µL). After a 1-hr stirring at 37°C, 1 N HCl (20 µL) was added to the mixture. The mixture was diluted 10-fold with acetonitrile and subjected to HPLC analysis. The active compound purified from L. decastes was also derivatized in the same way, except that the concentration of the solution of 6-hydroxytryptophan was changed to 0.45 mM. The conditions for the UPLC analysis were as follows: column: ACQUITY UPLC BEH C18 1.7 µm 2.1 × 50 mm (Waters); solvent: 0.1% formic acid in water (A) and 0.1% formic acid in acetonitrile (B); gradient: 5%-70% B/(A + B) within 10 min, flow rate: 0.2 mL/min; column temperature: 40°C; detection: 200–600 nm.
Determination of the contents of 6-hydroxy-L-tryptophan and related compounds in L. decastes
The contents of 6-hydroxy-L-tryptophan and tryptophan in the hot water extract of the freeze-dried fruiting body of L. decastes were analyzed by HPLC. Authentic tryptophan were purchased from Wako Pure Chemical Industries. HPLC conditions were as follows: column: Cosmosil AR-II 4.6 × 150 mm; solvent: 0.1% trifluoroacetic acid in water (A) and methanol (B); gradient: 5%-80% B/(A + B) within 30 min; flow rate: 0.8 mL/min; column temperature: 40°C; detection: 280 nm.
Results
The hot water extract of the freeze-dried fruiting body of L. decastes inhibited tyrosinase activity by 48%. To purify the active compounds, we first fractionated the extract through ODS column chromatography. The 20% methanol fraction showed the strongest inhibitory activity (12.7% inhibition). This fraction was further fractionated by ODS column chromatography. The resulting 5% faction showed the strongest activity (36% inhibition). The repeated preparative HPLC of this fraction with an ODS and a HILIC column resulted in the isolation of 2.1 mg of active compound.
The molecular weight of this compound was determined as 220 by LC-MS analysis on the basis of the detection of [M-H]− ion at m/z 219. HR ESI MS data showed the peak of [M-H]− at m/z 219.0775, establishing the molecular formula of the compound as C11H12O3N2; thus, the hydrogen deficiency index was determined to be 7. Four 1H NMR signals (δH 7.55, 7.15, 6.95, and 6.77 ppm) and eight 13C NMR signals (δC 152.3, 137.9, 124.5, 121.8, 120.0, 110.1, 108.2, and 97.9 ppm) suggested the presence of a monosubstituted indole ring. The coupling pattern of three signals of aromatic protons (δH 7.55, 6.95, and 6.77 ppm) and the chemical shift of the 13C NMR signal at δC 152.3 suggested the substitution of a proton by an oxygen atom at 5- or 6-position on the indole ring. The chemical shift of the 13C NMR signal at δC 175.0 indicated the presence of a carboxyl group. The coupling pattern of three proton signals at δH 4.01, 3.41, and 3.23 ppm suggested the presence of a methine group with a chiral carbon bonding to a methylene group. Based on this information, we assumed the active compound to be 5- or 6-hydroxytryptophan. The UV-Vis spectrum of active compound was identical with previously reported spectrum of 6-hydroxytryptohan (), but clearly different from that of 5-hydroxytryptophan[Citation25]. Further, the NMR data of the active compound were similar to the data in previous literature [Citation27]) indicating that the compound is 6-hydroxytryptophan ().
To confirm this conclusion, and to determine the stereochemistry of the purified compound, we prepared the 6-hydroxy-L-tryptophan and 6-hydroxy-D-tryptophan from L- and D-tryptophan, respectively, using the Fenton reaction. The CD spectra of the enantiomers were mirror images of each other (). The CD spectrum of the purified active compound was similar to that of synthesized 6-hydroxy-L-tryptophan.
Figure 2. Determination of the stereochemistry of the active compound.
(a) CD spectra of the active compound purified from the L. decastes fruiting body (red line), 6-hydroxy-L-tryptophan (green line), and 6-hydroxy-D-tryptohan (blue line). (b) UPLC analysis of FDLA derivatives of the active compound (red line) purified from the L. decastes fruiting body and a mixture of 6-hydroxy-L-tryptophan and 6-hydroxy-D-tryptohan (black line).
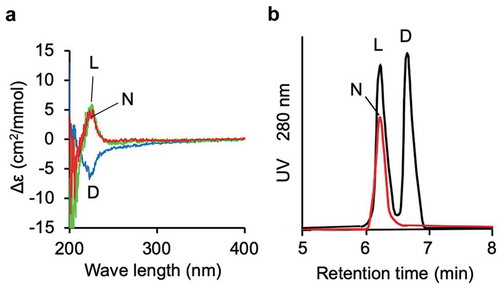
To examine the presence of a small amount of 6-hydroxy-D-tryptophan in the purified compound, we derivatized the compound purified from the mushroom with FDLA and subjected it to UPLC analysis (). No peak corresponding to an FDLA derivative of 6-hydroxy-D-tryptophan was detected through the analysis, indicating that the compound was optically pure 6-hydroxy-L-tryptophan.
By using the synthesized 6-hydroxy-L-tryptophan as a standard, we determined the contents of 6-hydroxy-L-tryptophan, together with tryptophan in the freeze-dried fruiting body of L. decastes by HPLC analysis. Their contents determined to be 0.58 and 0.96 mg/gDW, respectively.
We performed reactions with mushroom tyrosinase using various concentrations of 6-hydroxy-L-tryptophan, 6-hydroxy-D-tryptophan, and 5-hydroxy-L-tryptophan, as well as the known tyrosinase inhibitor hydroquinone (). The IC50 values of these compounds were 0.23 mM, 1.87 mM, 17.94 mM, and 9.82 mM, respectively.
Figure 3. Inhibition of tyrosinase by 6-hydroxy-L-tryptophan (green circles), 6-hydroxy-D-tryptophan (blue squares), 5-hydroxy-L-tryptophan (orange triangles), and hydroquinone (violet diamonds).
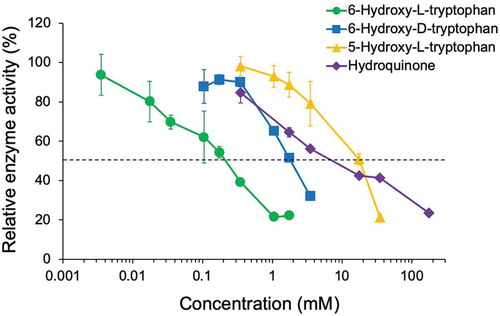
Next, we performed enzyme reactions in the presence of 6-hydroxy-L-tryptophan at 0.175 mM and 0.07 mM to determine the mode of inhibition. In the Lineweaver–Burk plot (), the regression lines crossed on the y-axis, indicating competitive inhibition. We determined the Ki value of 6-hydroxy-L-tryptophan to be 0.11 mM on the basis of the equation given by Cheng and Prusoff (1973)[Citation28].
Discussion
We identified 6-hydroxy-L-tryptophan to be the tyrosinase inhibitor in L. decastes. 6-Hydroxy-L-tryptophan has not been reported from mushrooms. The IC50 value of 6-hydroxy-L-tryptophan for tyrosinase was 0.23 mM. This value was much smaller than that for hydroquinone, indicating that 6-hydroxy-L-tryptophan is a powerful inhibitor of tyrosinase in vitro. The crude extract of L. decastes showed 48% inhibition of tyrosinase activity. The 6-hydroxy-L-tryptophan concentration in the crude extract was determined to be 23 µg/mL. This value is almost half of the IC50 value, which is 50.6 µg/mL (0.23 mM). Thus, the concentration of 6-hydroxy-L-tryptophan in the crude extract was not sufficient to explain the 48% inhibition. Other components in the extract likely inhibited the enzyme activity.
6-Hydroxy-L-tryptophan is a simple but rare amino acid, and its occurrence of in natural resources has not been reported. 6-Hydroxytryptophan has attracted relatively less attention, considering the limited number of papers that deal with this compound. Our database search with SciFinder on 6-hydroxytryptophan found only 32 papers and five patents. The reported biological activities of 6-hydroxytryptophan are antioxidant activity [Citation29]) and inhibitory activity on the tyrosine hydroxylase involved in the biosynthesis of norepinephrine[Citation30]. N-Acetyl-6-hydroxytryptophan was identified as the precursor of conidiophore pigment of Aspergillus nidulans, and was shown to be the natural substrate of a specific phenol oxidase that involved in the biosynthesis of the pigment[Citation31].
6-Hydroxy-L-tryptophan showed a strong activity in comparison with 5-hydroxy-L-tryptophan, with a 78-fold difference in IC50 values (). This finding clearly indicates the importance of the position of the hydroxy group on the indole ring on the inhibitory activity. In addition, there was an 8.1-fold difference in the inhibitory activity against tyrosinase between 6-hydroxy-L-tryptophan and 6-hydroxy-D-tryptophan. Thus, the stereochemistry of the amino acid is recognized by the tyrosinase. Generally, the tyrosinases accept a wide range of monophenols and polyphenols as substrate. Although the natural substrate for the mushroom tyrosinase has not been identified, it is likely that the enzyme has high substrate specificity for the natural substrate. The differential inhibitory activity of hydroxytryptophans may be a reflection of the substrate specificity.
Multiple compounds with tyrosinase inhibitory activity have been isolated from fungi. These compounds include azelaic acid (1,7-heptanedicarboxylic acid) from Pityrosporum ovale[Citation32], kojic acid from Aspergillus and Penicillium[Citation33], and agaritine (β-N-(γ-L-glutamyl)-4-hydroxymethyphenylhydrazine) from Agaricus bisporus[Citation34]. We previously isolated two potent tyrosinase inhibitors, 1,3-dihydroisobenzofuran-4,5,7-triol and 5-methoxy-1,3-dihydroisobenzofran-4,7-diol, from Neolentinus lepideus[Citation11]. Edible mushrooms may be a promising source of novel tyrosinase inhibitors.
Author contribution
A.I. and E.S. designed the experiments. N.S. and T.B. performed the experiments, while T.B., N.U., and K.U. contributed to the discussion of the experimental details. E.S. and Y.O. cultured L. decastes and first found the tyrosinase inhibitory activity of its water extract. A.I and N.S. analyzed the data and edited the manuscript.
Acknowledgments
We thank Ms. Mizuki Yokono, Technical Department, Tottori University, for her assistance with HR-ESI-MS.
Disclosure statement
No potential conflict of interest was reported by the authors.
References
- Cole RJ, Schweikert MA, Jarvis BB. Handbook of Secondary Fungal Metabolites Volumes 1-3. Amsterdam: Elsevier; 2003.
- Sánchez-Ferrer A, Rodríguez-López JN, García-Gánovas F, et al. Tyrosinase: a comprehensive review of its mechanism. Biochim Biophys Acta. 1995;1247:1–11.
- Arandt KA, Fitzpatrick TB. Topical use of hydroquinine as a depigmenting agent. J Am Med Assoc. 1965;194:117–119.
- Fitztrick TB, Arandt KA, El-Mofty AMMA. Hydroquinone and psoralens in the therapy of hypermelanosis and vitiligo. Arch Dermatol. 1966;93:589–600.
- Maeda K, Fukuda M. In vitro effectiveness of several whitening cosmetic components in human melanocyte. J Soc Cosmet Chem. 1991;42:361–368.
- Kligman AM, Willis I. A new formula for depigmenting human skin. Arch Dermatol. 1975;111:40–48.
- Matsuki M, Watanabe T, Ogasawara A, et al. Inhibitory mechanism of melanin synthesis by glutathione. J Pharm Soc Jpn (Yakugaku Zasshi). 2008;128:1203–1207.
- Aoyama Y, Ito A, Suzuki K, et al. The first epidemiological report of rhododenol-induced leukoderma in Japan based on a nationwide survey. Nippon Hifuka Gakkai Zasshi. 2014;124:2095–2109.
- Suzuki K, Aoyama Y, Ito A, et al. The second epidemiology report of rhododenol-induced leukoderma in Japan based on a nationwide survey. Nippon Hifuka Gakkai Zasshi. 2014;124:3125–3142.
- Sasaki M, Kondo M, Sato K, et al. Rhododendrol, a depigmentation-inducing phenolic compound, exerts melanocyte cytotoxicity via a tyrosinase-dependent mechanism. Pigment Cell Melanoma Res. 2014;27:754–763.
- Ishihara A, Ide Y, Bito T, et al. Novel tyrosinase inhibitors from liquid culture of Neolentinus lepideus. Biosci Biotechnol Biochem. 2014;82:22–30.
- Chen P, Li L, Wang J, et al. BmPAH catalyzes the initial melanin biosynthetic step in Bombyx mori. Plos One. 2018;8:e71984.
- Ashida M, Brey PT. Role of the integument in insect defense: pro-phenol oxidase cascade in the cuticular matrix. Proc Natl Acad Sci USA. 1995;92:10698–10702.
- Wheeler MH. Comparisons of fungal melanin biosynthesis in ascomycetous, imperfect and basidiomycetous fungi. Trans Br Mycol Soc. 1983;81:29–36.
- Langfelder K, Streibel M, Jahn B, et al. Biosynthesis of fungal melanins and their importance for human pathogenic fungi. Fungal Genet Biol. 2003;38:143–158.
- Gómez BL, Nosanchuk JD. Melanin and fungi. Curr Opin Infect Dis. 2003;16:91–96.
- Roberts P, Evans S. The book of fungi. A life-size guide to six hundred species from around the world. East Sussex, UK: Ivy Press; 2011. p. 219.
- Hara H, Sonoda T, Itoh M; Japan Kokai Tokkyo Koho. Japan Patent Office; H2-41295. 1990.
- Ukawa Y, Hisamatsu M, Ito H. Antitumor effects of (1→3)-β-D-glucan and (1→6)-β-D-glucan purified from newly cultivated mushroom, Hatakeshimeji (Lyophyllum decastes Sing.). J Biosci Bioeng. 2000;90:98–104.
- Ukawa Y, Andou M, Furuichi Y, et al. Angiotensin I-converting enzyme inhibitory activity and antitumor activity of Hatakeshimeji (Lyophyllum decastes Sing.). Nippon Shokuhin Kagaku Kogaku Kaishi. 2001;48:58–63, in Japanese
- Ukawa Y, Izumi Y, Ohbuchi T, et al. Oral administration of the extract from hatakeshimeji (Lyophyllum decastes Sing.) mushroom inhibits the development of atopic eermatitis-like skin lesions in NC/Nga mice. J Nutr Sci Vitaminol. 2007;53:293–296.
- Ukawa Y, Furuichi Y, Kokean Y, et al. Effect of hatakeshimeji (Lyophyllum decastes Sing.) mushroom on serum lipid levels in rats. J Nutr Sci Vitaminol. 2002;48:73–76.
- Shimizu K, Kondo R, Sakai K, et al. The Inhibitory components from Artocarpus incisus on melanin biosynthesis. Planta Med. 1998;64:408–412.
- Arung ET, Shimizu K, Kondo R. Inhibitory effect of artocarpanone from Artocarpus heterophyllus on melanin biosynthesis. Biol Pharm Bull. 2006;29:1966–1969.
- Maskos Z, Rush JD, Koppenol WH. The hydroxylation of tryptophan. Arch Biochem Biophys. 1992;296:514–520.
- Fujii K, Ikai Y, Oka H, et al. A nonempirical method using LC/MS for determination of the absolute configuration of constituent amino acids in a peptide: combination of Marfey’s method with mass spectrometry and its practical application. Anal Chem. 1997;69:5146–5151.
- van Wickern B, Muller B, Simat T, et al. Determination of γ-radiation induced products in aqueous solutions of tryptophan and synthesis of 4-, 6- and 7-hydroxytryptophan. J Chromatogr A. 1997;786:57–65.
- Cheng Y, Prusoff WH. Relationship between the inhibition constant (K1) and the concentration of inhibitor which causes 50 per cent inhibition (I50) of an enzymatic reaction. Biochem Pharmacol. 1973;22:3099–3108.
- Farmanzadeh D, Najafi M. On the antioxidant activity of the tryptophan derivatives. Bull Chem Soc Jpn. 2013;86:1041–1050.
- Zhelyaskov DK, Levitt M, Udenfriend S. Tryptophan derivatives as inhibitors of tryrosine hydroxylase in vivo and in vitro. Mol Pharmacol. 1968;4:445–451.
- McCorkindale NJ, Hayes D, Johnston GA, et al. N-acetyl-6-hydroxytryptophan a natural substrate of a monophenol oxidase from Aspergillus nidulans. Phytochemistry. 1983;22:1026–1028.
- Schallreuter KU, Wood JW. A possible mechanism of action for azelaic acid in the human epidermis. Arch Dermatol Res. 1990;282:168–171.
- Parrish FW, Wiley BJ, Simmons EG, et al. Production of aflatoxins and kojic acid by species of Aspergillus and Penicillium. Appl Microbiol. 1966;14:139.
- Espín JC, Jolivet S, Wichers HJ. Inhibition of mushroom polyphenol oxidase by agaritine. J Agric Food Chem. 1998;49:2976–2980.