ABSTRACT
A low-temperature protein expression system is useful for the production of thermolabile proteins. We previously developed a system that enables constitutive protein production at low temperatures, using the psychrotrophic bacterium Shewanella livingstonensis Ac10 as the host. To increase the utility of this system, in the present study, we introduced a repressible promoter of the trp operon of this bacterium into the system. When ß-lactamase was produced under the control of this promoter at 18°C and 4°C, the yields were 75 and 33 mg/L-culture, respectively, in the absence of L-Trp, and the yields were decreased by 72% and 77%, respectively, in the presence of L-Trp. We also found that 3-indoleacrylic acid, a competitive inhibitor of the Escherichia coli trp repressor, increased the expression of the reporter gene. This repressible gene expression system would be useful for regulatable recombinant protein production at low temperatures.
Graphical Abstract

A regulatable low-temperature protein expression system was constructed by using a cold-adapted bacterium as the host and its trp operon promoter.
A number of recombinant protein expression systems have been constructed using various organisms including bacteria [Citation1], eukaryotic microorganisms [Citation2], and mammalian cells [Citation3] as the hosts. However, there are still many proteins that are difficult to overproduce using conventional recombinant protein expression systems. These include low-stability [Citation4] and toxic [Citation5] proteins. To achieve efficient production of these proteins, a low-temperature protein-expression system is expected to be useful. At low temperatures, thermal denaturation of proteins is alleviated. Moreover, as the low temperature expression system can suppress enzyme activity by lowering the temperature, it is also effective for the production of enzymes that may harm the host cells [Citation6]. To date, cold-adapted microorganisms, such as Antarctic sea bacteria, Shewanella livingstonensis Ac10 [Citation7] and Pseudoalteromonas haloplanktis TAC125 [Citation8], have been employed for heterologous protein expression at low temperatures.
S. livingstonensis Ac10 grows well at low temperatures close to 0 °C but cannot grow at temperatures above 30°C [Citation9]. In our previous work, we found a promoter, named LI3, that leads to strong expression of heterologous proteins in this strain and developed a low-temperature expression system using this strain as a host [Citation7]. The LI3 promoter was obtained from the upstream noncoding region of the gene encoding a putative alkyl hydroperoxide reductase (AhpC), which is highly produced in this strain both at 18°C and 4°C. Under the control of this promoter, the maximum yield of the reporter protein ß-lactamase (BLA) was 139 and 91 mg/L-culture at 18°C and 4°C, respectively, in the late stationary phase [Citation7]. We also found that this protein production system is suitable for the production of thermolabile peptidases, PepF and PepQ, from a psychrophilic strain, Desulfotalea psychrophila DSM12343 [Citation7,Citation10]. The production of these proteins in S. livingstonensis Ac10 under the LI3 promoter system was higher than that under the Escherichia coli T7 promoter system. These results demonstrated that the LI3 promoter is useful for constitutive production of foreign proteins, especially those with low thermostability. Nevertheless, there is a drawback of this system: the LI3 promoter is not regulatable; thus, the expression of the gene under the control of this promoter cannot be repressed. This could be problematic in the production of highly toxic proteins, the toxicity of which cannot be sufficiently suppressed even at low temperatures. In this study, we introduced a gene regulatory system of the trp operon into the heterologous protein production system operating at low temperatures using S. livingstonensis Ac10. We assessed its validity and found that the newly developed system is useful for regulatable expression of recombinant proteins at low temperatures.
Materials and methods
Bacterial strains, plasmids, and culture conditions
Bacterial strains used in this study are listed in . As the host, S. livingstonensis Ac10-Rifr, a rifampicin-resistant mutant of S. livingstonensis Ac10, was used. S. livingstonensis Ac10-Rifr was grown under aerobic conditions in Luria-Bertani (LB) medium consisting of 10 g/L tryptone (BD Difco, Detroit, MI), 10 g/L NaCl (Nacalai Tesque, Kyoto, Japan), and 5 g/L yeast extract (BD Difco) for 24 h at 18°C, and then transferred to modified DSMZ medium 79 (a minimal synthetic sea water medium containing 1 g/L KH2PO4, 1 g/L NH4NO3, 10 g/L NaCl, 0.2 g/L MgSO4 · 7H2O, 10 mg/L FeSO4, and 10 mg/L CaCl2 · 2H2O) supplemented with 0.5% w/v casamino acids as carbon source (BD Difco), which was used as the basal medium throughout the present study [Citation11]. The cells grown in this medium at 18°C and 4°C were harvested in the log phase. As needed, 50 µg/mL of rifampicin (Sigma Aldrich, St. Louis, MO) and/or 30 µg/mL of chloramphenicol (Nacalai Tesque) were added. For regulation of gene expression, 0.1% (w/v) L-Trp (Kyowa Hakko Bio, Tokyo, Japan) or 40 µg/mL of 3-indoleacrylic acid (3-IAA) (Tokyo Chemical Industry, Tokyo, Japan) was added. E. coli DH5α was used as the host for plasmid construction, and E. coli S17-1/λpir was used as the donor strain in the conjugation experiments. E. coli DH5α and E. coli S17-1/λpir were grown in LB broth in the presence or absence of chloramphenicol (30 µg/mL). A derivative of a broad-host-range vector, pJRD215 [Citation12], containing the chloramphenicol selection cassette, pJRD-Cmr [Citation13], was used to construct plasmids for protein expression in S. livingstonensis Ac10-Rifr. Promoter-assay plasmids, pT1bla to pT10bla, constructed as described below were introduced into S. livingstonensis Ac10-Rifr to obtain the strains BLAT1 to BLAT10, respectively. BLAT0 was prepared as a control strain harboring pJRD-Cmr.
Table 1. Strains and plasmids used in this study.
Construction of plasmids
Plasmids and primers used in this study are listed in and S1, respectively. Q5 High-Fidelity Polymerase and NEBuilder HiFi DNA Assembly Master Mix were purchased from New England BioLabs, Ipswich, MA. Construction of the plasmids for the promoter assay is summarized in .
Figure 1. Construction of plasmids for the promoter assay.
rep: replication-related gene, mob: mobilization-related gene, oriV: replication origin, Cmr: chloramphenicol resistance gene, MluI and SpeI: restriction enzyme sites.
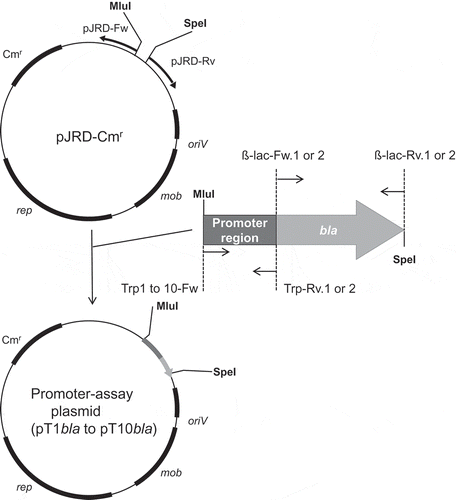
Each of the predicted promoter regions was amplified from the upstream region of the trpE gene () by using the following primer sets: Trp1-Fw/Trp-Rv.1 for the T1 region, Trp2-Fw/Trp-Rv.1 for the T2 region, Trp3-Fw/Trp-Rv.1 for the T3 region, Trp4-Fw/Trp-Rv.1 for the T4 region, Trp5-Fw/Trp-Rv.1 for the T5 region, Trp6-Fw/Trp-Rv.2 for the T6 region, Trp7-Fw/Trp-Rv.1 for the T7 region, Trp8-Fw/Trp-Rv.1 for the T8 region, Trp9-Fw/Trp-Rv.2 for the T9 region, and Trp10-Fw/Trp-Rv.1 for the T10 region (Table S1 “Amplification of predicted promoter regions” section). By using the primer set, pJRD-Fw/pJRD-Rv, a linear plasmid fragment of pJRD-Cmr was amplified with Q5 High-Fidelity DNA Polymerase. BLA-coding gene was amplified from pBR322 by using the primer set, ß-lac-Fw.1/ß-lac-Rv.1 or ß-lac-Fw.2/ß-lac-Rv.2. The purified linear plasmid fragment and each of the insert gene fragments consisting of the predicted promoter regions and the BLA-coding gene were subjected to the NEBuilder HiFi DNA Assembly Cloning System to generate promoter-assay plasmids pT1bla to pT10bla.
Figure 2. The trp operon of S. livingstonensis Ac10 and the 5ʹ-untranslated region (5ʹ-UTR) of trpE tested for its promoter activity.
(a) Genetic region containing the trp operon of S. livingstonensis Ac10. The scale bar represents 1 kb. (b) Schematic illustration of 5ʹ-UTR of trpE tested for its promoter activity by using the BLA gene as a reporter. DNA fragments of the 5ʹ-UTR of trpE (T1–T10) were fused with the BLA-coding gene and introduced into the promoter-assay plasmid as shown in . Putative promoter sequences were predicted by Neural Network Promoter Prediction (ver.2.2) (http://www.fruitfly.org/seq_tools/promoter.html), and the locations of the sequences with a prediction score over 0.95 are indicated with triangles.
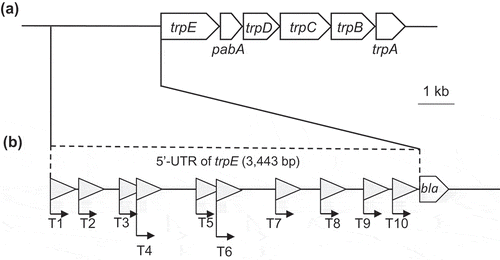
Conjugative transformation of S. livingstonensis Ac10-Rifr
pJRD-Cmr and its derivatives were introduced into E. coli S17-1/λpir and then transferred to S. livingstonensis Ac10-Rifr by conjugation as follows. E. coli S17-1/λpir harboring pJRD-Cmr and its derivatives was cultivated at 37°C aerobically in 5 mL of LB medium with 30 µg/ml chloramphenicol until the optical density at 600 nm (OD600) reached about 1.0. S. livingstonensis Ac10-Rifr was cultivated in 5 mL of LB medium at 18°C aerobically until the OD600 reached about 2.0. These cells were washed twice with fresh LB medium without antibiotics. After washing, 100 µL of the cultures of each of the donor cells and the recipient cells were mixed, and the mixture was incubated on LB plate for 24 h at 18°C. The bacteria grown on the plate were collected with LB medium and spread onto an LB plate containing 30 µg/mL chloramphenicol and 50 µg/mL rifampicin. The plates were incubated at 18°C, and the transformants were selected.
Quantitative real-time RT-PCR analysis
Total RNA was extracted using the High Pure RNA Isolation Kit (Roche, Basel, Switzerland) from the cells cultivated in modified DSMZ medium 79 with 0.5% w/v casamino acids supplementation and with or without L-Trp supplementation. Total RNA was dissolved in 0.1% diethyl pyrocarbonate (Nacalai Tesque)-treated water and stored at –80°C until use. Quantitative real-time RT-PCR was performed with SuperScript III Platinum SYBR Green One-Step qRT-PCR Kit (Invitrogen, Waltham, CA) and the Mx3000P Multiple Quantitative RT-PCR system (Agilent Technologies, Santa Clara, CA). The amplification curve was obtained from RT-PCR reaction mixtures containing 0.1–1,000 pg of the purified total RNA extract of S. livingstonensis Ac10. Primers listed in the “Real-time RT-PCR” section of Table S1 were used to amplify the internal region of the genes involved in tryptophan synthesis (trpA to trpE). The reaction conditions were as follows: 95°C for 10 min and 50 cycles of 95°C for 30 s, 55°C for 30 s, and 72°C for 30 s. The threshold cycle (Ct) value for each sample was normalized with the Ct value for 16S rRNA.
BLA assay
The recombinant S. livingstonensis Ac10-Rifr strains harboring each of the promoter-assay plasmids were cultivated aerobically in liquid modified DSMZ medium 79 with 0.5% w/v casamino acids supplementation containing 30 µg/mL of chloramphenicol in the presence or absence of 0.1% L-Trp at 18°C and 4°C. The cells were harvested by centrifugation at a temperature identical to the cultivation temperature and resuspended in 500 µL of 100 mM potassium phosphate buffer (pH 7.0). The cells were homogenized by sonication, and the soluble protein extracts were used for the BLA assay. The BLA activity was measured at 25°C with 100 µM nitrocefin (Cayman Chemical, Ann Arbor, MI), a chromogenic substrate, in 100 mM potassium phosphate buffer (pH 7.0). The formation of the product was monitored by measuring the increase of the absorbance at 486 nm with SPECTRA MAX 190 (Molecular Devices, San Jose, CA). The molar extinction coefficient of hydrolyzed nitrocefin is 20,500 M−1·cm−1. One unit of the enzyme was defined as the amount of the enzyme that catalyzed the formation of 1 µmol of the product in 1 min.
Sodium dodecyl sulfate-polyacrylamide gel electrophoresis (SDS-PAGE) and protein quantification
We performed SDS-PAGE to quantify the expression levels of BLA. Cells harvested at stationary phase (OD600 = 1.8–2.0) were applied to 12.5% polyacrylamide gel. Gels were stained with Coomassie Brilliant Blue G-250 in 10% acetic acid, and protein bands of BLA were quantified with ImageJ version 1.52o (imagej.nih.gov/ij/download.html). A standard curve of protein band intensities versus protein amounts was generated from band intensities of protein molecular weight marker, Precision Plus ProteinTM Unstained Protein Standards (Bio-Rad, Hercules, CA).
Cultivation in the presence of 3-IAA
After the cells were grown in LB medium or LB medium containing 0.1% L-Trp until the log phase (OD600 = 0.7–0.8), the cells were harvested and washed once with LB medium containing or absent of 3-IAA. After washing, the cells were transferred to fresh LB medium containing or absent of 3-IAA, which is used as a gratuitous inducer of the trp promoter [Citation16], at a final concentration of 0.1%. After a 2 h incubation at 18°C or 4°C, the cells were harvested by centrifugation. Harvested cells were homogenized by sonication, and the crude extracts were collected by centrifugation for the BLA assay.
Results and discussion
Characterization of the trp operon of S. livingstonensis Ac10
To develop a regulatable protein production system using S. livingstonensis Ac10 as the host, we focused on a gene cluster (DDBJ: LC474873) containing Trp biosynthesis genes assigned as trpA, trpB, trpC, trpD, pabA, and trpE ()). The amino acid sequence identities between TrpA, TrpB, TrpC, TrpD, and TrpE of S. livingstonensis Ac10 and the corresponding proteins of E. coli were 63%, 77%, 45%, 52%, and 50%, respectively. Trp-biosynthesis genes are known to be included in a gene cluster called the trp operon and transcribed together during Trp biosynthesis [Citation17–Citation19]. Expression of the trp operon is suppressed in the presence of excess L-Trp [Citation20,Citation21], and this repressive regulation is found in many organisms [Citation22–Citation24]. Compared with the E. coli trp operon, the putative Trp-biosynthesis gene cluster of S. livingstonensis Ac10 lacks a trpL coding for a leader peptide, which is involved in the regulation of Trp synthesis by attenuation [Citation25]. This suggests that expression of the trp operon of S. livingstonensis Ac10 is mainly regulated by a repressor protein [Citation20,Citation21]. We examined whether the transcriptional regulation system for the trp operon is applicable to regulatable gene expression of recombinant protein production at low temperatures in the following experiments.
Analysis of the transcription level of the Trp-biosynthesis genes in S. livingstonensis Ac10-Rifr
We investigated the effects of L-Trp supplementation on the growth of S. livingstonensis Ac10-Rifr using modified DSMZ medium 79 supplemented with 0.5% w/v casamino acids as a basal medium. Both at 18°C and 4°C, this strain grew better in the absence of L-Trp than in the presence of 0.1% L-Trp ()). The maximum OD600 were 3.5 and 1.7, respectively, at 18°C and 2.0 and 1.0, respectively, at 4°C.
Figure 3. Effects of L-Trp supplementation on the growth of S. livingstonensis Ac10-Rifr and expression of its Trp-biosynthesis genes.
(a, b) Growth curves of S. livingstonensis Ac10-Rifr. The cells were grown at 18°C (a) and 4°C (b) in modified DSMZ medium 79 with 0.5% w/v casamino acids supplementation containing (■) and absent of L-Trp (□) at a final concentration of 0.1%. The statistical analysis was performed using a Student’s t-test from three independent experiments. Error bars indicate SD. An asterisk (*) indicates a statistically significant difference (Student’s t-test, P < 0.01). (c, d) Transcription levels of the Trp-biosynthesis genes. The ratios of the amounts of mRNA of the genes in the trp operon in the cells grown with or without 0.1% L-Trp are shown. The cells were grown at 18°C (c) or 4°C (d) and harvested in the log phase. All values were normalized to the amounts of 16S rRNA. Error bars represent SD from three independent experiments.
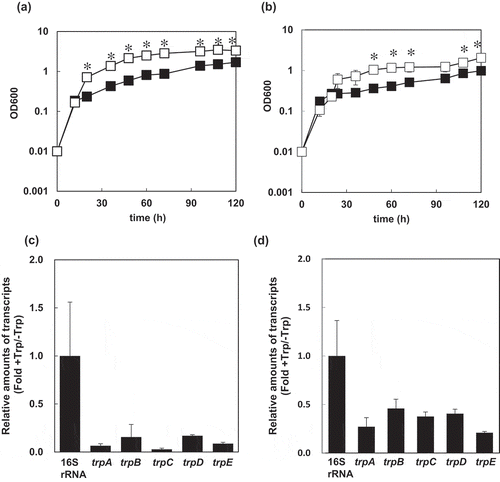
Next, to examine whether the expression of Trp-biosynthesis genes is transcriptionally regulated by L-Trp supplementation, relative amounts of mRNAs of Trp-biosynthesis genes were determined by real-time RT-PCR. In the presence of 0.1% L-Trp, relative amounts of mRNA of trpA, trpB, trpC, trpD, and trpE declined to less than 20% and 40% of those in the absence of L-Trp supplementation at 18°C and 4°C, respectively ()). These results indicated that supplementation of L-Trp suppresses the expression of Trp-biosynthesis enzymes of S. livingstonensis Ac10, and this repressive gene expression system may be applicable to regulatable heterologous protein production using S. livingstonensis Ac10 as the host.
Identification and characterization of a promoter of the trp operon of S. livingstonensis Ac10
Using the promoter predictor (Neural Network Promoter Prediction Ver. 2.2, www.fruitfly.org/seq_tools/promoter.html), we found ten putative promoter regions from the 5ʹ-UTR of the Trp-biosynthesis gene cluster (3,443 bp) ()). The predicted promoter regions, which have conserved sequences of transcriptional regulation elements [Citation26], were named T1, T2, T3, T4, T5, T6, T7, T8, T9, and T10. To investigate their function as promoters, we constructed ten plasmids (pT1bla, pT2bla, pT3bla, pT4bla, pT5bla, pT6bla, pT7bla, pT8bla, pT9bla, and pT10bla) harboring these predicted promoter regions upstream of the BLA-coding gene ()). These plasmids were introduced into S. livingstonensis Ac10-Rifr, and the transformants (named as shown in ) were cultured in the basal medium with or without L-Trp supplementation at 18°C and 4°C to examine the expression of the BLA gene.
All the transformants showed BLA activities both at 18°C and 4°C (). Higher specific activities were observed at 18°C than at 4°C in the absence of L-Trp. At 18°C, the specific activities were in the range of 5.3–27.9 U/mg. The highest specific activity was observed for BLAT9 both at 18°C and 4°C. The specific activity of BLAT9 at 18°C was more than double of that at 4°C. The higher specific activity at 18°C may be partially due to a higher copy number of pJRD-Cmr at this temperature than at 4°C [Citation7]. The predicted promoter T9 has a putative transcription initiation site at -1,186 position from trpE start codon; the site neither was empirically determined nor could be validated from sequence inspection (e.g. no apparent trp operator sequence in the vicinity [Citation27]). Further experiments will be needed in the future to identify the mode of action of T9 promoter.
Figure 4. Production of BLA by recombinant S. livingstonensis Ac10-Rifr harboring various promoter-assay plasmids.
Specific activities of BLA produced by recombinant S. livingstonensis Ac10-Rifr cells (BLAT 1 to BLAT10) harboring the promoter-assay plasmids, pT1bla to pT10bla, respectively, are shown. BLAT0 is a negative control strain harboring pbla, which contains the BLA gene without a promoter sequence. The cells were grown at 18°C (a) and 4°C (b) in the presence (open bars) or absence (closed bars) of 0.1% L-Trp and harvested in the stationary phase (OD600 = 1.8–2.0). Error bars represent SD from three independent experiments. An asterisk (*) indicates a statistically significant difference (Student’s t-test, P < 0.01).
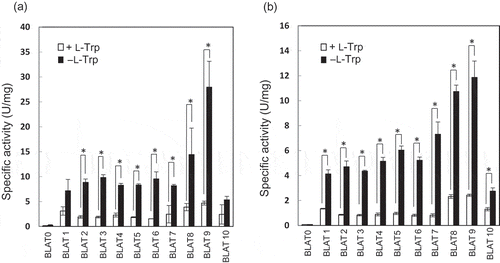
SDS-PAGE analysis of the proteins of BLAT9 showed that the production of the reporter protein was 75 and 33 mg/L-culture at 18°C and 4°C, respectively ()), and was comparable to that achieved by using the LI3 promoter, which was previously developed for low-temperature overproduction of foreign proteins in S. livingstonensis Ac10 [Citation7]. By supplementation with L-Trp, the production of this protein was significantly decreased both at 18°C and 4°C ()). Real-time RT-PCR analysis demonstrated that, in the presence of L-Trp, the expression of bla was suppressed to about 15% and 30% of that without L-Trp supplementation at 18°C and 4°C, respectively ()). These results suggest that the T9 promoter is useful for regulatable heterologous protein production at low temperatures.
Figure 5. Expression of bla in recombinant S. livingstonensis Ac10-Rifr harboring pT9bla (BLAT9) .
(a, b) SDS-PAGE analysis of proteins of BLAT9 grown with or without L-Trp at 18°C (a) and 4°C (b). Arrowheads indicate the position of the BLA protein band. M: protein marker; Cont.: BLAT0 grown without L-Trp. (c, d) Relative amounts of bla mRNA in BLAT9 grown with 0.1% L-Trp in comparison with that in the cells grown without L-Trp. The cells were grown at 18°C (c) and 4°C (d). All values were normalized to the amounts of 16S rRNA. Error bars represent SD from three independent experiments.
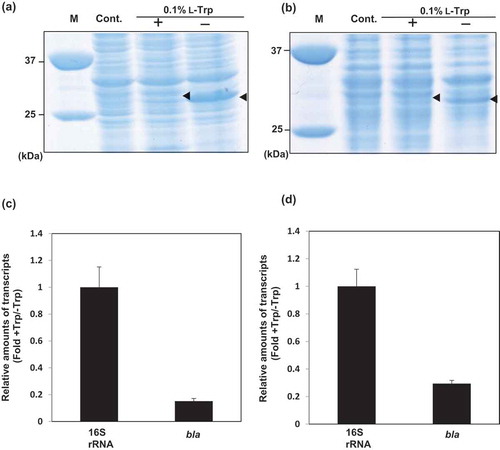
Regulation of heterologous gene expression using the T9 promoter
We examined the validity of the T9 promoter in regulation of heterologous gene expression under various conditions. First, we examined whether the expression level can be modulated by L-Trp in a short incubation period after exchanging the culture medium. BLAT9 cells were grown in the basal medium without L-Trp supplementation until the log, late-log, and early stationary phases at 18°C or 4°C, and the whole cells obtained were transferred to the basal medium with or without 0.1% L-Trp supplementation. After a 2 h incubation at 18°C or 4°C, cell extracts were prepared, and BLA activities were measured. When the cells grown to the late-log phase were transferred to the medium supplemented with 0.1% L-Trp, both total activity and specific activity were suppressed to less than 50% of those transferred to the medium without L-Trp supplementation at 18°C (Figure S1(a,b)). At 18°C, a significant suppressive effect was also observed for the cells grown to the log phase. Suppression of the BLA activity by L-Trp supplementation was also observed for the cells cultivated at 4°C (Figure S1(c,d)). Real-time RT-PCR analysis of bla mRNA in the cells incubated in the presence or absence of L-Trp supplementation demonstrated that transcription of the gene was significantly decreased in the presence of L-Trp (Figure S1(e)), indicating that production of BLA is regulated at the transcriptional level. Thus, expression of genes under the control of the T9 promoter can be suppressed by incubation with L-Trp for a short period at low temperatures after exchanging the culture medium. Since BLA was detected as one of the major protein bands in the cells incubated without L-Trp supplementation (Figure S1(f)), the T9 promoter can be considered as a powerful and regulatable promoter useful for production of heterologous proteins in S. livingstonensis Ac10.
We also performed a similar experiment by using the BLAT9 cells pre-cultivated in the basal medium supplemented with L-Trp to suppress expression of the heterologous protein. The cells grown to the late-log phase at 18°C or 4°C were transferred to the basal medium with or without 0.1% L-Trp supplementation and incubated for 2 h at the respective temperature. In this case, the total activity of BLA was about 3.7 and 1.9 times higher when the cells were incubated in the absence of L-Trp than in the presence of L-Trp at 18°C and 4°C, respectively (Figure S2(a,c)). The specific activity was about 3.2 and 2.4 times higher when the cells were incubated in the absence of L-Trp at 18°C and 4°C, respectively (Figure S2(b,d)). Induction of expression of heterologous proteins by incubation in the absence of L-Trp after obtaining a large amount of the cells by pre-cultivation in the presence of L-Trp would be useful for production of toxic proteins of which production inhibits the growth of the host cells.
We next examined the effects of 3-IAA, which is used as a gratuitous inducer of the trp promoter of E. coli [Citation16], on expression of genes under the control of the T9 promoter. For this experiment, LB medium was used instead of the synthetic minimal medium. The cells grown in LB medium to the log (OD600 = 0.7–0.8), late-log (OD600 = 0.8–1.0), and stationary (OD600 = 1.8–2.0) phases at 18°C and 4ºC were transferred to LB medium containing 0.1% 3-IAA and incubated for 2 h ()). As a control, cells were transferred to LB medium without 3-IAA. The highest total activity and specific activity were observed when the cells grown to log phase were transferred to the 3-IAA-containing medium both at 18°C and 4°C. The total activity in the 3-IAA-containing medium was 3.2 and 3.3 times higher than that in the medium without 3-IAA at 18°C and 4°C, respectively. The specific activity in the 3-IAA-containing medium was 2.6 and 3.8 times higher than that in the medium without 3-IAA at 18°C and 4°C, respectively. Thus, 3-IAA is expected to be useful for inducible expression of heterologous genes under the control of the T9 promoter.
Figure 6. Effects of 3-indoleacrylic acid (3-IAA) on expression of the gene under the control of the T9 promoter.
Total activity (a and c) and specific activity (b and d) of BLA from a 5 mL culture of BLAT9. The cells grown at 18°C (a and b) and 4°C (c and d) to the log (OD600 = 0.7–0.8), late-log (OD600 = 0.8–1.0), and stationary (OD600 = 1.8–2.0) phases were transferred to LB medium with (open bars) or without (closed bars) 0.1% 3-IAA supplementation. As a control, BLAT0 harboring pbla, which contains the BLA gene without a promoter, was used. Error bars represent SD values from three independent experiments. Asterisks (*) indicate statistically significant differences (Student’s t-test, P < 0.01).
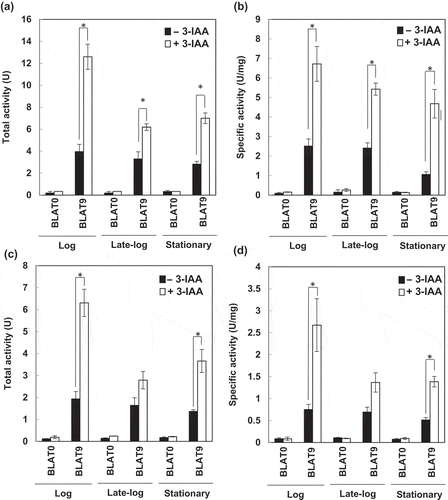
Through this study, we succeeded in applying a promoter of the Trp-biosynthesis operon, named the T9 promoter, of S. livingstonensis Ac10 to regulatable gene expression at low temperatures. In the absence of L-Trp, 75 mg/L and 33 mg/L cultures of BLA were produced as one of the major proteins at 18°C and 4°C, respectively. These yields are comparable to those obtained using the LI3 promoter, which was previously developed for overproduction of foreign proteins in S. livingstonensis Ac10 [Citation7]. We also demonstrated that gene expression under the control of the T9 promoter can be regulated by 3-IAA supplementation. Thus, the T9 promoter is expected to be useful for the production of various proteins including toxic proteins whose production should be suppressed during pre-cultivation to obtain a large enough number of cells for their production. Finding of the T9 promoter as a new regulatable promoter could expand the utility of the low-temperature protein expression systems with S. livingstonensis Ac10 as the host.
Author contribution
S.K., J.K., and T.K. designed the study. S.K. performed the experiments. S.K., J.K., and T.K. discussed the results. S.K., J.K., T.O., and T.K. wrote the paper.
Kawai_BBB_FigureS2_final.pdf
Download PDF (72.2 KB)Kawai_BBB_FigureS1_final.pdf
Download PDF (146.3 KB)Kawai_BBB_TableS1_final.pdf
Download PDF (46.5 KB)Disclosure statement
No potential conflict of interest was reported by the authors.
Supplementary material
Supplemental data for this article can be accessed here.
Additional information
Funding
References
- Sørensen HP, Mortensen KK. Soluble expression of recombinant proteins in the cytoplasm of Escherichia coli. Microb Cell Fact. 2005;4:1–8.
- Rasala BA, Mayfield SP. Photosynthetic biomanufacturing in green algae; production of recombinant proteins for industrial, nutritional, and medical uses. Photosynth Res. 2015;123(3):227–239.
- Ritacco FV, Wu Y, Khetan A. Cell culture media for recombinant protein expression in Chinese hamster ovary (CHO) cells: history, key components, and optimization strategies. Biotechnol Prog. 2018;34(6):1407–1426.
- Gerday C, Aittaleb M, Bentahir M, et al. Cold-adapted enzymes: from fundamentals to biotechnology. Trends Biotechnol. 2000;18(3):103–107.
- Cronan JE. Improved plasmid-based system for fully regulated off-to-on gene expression in Escherichia coli: application to production of toxic proteins. Plasmid. 2013;69(1):81–89.
- Mujacic M, Cooper KW, Baneyx F. Cold-inducible cloning vectors for low-temperature protein expression in Escherichia coli: application to the production of a toxic and proteolytically sensitive fusion protein. Gene. 1999;238(2):325–332.
- Miyake R, Kawamoto J, Wei YL, et al. Construction of a low-temperature protein expression system using a cold-adapted bacterium, Shewanella sp. strain Ac10, as the host. Appl Environ Microbiol. 2007;73(15):4849–4856.
- Sannino F, Giuliani M, Salvatore U, et al. A novel synthetic medium and expression system for subzero growth and recombinant protein production in Pseudoalteromonas haloplanktis TAC125. Appl Microbiol Biotechnol. 2017;101(2):725–734.
- Kawamoto J, Kurihara T, Kitagawa M, et al. Proteomic studies of an Antarctic cold-adapted bacterium, Shewanella livingstonensis Ac10, for global identification of cold-inducible proteins. Extremophiles. 2007;11:819–826.
- Rabus R, Ruepp A, Frickey T, et al. The genome of Desulfotalea psychrophila, a sulfate-reducing bacterium from permanently cold Arctic sediments. Environ Microbiol. 2004;6(9):887–902.
- Papa R, Glagla S, Danchin A, et al. Proteomic identification of a two-component regulatory system in Pseudoalteromonas haloplanktis TAC125. Extremophiles. 2006;10(6):483–491.
- Davison J, Heusterspreute M, Chevalier N, et al. Vectors with restriction site banks. V. pJRD215, a wide-host-range cosmid vector with multiple cloning sites. Gene. 1987;51(2,3):275–280.
- Toyotake Y, Cho HN, Kawamoto J, et al. A novel 1-acyl-sn-glycerol-3-phosphate O-acyltransferase homolog for the synthesis of membrane phospholipids with a branched-chain fatty acyl group in Shewanella livingstonensis Ac10. Biochem Biophys Res Commun. 2018;500(3):704–709.
- Hanahan D, Jessee J, Bloom FR. Plasmid transformation of Escherichia coli and other bacteria. Methods Enzymol. 1991;204:63–113.
- Simon R, Priefer U, Pühler A, et al. A broad host range mobilization system for in vivo genetic engineering: transposon mutagenesis in gram negative bacteria. Bio Technol. 1983;1:784–791.
- Somerville RL. The trp promoter of Escherichia coli and its exploitation in the design of efficient protein production systems. Biotechnol Genet Eng Rev. 1988;6:1–41.
- Liubetskaia EV, Leont’ev LA, Gel’fand MS, et al. Search for alternative secondary structures of RNA, regulating expression of bacterial genes. Mol Biol. 2003;37(5):834–842.
- Das A, Crawford IP, Yanofsky C. Regulation of tryptophan operon expression by attenuation in cell-free extracts of Escherichia coli. J Biol Chem. 1982;257(15):8795–8798.
- Kelley RL, Yanofsky C. Trp aporepressor production is controlled by autogenous regulation and inefficient translation. Proc Natl Acad Sci U S A. 1982;79(10):3120–3124.
- Jeeves M, Evans PD, Parslow RA, et al. Studies of the Escherichia coli Trp repressor binding to its five operators and to variant operator sequences. Eur J Biochem. 1999;265(3):919–928.
- Arvidson DN, Grove Arvidson C, Lawson CL, et al. The tryptophan repressor sequence is highly conserved among the Enterobacteriaceae. Nucleic Acids Res. 1994;22(10):1821–1829.
- Palego L, Betti L, Rossi A, et al. Tryptophan biochemistry: structural, nutritional, metabolic, and medical aspects in humans. J Amino Acids. 2016;2016:1–13.
- Abbas SH, Sohail M, Saleem M, et al. Effect of L-tryptophan on plant weight and pod weight in chickpea under rainfed conditions. Sci Tech Dev. 2013;32(4):277–280.
- Xie Y, Reeve JN. Regulation of tryptophan operon expression in the archaeon Methanothermobacter thermautotrophicus. J Bacteriol. 2005;187(18):6419–6429.
- Bertrand K, Yanofsky C. Regulation of transcription termination in the leader region of the tryptophan operon of Escherichia coli involves tryptophan or its metabolic product. J Mol Biol. 1976;103(2):339–349.
- Hook-Barnard IG, Hinton DM. Transcription initiation by mix and match elements: flexibility for polymerase binding to bacterial promoters. Gene Regul Syst Biol. 2007;1:275–293.
- Mironov AA, Koonin EV, Roytberg MA, et al. Computer analysis of transcription regulatory patterns in completely sequenced bacterial genomes. Nucleic Acids Res. 1999;27(14):2981–2989.