ABSTRACT
The protein and oil contents in soybean seeds are major factors in seed quality. Seed proteins and oils are synthesized from sucrose and nitrogenous compounds transported into maturing seeds. In this study, we compared changes in the activity of phosphoenolpyruvate carboxylase (PEPC) and the accumulation profiles of protein and oil in maturing seeds of two soybean cultivars, which exhibit different protein and oil contents in seeds, to determine the interrelationships of them. A principal component analysis indicated a concordance of seed PEPC activity with the protein content, but did not with the oil content. PEPC activity per seed was highest in the late maturation stage, when the physiological status of the vegetative organs drastically changed. The high-protein cultivar had higher PEPC activity compared to the low-protein cultivar. These results highlight the biological role of PEPC in the synthesis of protein, therefore it was implied that PEPC could be a biomarker in soybean breeding.
Abbreviations: ANOVA: analysis of variance; DS: developmental stage; DW: dry weight; FW: fresh weight; NIR: near infrared; PEP(C): phosphoenolpyruvate (carboxylase); PC(A): principal component (analysis); S.E.: standard error; WC: water content.
Graphical abstract
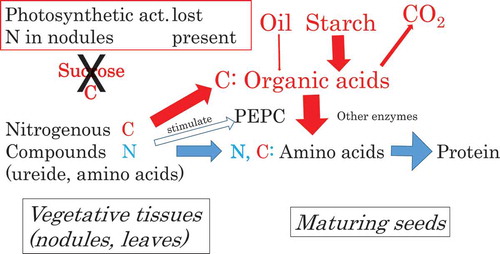
Soy seed PEPC play a role for providing carbon skelton of amino acids of storage protein from starch in responce to N supply at the late maturing stage.
Soybean seeds contain approx. 40% protein, 20% oil, and 35% carbohydrate [Citation1]. Soybean seeds have the highest protein content among crops grown for foodstuffs and feeds. Further genetic improvement of seed protein content is a critical challenge for development of better seed crops, and it requires understanding the biological basis of how seed protein content is determined. It has been thought as a possible key that seed protein content was often inversely correlated with seed oil content among soybean cultivars (CVs) [Citation2–Citation4].
The enzyme phosphoenolpyruvate carboxylase (PEPC, EC 4.1.1.31) catalyzes the β-carboxylation of phosphoenolpyruvate (PEP) to produce oxaloacetate and phosphate. Maturing plant seeds have high PEPC activity of 1.0–1.4 U g–1 fresh weight at maximum [Citation5,Citation6]. A possible role of PEPC in plant seeds is the provision of carbon skeletons used in amino acid biosynthesis, fatty acid biosynthesis, and respiration [Citation7–Citation9]. We observed a high correlation of PEPC activity and protein content in mature seeds among 11 cultivars of soybean [Citation10]. Since Escherichia coli PEPC plays a role in the replenishment of the tricarboxylic acid cycle intermediates, which is called an “anaplerotic reaction”, PEPC could support amino acid biosynthesis [Citation11]. Aside, PEP can be transported to plastids and converted into pyruvate, which is used for fatty acid biosynthesis [Citation12,Citation13]. The organic acid metabolism pathway including PEPC may be a branch point of amino acid and fatty acid biosynthesis.
In the present study, we chose two soybean CVs with different seed protein contents: a high-protein CV, Enrei (EN), and a low-protein CV, Tamahomare (TA), to compare the developmental patterns of PEPC activity with the accumulation of protein and oil in seeds by performing a principal component analysis (PCA). This study present primarily evaluation of the biological role of PEPC and a potential that PEPC can be a biomarker in soybean breeding and agriculture.
Materials and methods
Plant materials
Plants of the two soybean CVs, EN and TA, were grown in a field at Kobe University (34°43′ 27″N, 135°13′ 58″E) during the summer season of 2014. Immature seeds of EN were harvested on 20th Aug. and 1st, 11th, 16th, and 25th Sept. to cover almost all the seed maturation stages. Immature seeds of TA were harvested on 11th, 16th, and 25th Sept. and 4th and 14th Oct. to obtain immature seeds corresponding to the same developmental stages as the EN seeds. Pods with seeds were frozen in liquid nitrogen immediately after harvest and stored at −80°C until use. These seeds were subdivided into different weight ranges as described in the next paragraph. Three or four randomly selected seeds sampled at each stage were individually weighed and lyophilized. Frozen dried seeds were weighed. Mature seeds were collected at the end of Sept. for EN and mid-Oct. for TA and air-dried in a warehouse set in the field for the analysis of seed composition using near-infrared reflectance (NIR) spectroscopy.
In this study, we defined seven developmental stages (DSs) of seeds (Supplemental Figure 1). The correspondence of the harvested dates of seeds and the seed fractions based on their fresh weights (FW) (Supplemental Table 1). Seed weight ranges were 100 ~ 250 mg for DS1, 250 ~ 400 mg for DS2, 400 ~ 600 mg for DS3, 600 ~ 700 mg for DS4, 700 ~ 900 mg for DS5, 900 ~ 600 mg for DS6, and 600 ~ 300 mg for DS7. DS5 seeds were the largest among all the stages. The desiccation of the seeds was observed at DS6.
Figure 1. Changes in PEPC activity and accumulation of protein and oil in seeds of two cultivars, Enrei and Tamahomare, during seed maturation. A: amount of proteins per seed, B: PEPC activity per seed. Black and white circles indicate the cultivar, Enrei and Tamahomare, respectively. Error bar represents standard error (n = 3).
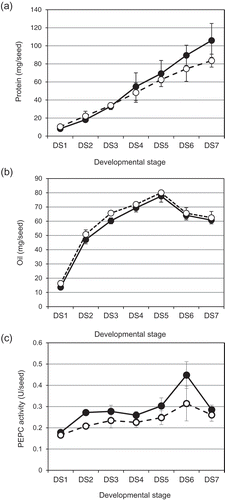
Measurements of chemical composition of mature seeds
Protein, oil, carbohydrate, and water content (WC) in mature seeds were determined by an Infratec 1241 grain analyzer (Foss, Hillerød, Denmark). The protein amount was determined from the nitrogen amount using the conversion factor of 5.71 [Citation14]. The contents of the three seed components, i.e. protein, oil and carbohydrate, were calculated on a water-free basis.
Chemical analysis of protein and oil in immature seeds
Frozen immature seeds were homogenized with 2:1 of chloroform/methanol to extract lipid (crude oil) for the estimation of oil content. After the homogenates were centrifuged, the chloroform/methanol solvent was collected and the solvent was evaporated with the use of a vacuum centrifuge concentrator for weighing the residue as crude oil. Protein amounts in the defatted extracts of maturing seeds were determined by the method described by Sasou et al. [Citation15] using an SDS-sample buffer of Laemli method as an extractant.
PEPC activity measurement of immature seeds
For the assay of PEPC activity, a crude protein fraction was prepared from immature seeds at 4°C. The PEPC protein was extracted in a buffer [100 mM Tris–HCl, pH 7.8, 1 mM EDTA, 1 mM 2-mercaptoethanol, 10% (w/v) glycerol, 25 mM NaF, and 5 mM NaPPi] with Complete Protease Inhibitor Cocktail (Hoffmann-La Roche, Basel, Switzerland) at six times the amount of the sample fresh weight with a mortar and pestle. The homogenate was centrifuged at 13,000 g for 30 min, and the resulting supernatant was the crude protein fraction. Measurement of PEPC activities in the maturing seeds was done by the method of Yamamoto et al. [Citation16].
Statistical analysis and principal component analysis
We used Student’s t-test to determine the statistical significance of differences in each type of data between the two CVs. We used the function ‘prcomp‘ of the R statistical package “stats” (https://www.r-project.org/) with the option ‘scale = TRUE‘ for the PCA to test the data regarding PEPC activity, protein and oil contents, and the seed weights of immature seeds. The results were visualized using the factoextra R package.
Results
Profiles of protein and oil accumulations and the growth of immature seeds from the Enrei and Tamahomare plants
Maturing seeds from the EN and TA plants continued to increase their FW until DS5, and then started to dehydrate and lose their green color (Supplemental Figure 1). We monitored the increases in the amounts of protein and oil per seed in immature seeds from each CV. The accumulation patterns of protein in a seed of both CVs were rectilinear, and the patterns were similar to each other until DS5. At DS6 and DS7, the EN seeds showed a greater increase in the amount of protein per seed compared to in the TA seeds (). In contrast, the amounts of oil per seed did not show such rectilinear patterns; they showed upwardly convex increases during stages DS1–DS3, and the increases during stages DS3-DS7 in the amount of oil per seed were slight (). In addition, the two CVs did not exhibited any clear difference in the amount of oil per seed during maturation.
As expected, the mature EN seeds showed a significantly higher seed protein content than TA (p < 0.01) based on the relative content per DW and the protein amount per seed (Supplemental Table 2). The amount of protein per seed in the EN seeds was 27.5% higher than that in the TA seeds (p < 0.01). The relative contents of oil and carbohydrate were also significantly different between the CVs (p < 0.05), but there was no significant difference in the amounts of oil and carbohydrate per seed between the two CVs. The amount of oil per seed in EN was lower than that in TA, but not statistically significant.
Comparison of PEPC activities in maturing seeds between Enrei and Tamahomare plants
We monitored the transitional changes of PEPC activity in seeds during maturation on three different bases: per seed, FW, and dry weight (DW). PEPC activity per seed showed an increasing trend until DS6 in both CVs (). PEPC activity was significantly higher in the EN seeds than the TA seeds throughout seed maturation (p < 0.01, two-way analysis of variance [ANOVA]). PEPC activity per FW also showed a trend of higher activity in EN than TA (Supplemental Figure 2A), but it was not constant through seed maturation for both CVs: PEPC activity was high at DS1 and DS2, decreased until DS5, and then increased at DS6. PEPC activity per DW was also higher in EN than TA (Supplemental Figure 2B), but the trend of the developmental patterns differed from that of the PEPC activity per seed. Namely, PEPC activities per FW and DW of both CVs had a downward trend until DS5, but EN had increased PEPC activity during the stages DS6-DS7, and TA did not exhibit clear up-regulation during stages DS5-DS7. Notably, PEPC activities on both the FW and DW bases at DS3 and DS5 were similar between the two CVs. The PEPC activity per FW for EN was kept at a high level during stages DS6-DS7, but the PEPC activity per DW was decreased at DS7. TA exhibited an up-regulation of PEPC activity per FW after DS5, but it was lower than that of EN (Supplemental Figure 2A).
Pattern analysis by PCA
In order to find interrelationships among PEPC activity, proteins, oil, and seed FWs in the two CVs, we performed a PCA analysis. The two principal components PC1 and PC2 explained 90.0% of the total variance. Based on the distribution of samples in the PCA biplot and contributions of variables for these two major principal components (), PC1 seemed to explain the seed development status, and PC2 seemed to explain variables in the seed components, of which the major factor was PEPC activity. Here, the PCA biplot indicated associations between seed FW and oil, and protein and PEPC activity, respectively (). The Pearson’s correlation coefficients between the oil contents in the seeds and the PEPC activity was 0.523 (not significant) but the Pearson’s correlation coefficients between the protein contents in the seeds and the PEPC activity was 0.690 (p value < 0.01). The association between oil and FW was also supported by a high Pearson’s correlation coefficient (0.902, p value < 0.01). Since EN and TA seeds at DS6 were distantly located in the PCA biplot, the data of DS6 is likely to contribute on these associations. In fact, a PCA without the DS6 data did not show such clear specific associations on a biplot, verifying the importance of DS6 (data not shown).
Figure 2. The results of PCA in seed compositions, protein, oil, seed weight and PEPC activities during seed maturation. Characters and numerals show the name of cultivars and stage of samples, respectively. The characters EN and TA indicate Enrei and Tamahomare, respectively. The numerals with DS indicate the stage of samples. A: a biplot of the two major principal components, representing a distribution of the samples analyzed. Marks in black indicate samples, and arrows indicate the directions of protein content (Protein), oil content (Oil), seed fresh weight (SW), and PEPC activity per seed (PEPC). Color of arrows indicate contributions of variances (%). Horizontal- and vertical- axes represent principal component 1 (PC1) and principal component 2 (PC2), respectively. PC1 and PC2 explain 73.6 and 16.4% of the data variances, respectively. B and C: contributions of the variables on PC1 and PC2, respectively. Dot lines indicate the averaged values.
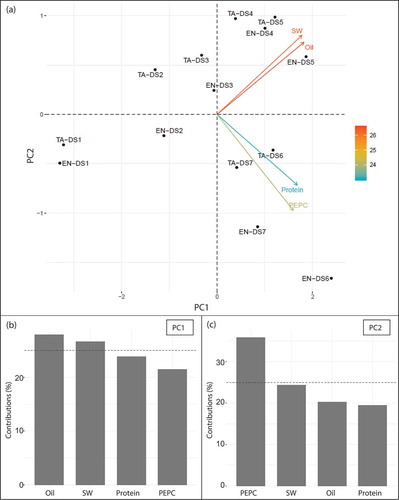
Discussion
Soybean plants represent a biologically significant change during the late maturation period: yellowing of leaves, which indicates the loss of the leaves’ photosynthetic activity. As a result, both the N2 fixation by nodules and the photosynthate (namely sugar) supply to maturing seeds cease at the late stage of maturation. Bender et al. noted that the nitrogen uptake in the seeds was still active at the late maturation stage [Citation17]. Therefore, developing seeds receive nitrogenous compounds from senescent nodules and leaves but not carbon from leaves at the late maturing stage. Another possible source of carbon is starch stored temporarily in immature seeds [Citation1]. Monma et al. reported that in the CV EN, (1) starch granules observed in immature seeds disappeared in mature seeds, and (2) starch content in matured seeds was < 1% of the highest value of that in the immature seeds [Citation18]. Our present and previous observations [Citation19] showed that in the case of EN, the oil accumulation ceased before the late maturing stage of the seeds but the protein accumulation continued until the end of ripening. Based on these observations we suggest that in the CV EN, PEPC plays a role in the anaplerotic reaction by changing organic acids to carbon skeletons of amino acids from digested starch in maturing seeds.
It was reported that the activity of PEPC in maturing seeds of wheat [Citation6,Citation20] and rice [Citation21] increased along with the increased supply of fertilized N at the flowering stage, which suggested both that PEPC in maturing crop seeds has a role in the synthesis of protein and that maturing EN seeds receive higher amounts of nitrogenous compounds from vegetative tissue compared to maturing TA seeds. We also observed in a previous investigation that plants of eight of 13 CVs showed a decrease in protein content and an increase in oil content in seeds when we prevented nodule formation by the application of a high amount of a short-lived slow-release nitrogen fertilizer [Citation22]. We also observed that the seeds’ PEPC activity was proportional to the leaf N concentration [Citation22]. These observations indicate an important role of PEPC in maturing seeds: supplement of organic acids for amino acid synthesis.
Crop seeds including soybean typically increase their FW and DW at the early maturation stage and decrease their FW by dehydration from middle to maturation stages. While developmental patterns of seed PEPC activity are diverse among crop species [Citation5,Citation23–Citation25]. Our results showed that PEPC activity in EN and TA were differently regulated in developing seeds (, Supplemental Figure 2). To assess the role of this enzyme, we opted to use total PEPC activity per seed as a primary indicator, but neither weight-based PEPC activity (U/FW or U/DW) nor the specific PEPC activity (U/mg soluble protein). These two parameters are apparently biased by seed storage protein accumulated in developing seeds and differences in seed FW between EN and TA at DS5. Therefore, we used total PEPC activity per seeds for biological evaluation between PEPC activity and seed protein. A PCA using DW-based PEPC activity at DS6 to DS7 supported that PEPC activity was associated with seed protein (data not shown).
In summary, a PCA showed that the PEPC activity and protein content in soybean immature seeds are correlated with each other. This result suggests that PEPC supplies organic acids for the synthesis of storage protein in maturing soybean seeds. More rapid biosynthesis of protein at the late maturation period seemed to increase the protein content of EN seeds. Our results imply that soybean seed PEPC during desiccation can be applicable as a biomarker for selecting breeding materials or diagnosis of agricultural conditions. These concepts remain to be proved by an examination using a larger number of cultivars.
Author contributions
NY designed experiments, collected maturing seeds and measured the enzyme activities and amounts of storage compounds in maturing seeds, TM measured contents of matured seeds by NIR, KY designed experiments, TS cultivated soybean plants and prepared the manuscript.
Figshare_yamamoto.pdf
Download PDF (966 KB)Disclosure statement
No potential conflict of interest was reported by the authors.
Supplementary material
Supplemental data for this article can be accessed here.
Additional information
Funding
References
- Liu K. Soybeans: chemistry, technology, and utilization. In: Proceedings of the Crop Science Society of Japan. New York (NY): Chapman Hall; 1997. p. 25–35.
- Taira H, Taira H. Influence of location on the chemical composition of soybean seeds. I. Protein, oil, carbohydrate, and ash contents. Jpn J Crop Sci. 1971;40:530–544. Japanese.
- Hymowitz T, Collins FI, Panczner J, et al. Relationship between the content of oil, protein, and sugar in soybean seed. Agron J. 1972;64:613–616.
- Li W, Sun D, Du Y, et al. Quantitative trait loci underlying the development of seed composition in soybean (Glycine max L. Merr.). Genome. 2007;50:1067–1077.
- Yamamoto N, Shimada M, Sugimoto T, et al. Dynamic protein expression of phosphoenolpyruvate carboxylase in developing rice seeds. J Cereal Sci. 2014;60:457–460.
- Yamamoto N, Kinoshita Y, Sugimoto T, et al. Role of nitrogen-responsive plant-type phosphoenolpyruvate carboxylase in the accumulation of seed storage protein in ancient wheat (spelt and kamut). Soil Sci Plant Nutr. 2017;63:23–28.
- Plaxton WC, Podestá EF. The functional organization and control of plant respiration. CRC Crit Rev Plant Sci. 2006;25:159–198.
- Andre C, Froehlich JE, Moll MR, et al. Heteromeric plastidic pyruvate kinase complex involved in seed oil biosynthesis in Arabidopsis. Plant Cell. 2007;19:2006–2022.
- Junker BN, Lonien J, Heady LE, et al. Parallel determination of enzyme activities and in vivo fluxes in Brassica napus embryos grown on organic or inorganic nitrogen source. Phytochem. 2007;68:2232–2242.
- Sugimoto T, Tanaka K, Monma M,Y, et al. Phosphoenolpyruvate carboxylase level in soybean seed highly correlates to its contents of protein and lipid. Agric Biol Chem. 1989;53:885–887.
- Ashworth JM, Kornberg HL. The anaplerotic fixation of carbon dioxide by Escherichia coli. Proc R Soc Lond B Biol Sci. 1966;165:179–188.
- O’Leary B, Park J, Plaxton WC. The remarkable diversity of plant PEPC (phosphoenolpyruvate carboxylase): recent insights into the physiological functions and post-translational controls of non-photosynthetic PEPCs. Biochem J. 2011;436:15–34.
- Yamamoto N, Sasou A, Saito Y, et al. Protein and gene expression characteristics of a rice phosphoenolpyruvate carboxylase Osppc3; its unique role for seed cell maturation. J Cereal Sci. 2015;64:100–108.
- Tkachuk R. Nitrogen to protein conversion factors for cereals and oilseed meals. Cereal Chem. 1969;46:419–423.
- Sasou A, Shigemitsu T, Saito Y, et al. Control of foreign polypeptide localization in specific layers of protein body type I in rice seed. Plant Cell Rep. 2016;35:1287–1295.
- Yamamoto N, Kubota T, Masumura T, et al. Molecular cloning, gene expression and functional expression of a phosphoenolpyruvate carboxylase Osppc1 in developing rice seeds: implication of involvement in nitrogen accumulation. Seed Sci Res. 2014;24:23–36.
- Bender RR, Haegele JW, Below FE. Nutrient uptake, partitioning, and remobilization in modern soybean varieties. Agron J. 2015;107:563–573.
- Monma M, Sugimoto T, Monma M, et al. Starch breakdown in developing soybean seeds (Glycine max cv. Enrei). Agric Biol Chem. 1991;55:67–71.
- Sugimoto T, Nomura K, Masuda R, et al. Effect of nitrogen application at the flowering stage on the quality of soybean seeds. J Plant Nutr. 1998;21:2065–2075.
- Yamamoto N, Sugimoto T, Masumura T. Concomitant increases of the developing seed phosphoenolpyruvate carboxylase activity and the seed protein content of field-grown wheat with nitrogen supply. Agric Sci. 2014;5:1558–1565.
- Sugimoto T, Sueyoshi K, Oji Y. Increase of PEPC activity in developing rice seeds with nitrogen application at flowering stage. Dev Plant Soil Sci. 1997;78:811–812.
- Sugimoto T, Shiraishi N, Oji Y. Changes in contents of storage compounds in soybean seeds by application of nitrogen fertilizers. In: Ohyama T, editor. Improvement of production and quality of soybean in relation to plant nutrition and physiology. Tokyo: Hakuyusha; 2005. p. 40–58. Japanese.
- Sangwan RS, Singh N, Plaxton WC. Phosphoenolpyruvate carboxylase activity and concentration in the endosperm of developing and germinating castor oil seeds. Plant Physiol. 1992;99:445–449.
- Feria AB, Alvarez R, Cochereau L, et al. Regulation of phosphoenolpyruvate carboxylase phosphorylation by metabolites and abscisic acid during the development and germination of barley seeds. Plant Physiol. 2008;148:761–774.
- Ruiz-Ballesta I, Baena G, Gandullo J, et al. New insights into the post-translational modification of multiple phosphoenolpyruvate carboxylase isoenzymes by phosphorylation and monoubiquitination during sorghum seed development and germination. J Exp Bot. 2016;67:3523–3536.