ABSTRACT
Electronic cigarette(s) (EC) becoming a preferred replacement for nicotine delivery among many smokers in recent years. However, the effect of EC on human health is inconclusive due to a lack of empirical research investigating EC-induced health hazard or benefit. In this study, we examine the effect of vapor produced by EC on exercise performance and health-related profiles in a mouse model. Female ICR mice were divided into five groups (n = 6 per group) and exposed for 14 days. Our results indicate that EC exposure leads to dose-dependent decrease in the grip strength and swimming time of the mice. The EC-treated groups also showed a dose-dependent decrease in liver and muscle glycogen storage. In addition, EC treatment had no negative effect on levels of biochemical indices. We also did not detect any adverse effect or gross abnormalities on the morphology of the major organs.
Graphical abstract
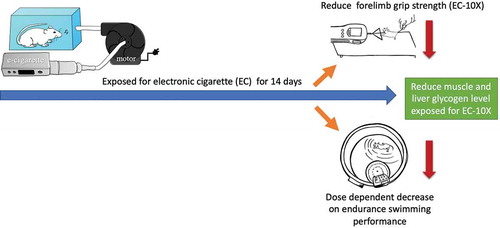
EC exposure leads to dose-dependent decrease in exercise performance (endurance and explosiveness) and glycogen storage capacity of the mice.
Electronic cigarette(s) (EC), also referred to as vaporizers, have been promoted to help with abstinence from cigarette [Citation1–Citation5] and are readily available in many countries [Citation6,Citation7]. Although ECs are forbidden in Taiwan, they are commercially readily available through online shopping, shopping malls and local shops in the United States. An EC comprises a Temperature Control System (TCR) and an atomizer with a tank to refill liquid containing flavoring, a humectant (such as propylene glycol or vegetable glycerin) and nicotine. TCR is battery-operated and upon activation, the heating element/atomizer heats up the liquid to make vapor or aerosol [Citation8]. The health-related ramifications of EC have not been well researched and there is a need for more evidence to support EC as an effective and safe cigarette substitute. Some studies have highlighted the toxicity concerns of EC [Citation1,Citation2], while others acknowledge that although EC are addictive and may not be completely safe, are still considered less harmful than conventional cigarettes [Citation9].
Cigarettes are the most common source of nicotine. Although nicotine could induce stimulation of the sympathetic nervous system leading to increased heart rate, blood pressure and muscle blood flow [Citation10,Citation11], the question of whether nicotine directly enhances exercise performance still remains partially answered. On one hand, some research have shown that nicotine patch application could increase exercise performance [Citation12,Citation13], but on the other hand, conventional cigarettes smoker may increase exercise fatigue and decrease average performance [Citation14] due to greater dependency on the use of glucose as a fuel source during submaximal steady-state exercise [Citation15]. Thus, research on EC is urgently needed in order to ensure that the decisions of regulators, health-care providers, and consumers are based on science. In general, the health effects of EC have not been well studied and the potential harm of exercise performance incurred by short-term use of these devices remains completely unknown. On the other hand, smokeless tobacco that is sniffed through the nose or placed between the cheek and gum. Same as EC, smokeless tobacco is a gateway to induce addiction. Furthermore, EC and smokeless tobacco have deleterious effects on human health, including exercise performance. Therefore, we conducted this study to evaluate the exercise performance and health-related profiles of EC treatment using our previously established in vivo platform [Citation16,Citation17]. Few reports have addressed the effect of EC intervention on exercise performance and health-related biochemical index. We aim to evaluate the effect of EC after 14 days of intervention in mice and examine the subchronic toxic effects of the different dose of EC for exercise performance and basic health parameters.
Materials and methods
Preparation of electronic cigarette (EC) exposure equipment
EC devices used in this study consist of two parts: a temperature control system (TCR; Joyetech Co., Ltd., China) and a vaporizer (Geek Vape, MI, USA.) (). Vanilla-flavored refillable E-liquid was purchased from NAKED FISH® (CA, USA). The concentration of E-liquid was adjusted with vegetable glycerin, odorless liquids and flavor additives. The EC vapor was produced and pumped using an electric air pump (0.5 ohm, 50 watt, and 4.7 V, Lisan Co., Ltd., Taiwan) into a chamber (20x15x15 cm3). Each mouse stayed in the vapor-exposed chamber until the E-liquid, which was pre-loaded in the vaporizer tank, was exhausted (4 mL) ().
Materials, animals, and experiment design
Female ICR mice (8-weeks old) grown under specific pathogen-free conditions were purchased from BioLASCO (Yi-Lan, Taiwan). All mice were provided a standard laboratory diet (No. 5001; PMI Nutrition International, Brentwood, MO, USA), distilled water ad libitum and housed at 12-h light/12-h dark cycle at room temperature (22°C ± 1°C) and 50–60% humidity. The Institutional Animal Care and Use Committee (IACUC) of National Taiwan Sport University (NTSU) inspected all animal experiments and the study conformed to the guidelines of protocol IACUC-10519 approved by the IACUC ethics committee. Thirty mice were randomly assigned to five groups (6 mice/group): (1) vehicle; (2) air; (3) EC-0X; (4) EC-1X; and (5) EC-10X. The EC-0X (0 mg/day) mouse group used the E-liquid without nicotine. The EC-1X mice were given E-liquid with 4 mL vegetable glycerin and 0.5 mg/mL nicotine final concentration. The EC-10X mouse had 4 mL vegetable glycerin with 5 mg/mL nicotine final concentration. Mice were exposed daily to the chamber with vapor pumped in by an electric air pump with the cigarette (contain E-liquid 4 mL) per day that was pre-loaded in the vaporizer tank. The vehicle group received the same volume in the chamber without vapor exposure. The air group received the same volume in the chamber with air exposure. Exposure time was about 30 min for 14 days and mice were randomly housed in groups of 2 per cage.
Forelimb grip strength test
A low-force testing system (Model-RX-5, Aikoh Engineering, Nagoya, Japan) was used to measure the forelimb grip strength of treated mice as we previously described [Citation18,Citation19].
Swimming exercise performance test
The swim-to-exhaustion test involved loads corresponding to 5% of the mouse BW (bodyweight) attached to the tail to evaluate endurance time as we previously described [Citation19,Citation20]. The swimming endurance time of each mouse was recorded from beginning to exhaustion, determined by observing the loss of coordinated movements and failure to return to the surface within 7 s.
Tissue glycogen determination and visceral organ weight
The stored form of glucose is glycogen, which exists mostly in liver and muscle tissue. Liver and muscle tissues were excised after the mice were sacrificed and weighed for glycogen content analysis as we described previously [Citation17,Citation18]. The weights of the related visceral organs were recorded.
Clinical biochemical profiles
At the end of the experimental period, mice were sacrificed by 95% CO2 asphyxiation and blood collected immediately. Serum was separated by centrifugation and levels of the clinical biochemical variables, including aspartate aminotransferase (AST), alanine aminotransferase (ALT), albumin, creatinine, blood urea nitrogen (BUN), total protein (TP), uric acid (UA), total cholesterol (TC), triacylglycerols (TG) and lactic dehydrogenase (LDH) were measured by using an autoanalyzer (Hitachi 7060).
Histology of tissues
All tissues were carefully removed and fixed in 10% formalin. Samples were embedded in paraffin and cut into 4-μm thick slices for morphological and histopathological evaluations. Tissues were stained with hematoxylin and eosin (H&E) and examined by a veterinary pathologist using an optical microscope equipped with a CCD camera (BX-51, Olympus, Tokyo).
Statistical analysis
All data are expressed as mean ± SEM, n = 6 mice/group. Statistical differences among groups were analyzed by one-way analysis of variance (ANOVA), followed by Duncan’s post-hoc test for differences between two groups. Dose-effect trend analysis was conducted using the Cochran-Armitage test. All analysis was performed using the SAS 9.0 software (SAS Inst., Cary, NC, USA) with p values < 0.05 considered significant.
Results
Effect of EC-exposure on exercise performance in forelimb grip strength and weight-loaded swimming test
After exposure to ECs for 14 days, we examined the effect of EC on forelimb grip strength in the mice (). The forelimb grip strengths for the control, air, EC-0X, EC-1X, and EC-10X groups were found to be 135 ± 4, 130 ± 9, 134 ± 5, 122 ± 6 and 86 ± 7 g, respectively. Except for the highest dose (EC-10X), all the other EC doses showed statistically insignificant differences in the forelimb grip strength compared with the vehicle or air-exposed controls. We further evaluated the anti-fatigue effects of EC exposure using exercise endurance as an index (). Endurance swimming times were 69 ± 16, 67 ± 17, 62 ± 20, 59 ± 15 and 41 ± 9 min with the control, air, EC-0X, EC-1X, and EC-10X treatment groups, respectively. The forelimb grip strength was lower by 36.21% in the EC-10X group compared to the control group (p < 0.0001). In the trend analysis, absolute exhaustive swimming time showed a slight, though not reaching significance, dose-dependent decrease with EC exposure (p = 0.0873).
Figure 3. Effect of 14 days of EC exposure on forelimb grip strength.
Mice were treated with control, air, EC-0X, EC-1X, and EC-10X for 14 days before forelimb grip strength was tested. Data are expressed as mean ± SEM, 6 mice/group and analyzed by one-way ANOVA. Different letters (a, b) indicating a significant difference at p < 0.05.
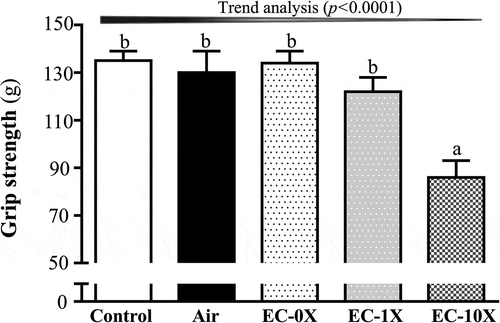
Figure 4. Effect of 14 days of EC exposure on swimming exercise performance.
Mice were treated with control, air, EC-0X, EC-1X, and EC-10X for 14 days. One hour later after the last dose administration, mice were subjected to an exhaustive swimming exercise with loads equivalent to 5% of bodyweight attached to their tails. Data are expressed as mean ± SEM, n = 6 mice/group and analyzed by one-way ANOVA. Different letters (a, b) indicate a significant difference at p< 0.05.
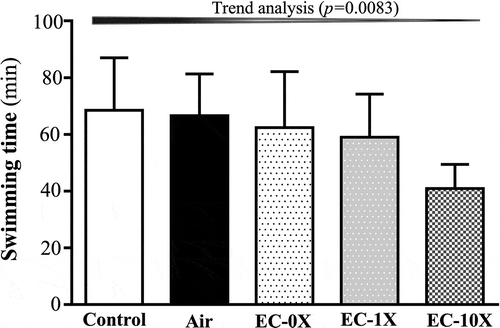
Effect of EC emission for 14 days on hepatic and muscle glycogen level
We measured glycogen content of liver and muscle tissues (). The liver glycogen levels in the control, air, EC-0X, EC-1X, and EC-10X groups were 10.05 ± 0.50, 10.41 ± 1.31, 9.73 ± 1.33, 8.08 ± 3.03 and 5.95 ± 0.65 mg/g, respectively. The EC-10X group showed a decrease of 40.78% (p = 0.0042) of liver glycogen content compared with the control group. The muscle glycogen levels in control, air, EC-0X, EC-1X, and EC-10X groups were 1.69 ± 0.07, 1.74 ± 0.11, 1.71 ± 0.05, 1.62 ± 0.08 and 1.26 ± 0.08 mg/g, respectively. The muscle glycogen content for the EC-10 group was significantly lower by 25.60% (p = 0.0007) than the control group. In the trend analysis, both liver and muscle glycogen content dose-dependently decreased with EC exposure (p = 0.0009; p = 0.0003). Our data suggest that EC emission could decrease both liver and muscle glycogen storage in the mice, leading to a significant effect on exercise performance.
General characteristics of mice with EC emission for 14 days
Initial BW (bodyweight) and final BW of the mice did not differ among the control, air, EC-0X, EC-1X and EC-10X groups (). The BW of the mice in each group steadily increased throughout the 14 days testing period. Similarly, daily intake of diet and water were no different between all the treatment groups. Analysis of the mice body composition after 14 days of EC exposure showed no significant difference in weights of liver, muscle, kidney, lung and ovarian fat pad (OFP). Trend analysis showed dose-dependent heart weight increase with increased EC exposure (p = 0.0448).
Table 1. Characteristic and tissue weights at the end of the experiment.
We also examined the relative tissue weights at the end of 14 days of EC exposure, calculated by % of the different tissue weights adjusted for by individual BW. The relative weights of liver, muscle, kidney, and lung were also not affected by the EC exposure. However, the relative heart weight of the EC-1X and EC-10X groups were 1.12- (p = 0.0207) and 1.13- (p = 0.0153) fold higher than the control group. The relative ovarian fat pad (OFP) weight of EC-10X was significantly decreased compared with vehicle (p = 0.0483), EC-0X (p = 0.0233) and EC-1X (p = 0.0276). In the trend analysis, relative heart weight enhanced in a dose-dependent manner with increased EC dose (p = 0.0032). Taken together, our results suggest that EC exposure may be associated with an increased risk of heart disease.
Effect of 14 days EC exposure on biochemical indices
The biochemical analyses were carried out at the end of the treatment period. Levels of AST, ALT, albumin, creatinine, BUN, TP, TC, TG and LDH were examined and found not to differ significantly among the groups (). Level of UA was significantly higher by 1.63- (p < 0.0001) and 1.65-fold (p < 0.0001) in the EC-1X and EC-10X group, respectively, compared with the control group. Trend analysis also revealed significant (p < 0.0001) dose-dependent increase on serum UA levels. As the risk of obesity, cardiovascular disease, hypertension, chronic kidney disease, and even diabetes are closely related with an increase in UA level, our results suggest that EC exposure may increase this risk.
Table 2. Biochemical analysis of the EC treatment groups at the end of experiment.
Effect of EC exposure on tissue histology
shows the representative histology of the liver, muscle, heart, kidney, lung and ovarian fat tissues taken at the end of treatment period from all the five mice groups (control, air, EC-0X, EC-1X, and EC-10X). Results indicate that there were no histopathological differences among the groups. Additionally, the examination of the tissues showed that EC treatment did not result in any toxic effects on the major organs such as the liver, skeletal muscles, heart, or kidney.
Discussion
In the current study, glycogen content and hepatic protein levels were found to significantly decrease after e-liquid or nicotine exposure [Citation21]. In this study we did not find histopathological abnormalities in the tissues or organs of the mice exposed to 14 days EC with the vanilla-flavored E-liquid, suggesting non-toxicity at the dose used. The previous study suggests that additional regulation may be required to ensure that cytotoxic chemicals such as cinnamaldehyde are not used in EC products, or if used, are at doses that are non-toxic or non-damaging to cells.
We found that EC exposure for 14 days could significantly reduce explosive exercise and endurance exercise performance. EC exposure did not influence BW, food, water intake, organ weights of liver, kidney, lung, muscle, OFP, and BAT. However, heart weight and relative heart weight were significantly increased in the EC-exposed groups. A few studies have reported the cardiovascular effects of e-cigarettes, with the studies suggesting that e-cigarettes can increase heart rate and blood pressure, as should be expected with systemic absorption in nicotine [Citation22]. Although the adverse health effects of e-cigarettes are not known, they are likely to be much less than those of tobacco smoking. Our results show that EC exposure leads to increased uric acid levels, associated with a higher risk of heart disease and obesity. This is in agreement with other studies that suggest that the effect of EC exposure could be significant in individuals with heart disease [Citation22]. Many of these health concerns are related to the ability of nicotine to release catecholamines, increase heart rate, transiently increase blood pressure, coronary vasoconstriction, and other vascular beds, as well as the adverse effects on lipids and induction of insulin resistance [Citation22]. According to our results, nicotine is the main hazardous substances in EC to affect exercise performance. Although average maximum serum nicotine concentration in experienced EC users appears to be generally lower than what has been reported from tobacco cigarette use [Citation23], the recommendation is still that EC-delivery of nicotine could have a negative effect on exercise performance. In previous studies, nicotine intoxication has been shown to be associated with dizziness, nausea, vomiting, pallor, tachycardia, sweating, abdominal pain, salivation, lacrimation, diarrhea and possibly death in cases of severe poisoning, due to hypotension and respiratory muscle weakness [Citation24].
EC exposure also has negative effects on liver and muscle glycogen content. Our data suggest that muscle glycogen levels were dose-dependently decreased with 14 days of EC exposure. A previous study has demonstrated that cigarette smoking leads to an impairment of insulin-dependent muscle glycogen recovery from exercise in a young healthy population [Citation25]. There is much evidence pointing to the deleterious effects of nicotine during exercise [Citation26]. Nicotine could induce the rise in blood pressure that accompanies tachycardia and stimulate the central sympathetic nervous system to locally release catecholamines from the cardiac sympathetic nerve terminals. Higher plasma catecholamine levels are associated with nicotine infusion during exercise and may lead to hemodynamic modifications effects that can compromise myocardial and muscular vascularization. Muscular strength may be impaired and the anaerobic threshold reached earlier [Citation27]. Similarly, we also found that EC exposure decreased liver glycogen storage, in agreement with a previous study demonstrating that diabetic rats exposed to cigarette during pregnancy were impaired in their liver glycogen storage [Citation28]. In addition, Pitzer et al. [Citation29] found that EC exposure could increase basal metabolic activity in mice, a possible reason for the decrease in liver and muscle glycogen storage. This raises the possibility that decreased liver and muscle glycogen storage could be the primary reason for the decrease in explosive and endurance exercise performance. In this study, we have provided conclusive evidence of EC’s effect in decreasing explosive and endurance exercise performance, decreasing muscle glycogen and effecting changes in biochemical indices with 14 days short-term exposure. Even though there were no obvious adverse effects seen on the major organs and tissues, our results do point to short-term nicotine delivery having a negative effect on exercise performance.
Nicotine and nicotine-associated stimuli have been shown to play divergent roles in maintaining smoking behavior in men and women. Females are able to metabolize nicotine faster than males, and therefore, would require more nicotine medication to achieve the same pharmacologic benefit. Additionally, it is possible that the presence of estrogen may block sensitization to nicotine [Citation30–Citation32].
The safety and health effects of e-cigarettes have been reviewed previously elsewhere [Citation33]. Past studies have concluded that tobacco smoking could have relatively little impact on the development of sarcopenia [Citation34] and loss of exercise capacity. Although it has been reported that tobacco smoke could induce muscular atrophy and loss of oxidative muscle capacity through dysregulation of several metabolic pathways [Citation35], to our knowledge, there has yet not been a study discussing the impact of EC on muscle strength. Our current study provides preliminary data showing a significant effect on exercise performance, accompanied with certain biochemical changes, in mice exposed to EC. Future research is still needed to evaluate the effect of EC on exercise performance in athletics and assess the health impact of long-term exposure to EC. This study and others would contribute towards determining the overall impact and hazards of e-cigarettes on the human population.
Author Contributions
Yi-Ming Chen, Chi-Chang Huang designed the experiments. Yi-Ming Chen, Mon-Chien Lee, Hsin-Ching Sung, and Chi-Chang Huang carried out the laboratory experiments. Yi-Ming Chen and Chi-Chang Huang analyzed the data, interpreted the results, prepared figures, and wrote the manuscript. Yi-Ming Chen and Chi-Chang Huang revised the manuscript. Yi-Ming Chen and Chien-Yu Hsiao contributed the reagents, materials and analysis platforms.
Acknowledgments
The authors thank Chien-Chao Chiu or conducting the histological and pathological examination.
Disclosure statement
No potential conflict of interest was reported by the authors.
Additional information
Funding
References
- Etter JF. Electronic cigarettes: a survey of users. BMC Public Health. 2010 May 4;10:231. PubMed PMID: 20441579; PubMed Central PMCID: PMCPMC2877672. eng.
- Etter JF, Bullen C. Electronic cigarette: users profile, utilization, satisfaction and perceived efficacy. Addiction. 2011 Nov;106(11):2017–2028. PubMed PMID: 21592253; eng.
- Dawkins L, Turner J, Roberts A, et al. ‘Vaping’ profiles and preferences: an online survey of electronic cigarette users. Addiction. 2013 Jun;108(6):1115–1125. PubMed PMID: 23551515; eng.
- Dockrell M, Morrison R, Bauld L, et al. E-cigarettes: prevalence and attitudes in Great Britain. Nicotine Tob Res. 2013 Oct;15(10):1737–1744. PubMed PMID: 23703732; PubMed Central PMCID: PMCPMC3768337. eng.
- Farsalinos KE, Romagna G, Tsiapras D, et al. Evaluating nicotine levels selection and patterns of electronic cigarette use in a group of “vapers” who had achieved complete substitution of smoking. Subst Abuse. 2013;7:139–146. PubMed PMID: 24049448; PubMed Central PMCID: PMCPMC3772898. eng.
- Ayers JW, Ribisl KM, Brownstein JS. Tracking the rise in popularity of electronic nicotine delivery systems (electronic cigarettes) using search query surveillance. Am J Prev Med. 2011 Apr;40(4):448–453. PubMed PMID: 21406279; eng.
- Noel JK, Rees VW, Connolly GN. Electronic cigarettes: a new ‘tobacco’industry? Tob Control. 2011;20(1):81.
- Riker CA, Lee K, Darville A, et al. E-cigarettes: promise or peril? Nurs Clin. 2012;47(1):159–171.
- Goniewicz ML, Lingas EO, Hajek P. Patterns of electronic cigarette use and user beliefs about their safety and benefits: an internet survey. Drug Alcohol Rev. 2013 Mar;32(2):133–140. PubMed PMID: 22994631; PubMed Central PMCID: PMCPMC3530631. eng.
- Narkiewicz K, van de Borne PJ, Hausberg M, et al. Cigarette smoking increases sympathetic outflow in humans. Circulation. 1998 Aug 11;98(6):528–534. PubMed PMID: 9714109; eng.
- Weber F, Anlauf M, Muller RD. Changes in muscle blood flow after smoking a cigarette determined by a new noninvasive method. Eur J Clin Pharmacol. 1989;37(5):517–520. PubMed PMID: 2598992; eng.
- Mundel T, Jones DA. Effect of transdermal nicotine administration on exercise endurance in men. Exp Physiol. 2006 Jul;91(4):705–713. PubMed PMID: 16627574; eng.
- Tucha O, Lange KW. Effects of nicotine chewing gum on a real-life motor task: a kinematic analysis of handwriting movements in smokers and non-smokers. Psychopharmacology (Berl). 2004 Apr;173(1–2):49–56. PubMed PMID: 14668975; eng.
- Lee CL, Chang WD. The effects of cigarette smoking on aerobic and anaerobic capacity and heart rate variability among female university students. Int J Womens Health. 2013;5:667–679. PubMed PMID: 24204174; PubMed Central PMCID: PMCPMC3804543. eng.
- Hoyt GL. Cigarette smoking: nicotine, carbon monoxide, and the physiological effects on exercise responses. Sport Sci Rev. 2013;22(1–2):5–24.
- Chen YM, Wei L, Chiu YS, et al. Lactobacillus plantarum TWK10 supplementation improves exercise performance and increases muscle mass in mice. Nutrients. 2016 Apr 7;8(4):205. PubMed PMID: 27070637; PubMed Central PMCID: PMCPMC4848674. eng.
- Chen YM, Lee HC, Chen MT, et al. Dehydroepiandrosterone supplementation combined with Weight-Loading Whole-Body Vibration Training (WWBV) affects exercise performance and muscle glycogen storage in middle-aged C57BL/6 mice. Int J Med Sci. 2018;15(6):564–573.
- Ma GD, Chiu CH, Hsu YJ, et al. Changbai Mountain Ginseng (Panax ginseng C.A. Mey) extract supplementation improves exercise performance and energy utilization and decreases fatigue-associated parameters in mice. Molecules. 2017 Feb 5;22(2):237. PubMed PMID: 28165424; eng.
- Chen YM, Tsai YH, Tsai TY, et al. Fucoidan supplementation improves exercise performance and exhibits anti-fatigue action in mice. Nutrients. 2014 Dec 31;7(1):239–252. PubMed PMID: 25558908; PubMed Central PMCID: PMCPMC4303836. eng.
- Chen YM, Lin CL, Wei L, et al. Sake protein supplementation affects exercise performance and biochemical profiles in power-exercise-trained mice. Nutrients. 2016;8(2):106.
- El Golli N, Jrad-Lamine A, Neffati H, et al. Impact of e-cigarette refill liquid with or without nicotine on liver function in adult rats. Toxicol Mech Methods. 2016 Jul;26(6):419–426. PubMed PMID: 27484987; eng.
- Farsalinos KE, Polosa R. Safety evaluation and risk assessment of electronic cigarettes as tobacco cigarette substitutes: a systematic review. Ther Adv Drug Saf. 2014 Apr;5(2):67–86. PubMed PMID: 25083263; PubMed Central PMCID: PMCPMC4110871. eng.
- St Helen G, Havel C, Dempsey DA, et al. Nicotine delivery, retention and pharmacokinetics from various electronic cigarettes. Addiction. 2016 Mar;111(3):535–544. PubMed PMID: 26430813; PubMed Central PMCID: PMCPMC4749433. eng.
- Solarino B, Rosenbaum F, Riesselmann B, et al. Death due to ingestion of nicotine-containing solution: case report and review of the literature. Forensic Sci Int. 2010 Feb 25;195(1–3):e19–e22. PubMed PMID: 19954906; eng.
- Price TB, Krishnan-Sarin S, Rothman DL. Smoking impairs muscle recovery from exercise. Am J Physiol Endocrinol Metab. 2003 Jul;285(1):E116–E122. PubMed PMID: 12637259; eng.
- Symons JD, Stebbins CL. Hemodynamic and regional blood flow responses to nicotine at rest and during exercise. Med Sci Sports Exerc. 1996 Apr;28(4):457–467. PubMed PMID: 8778551; eng.
- Chague F, Guenancia C, Gudjoncik A, et al. Smokeless tobacco, sport and the heart. Arch Cardiovasc Dis. 2015 Jan;108(1):75–83. PubMed PMID: 25497687; eng.
- Sinzato YK, Lima PH, Santos CE, et al. Association of diabetes and cigarette smoke exposure on the glycemia and liver glycogen of pregnant Wistar rats. Acta Cir Bras. 2008 Nov–Dec;23(6):481–485. PubMed PMID: 19030744; eng.
- Pitzer C, Breit M, Hoskinson H, et al. Metabolic monitoring and cage activity in mice exposed to 7-months of electronic cigarette vapor. Faseb J. 2017;31(1 Supplement):1089.12.
- Bjornson W, Rand C, Connett JE, et al. Gender differences in smoking cessation after 3 years in the lung health study. Am J Public Health. 1995 Feb;85(2):223–230. PubMed PMID: 7856782; PubMed Central PMCID: PMCPMC1615316. eng.
- Benowitz NL, Lessov-Schlaggar CN, Swan GE, et al. Female sex and oral contraceptive use accelerate nicotine metabolism. Clin Pharmacol Ther. 2006 May;79(5):480–488. PubMed PMID: 16678549; eng.
- Damaj MI. Influence of gender and sex hormones on nicotine acute pharmacological effects in mice. J Pharmacol Exp Ther. 2001 Jan;296(1):132–140. PubMed PMID: 11123373; eng.
- Bhatnagar A, Whitsel LP, Ribisl KM, et al. Electronic cigarettes: a policy statement from the American Heart Association. Circulation. 2014 Oct 14;130(16):1418–1436. PubMed PMID: 25156991; eng.
- Steffl M, Bohannon RW, Petr M, et al. Relation between cigarette smoking and sarcopenia: meta-analysis. Physiol Res. 2015;64(3):419–426. PubMed PMID: 25536323; eng.
- Kruger K, Dischereit G, Seimetz M, et al. Time course of cigarette smoke-induced changes of systemic inflammation and muscle structure. Am J Physiol Lung Cell Mol Physiol. 2015 Jul 15;309(2):L119–L128. PubMed PMID: 26001775; eng.