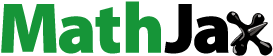
ABSTRACT
In experiments 1 and 2, effect of ingestion of maltobionic acid calcium salt (MBCa) on recovery of rats from a latent iron deficiency and from iron deficiency anemia was examined, respectively. After grouping rats into control and iron-deficiency groups, a latent iron deficiency or iron-deficiency anemia was induced in the latter group. And recovery from these states by MBCa containing diets (0%, 3%, and 6% MBCa in diet, classified into MBCa-0, MBCa-3, and MBCa-6 groups) was compared for convalescence period in light of iron sufficient control group. In experiment 1, MBCa ingestion significantly increased the iron concentration in the serum and liver, and promoted recovery from a latent iron deficiency. In experiment 2, hemoglobin and hematocrit levels increased significantly with MBCa intake, and recovery from iron-deficiency anemia was promoted. MBCa effectively promoted the recovery of rats from a subclinical iron deficiency and iron-deficiency anemia.
Abbreviations: ANOVA: analysis of variance; DMT1: divalent metal transporter 1; EDTA-2Na: disodium salt of ethylenediaminetetraacetic acid; Fpn: feroportin; Hb: hemoglobin; Ht: hematocrit; ICP-OES: inductivity coupled plasma optical emission spectrometer; MBCa: maltobionic acid calcium salt; nitroso-PSAP: 2-nitroso-5-[N-n-propyl-N-(3-sulfopropyl)amino]phenol; SE: standard error; SI: serum-iron concentration; TSAT: transferrin saturation; TIBC: total iron-binding capacity; UIBC: unsaturated iron-binding capacity.
Graphical abstract

Maltobionic acid accelerates recovery from anemia.
Iron, a trace metal, is essential for the maintenance of normal vital phenomena and is utilized during many life activities, including oxygen delivery, redox reactions, and nucleic acid synthesis [Citation1]. Iron-deficiency anemia is a frequently occurring nutrient deficiency disease worldwide; several countries have taken various countermeasures against it [Citation2]. In Japan, more than 10 million Japanese people have iron-deficiency anemia [Citation3]. The prevalence of iron-deficiency anemia and latent iron deficiency especially continues to increase among younger individuals and has become a problem [Citation4]. Iron-deficiency anemia causes tissue hypoxia, fatigue, headache, and palpitations. Iron-deficiency symptoms are not limited to anemia alone. Prolonged iron deficiency is known to cause inferior papillary atrophy, angular cheilitis, etc [Citation5]. Its association with neurological and psychiatric symptoms has been reported; for example, epidemiological evidence has shown that the restless leg syndrome is associated with iron-deficiency anemia [Citation6].
Iron is found in two forms, i.e. heme and non-heme iron, in animal and plant foods, respectively; however, non-heme iron constitutes approximately 90% of the iron absorbed from food [Citation7]. Though iron in foods is absorbed in the intestinal tract [Citation8], non-heme iron is very poorly absorbed, as compared with heme iron [Citation9]. Therefore, it is necessary to make iron absorption more efficient, to supplement the daily diet with iron.
Maltobionic acid (4-O-α-D-Glucopyranosyl-D-gluconic acid: CAS No. 534-42-9), which contain α-1,4-chain linked to glucose and gluconate, is an indigestible disaccharide found in honey. It can characteristically form stable salts with inorganic cations, and maintain high water solubility, upon binding to calcium and iron [Citation10]. We previously reported that maltobionic acid enhanced calcium and magnesium absorption, and increased calcium levels in the femur of rats, by maintaining the solubilized state of minerals throughout the gut [Citation11,Citation12]. In addition, a 24-week interventional study in postmenopausal women confirmed that maltobionic acid calcium salt (MBCa) ingestion maintains and increases bone mineral density; we also confirmed the safety of MBCa ingestion in a long-term ingestion study [Citation13]. There were no other previous reports about how maltobionic acid affected iron absorption and recovery from iron deficiency. Therefore, this study aimed to investigate how a maltobionic acid-containing diet enhanced iron-deficiency anemia in rats.
Materials and methods
Experimental animals
The study protocol was approved by the Gifu University Committee for Animal Research and Welfare and the Proper Use of Laboratory Animals (Approval number: 16074 for Exp. 1 and 17133 for Exp. 2). Male Wistar rats were obtained from SLC (Shizuoka, Japan). The rats were housed individually in stainless-steel wire-bottomed cages, in a room at 23 ± 1°C, under inverted 12-h light-dark cycle conditions (lights on at 06:00 AM). Prior to each feeding experiment, the rats were allowed to adapt to the AIN-93G diet for 7 d.
Experimental diets
The compositions of the diets used in experiments 1 and 2 are described in and . Two types of anemia-inducing diets were derived by partially modifying the AIN-93G diet provided to control group rats, i.e. the AIN-93G standard (Control diet; Control group) and iron-free (iron-deficient) diets. Three experimental diets were used during the recovery from iron deficiency, including the control diet (MBCa-0 group), 3% MBCa-supplemented diet (MBCa-3 group), and 6% MBCa-supplemented diet (MBCa-6 group). The iron content of the three recovery diets was adjusted to 100% of the iron content in the AIN-93G standard diet provided during experiment 1 () and to 50% of the iron content in the AIN-93G standard diet provided during experiment 2 (). MBCa was prepared by the method [Citation14] described by Fukami et al., using ferric citrate, calcium carbonate, sucrose, and soybean oil purchased from Nacalai Tesque Co., Ltd. (Kyoto, Japan), choline bitartrate purchased from FUJIFILM Wako Pure Chemicals, Co., Ltd. (Osaka, Japan), and other feed materials purchased from the Oriental Yeast Co., Ltd. (Tokyo, Japan).
Table 1. Composition of the experimental diets for Exp. 1 (gram per 100 g of diet).
Table 2. Composition of the experimental diets for Exp. 2 (gram per 100 g of diet).
Methodology for animal experiments
Recovery from latent iron deficiency (Exp. 1)
Eight-week-old rats were divided into the control (n = 7) and iron-deficient (n = 21) groups in such a manner that the mean body weights were equal, and then kept for 11 d; a latent iron-deficiency was induced in the iron-deficient group. The iron-deficient group was further divided into the MBCa-0, MBCa-3, and MBCa-6 (n = 7) groups in such a manner that rats from all the iron-deficient groups had equal serum-iron concentration (SI); they were then reared for 14 days (recovery period). During the feeding period, the animals were fed ad libitum and ion-exchanged water was provided for drinking. Five hundred microliters of blood were collected from the tail vein on day 0, 4, 11, and 15 of the recovery period. Rats were subjected to anesthesia with pentobarbital sodium (Somnopentyl®; Kyoritsu Pharmaceutical Co., Ltd, Tokyo, Japan) at 8:00 AM, 15 d after the start of the experiment. Blood samples were collected from the abdominal aorta using a syringe treated with heparin; rats were euthanized via cervical dislocation, and their spleen and liver were collected and weighed. The liver was perfused with physiological saline to remove blood from the liver.
Recovery from iron-deficiency anemia (Exp.2)
Four-week-old rats were divided into the control (n = 8) and iron-deficient (n = 24) groups in such a manner that their mean body weights were equal and then kept for 28 d, and an iron-deficiency was induced in the iron-deficient group. The iron‐deficient group was further divided into the MBCa-0, MBCa-3, and MBCa-6 (n = 8) groups in such a manner that the blood hemoglobin (Hb) and hematocrit (Ht) levels became equal, and the iron‐deficient group was bred for 28 d (recovery period). The animals were fed ad libitum and ion-exchanged water was provided for drinking. Three hundred and fifty microliters of blood were collected from the tail vein of rats on day 0 (week 0), 7 (week 1), 14 (week 2), 21 (week 3), and 28 (week 4) of the recovery period. Feces were collected from days 3–5 (week 1), 10–12 (week 2), 17–19 (week 3), and 24–26 (week 4) after initiating the study. Collected fecal samples were lyophilized and powdered using a Milser device (IFM-600 DG, Iwatani Sangyo Co., Ltd., Osaka, Japan). Rats were anesthetized with pentobarbital sodium at 9:30 AM on day 29 after initiating the experiment. After laparotomy, the animals were bled from the abdominal aorta using a heparinized syringe and euthanized via cervical dislocation. The spleen and liver were collected and weighed. The liver was perfused with physiological saline to remove blood from the liver.
Measurement of hematological parameters
A certain amount of the tail vein blood was supplemented with 1.5 mg/mL of disodium salt of ethylenediaminetetraacetic acid (EDTA-2Na), and Hb and Ht levels were analyzed on the day of blood collection. Hb levels were measured using the SLS hemoglobin method [Citation15]. Ht values showed the percentage of red blood cell volume to total blood volume after the blood was centrifuged in a Hematocrit centrifuge (Model-3220, KUBOTA Corporation, Tokyo, Japan) at 12,000 rpm for 5 min. The SI value and unsaturated iron-binding capacity (UIBC) were determined using the nitroso-PSAP (2-nitroso-5-[N-n-propyl-N-(3-sulfopropyl)amino]phenol) [Citation16] and bathophenanthroline methods, respectively [Citation17]. Total iron-binding capacity (TIBC) and serum-transferrin iron saturation (TSAT) values were calculated using the following equations:
Determination of hepatic iron and apparent iron absorption levels
We weighed 1 g of powdered feces or 0.5 g of liver tissue were weighed out into a Teflon beaker, added to 10 mL of concentrated nitric acid (heating for 90 min), then 10 mL of 70% perchloric acid (heating for 90 min), and finally 2 mL of 6 M hydrochloric acid (heating for 30 min), consecutively. Heating was done on a hot plate at 120°C to achieve wet ashing. Liver or fecal iron concentrations were determined using an ICP-OES device (Optima 7300 DV, Perkin Elmer, MA, USA). Apparent iron absorption rates were calculated from the quantities of iron present in excreta, using the following formula:
Apparent iron absorption rate (%) = [(iron intake-iron in feces)/(iron intake)] × 100
Statistical analysis
Data are expressed as mean ± standard error (SE) values. Statistical analysis of the control and MBCa-0 groups was initially performed using the Student’s t-test. If significant difference was confirmed to be present with regard to the control group, asterisks (*) were added to the value for the MBCa-0 group. One-way analysis of variance (ANOVA) and the Bartlett’s test were performed for all the three groups (MBCa-0, MBCa-3, and MBCa-6 groups), followed by Tukey’s multiple-comparison test. In cases involving unequal variance, a nonparametric (Kruskal–Wallis) test was performed, followed by the Steel–Dwass multiple-comparison test. Alphanumeric characters were used to indicate values for which differences were statistically significant, and groups not marked with the same symbol were assumed to be significantly different. The level of statistical significance in all the above tests was defined as a probability of less than 5% (p < 0.05). Microsoft Excel 2010 and BellCurve for Excel (Social Survey Research Information Co., Ltd., Tokyo, Japan) were used to conduct these analyses.
Results
Recovery from latent iron deficiency (Exp. 1)
Final body weight, weight gain, food intake, and food efficiency ratio
The final body weight, weight gain, food intake, and food efficiency ratio of the rats during the recovery period are described in . No differences were observed among the groups, with regard to any of the measured parameters.
Table 3. Growth parameters,food intake and feed efficiency of rats fed the experimental diets during recovery period. (Exp. 1).
Serum iron concentration and plasma transferrin saturation
At the beginning of the recovery process, the SI values of the control and iron-deficient diet groups were 231 μg/L and approximately 84 μg/L, respectively (). SI value in the MBCa-0 group was significantly lower as compared to the other three groups up to day 11. Significant increases were observed in MBCa-3 and MBCa-6 groups on day 4 and day 11 as compared to the values for the MBCa-0 group, and significantly higher values were observed for the MBCa-3 group on day 15 of the study (). The TSAT levels of the control and iron-deficient diet groups were 57% and 18%, respectively, at the beginning of the recovery process (). TSAT of the MBCa-0 group A was significantly low at all time periods as compared to value observed for the control group. In comparison to the values in the iron-deficient groups, the values in the MBCa-6 group were significantly higher than that in the MBCa-0 group on days 4 and 11, and values in the MBCa-3 group were significantly higher than that in the iron-deficient group after day 4 ().
Figure 1. Serum iron concentration (a) and transferrin saturation (b) of rats fed the experimental diets. (Exp. 1).
Rats were fed the experimental diets shown in for 15 d.○ Control, ▲MBCa-0,■ MBCa-3, ●MBCa-6Transferrin saturation (%) = [Serum iron (µg/dL)/(Unsaturated iron-binding capacity (µg/dL) + serum iron (µg/dL))] ×100Values are expressed as mean±SE (n = 7). For the experimental groups, see .* Significantly different from the control group at p < 0.05 as determined by Student’s t-test. Means in the same day, not sharing the same superscript letter, are significantly different at p < 0.05 as determined by one-way ANOVA, followed by Tukey’s multiple comparison test.
![Figure 1. Serum iron concentration (a) and transferrin saturation (b) of rats fed the experimental diets. (Exp. 1).Rats were fed the experimental diets shown in Table 1 for 15 d.○ Control, ▲MBCa-0,■ MBCa-3, ●MBCa-6Transferrin saturation (%) = [Serum iron (µg/dL)/(Unsaturated iron-binding capacity (µg/dL) + serum iron (µg/dL))] ×100Values are expressed as mean±SE (n = 7). For the experimental groups, see Table 1.* Significantly different from the control group at p < 0.05 as determined by Student’s t-test. Means in the same day, not sharing the same superscript letter, are significantly different at p < 0.05 as determined by one-way ANOVA, followed by Tukey’s multiple comparison test.](/cms/asset/49d5f407-8302-409e-b29c-90722f32cbc6/tbbb_a_1676694_f0001_b.gif)
Liver weight, spleen weight, and liver iron concentration
Liver weight, splenic weight, and liver iron content are shown in . Liver and spleen weights did not differ among all groups. The iron concentration in liver was significantly lower in the MBCa-0 group than in the control group. Among iron-deficient groups, the values observed in the MBCa-6 group were significantly higher than values in the MBCa-0 group.
Table 4. Weight of spleen and liver, and iron concentration in liver of rats fed the experimental diets. (Exp. 1).
Recovery from iron-deficiency anemia (Exp.2)
Final body weight, weight gain, food intake, and food efficiency ratio
The final body weight, weight gain, food intake, and food efficiency ratio of rats during the recovery period are described in . The final body weight and feed efficiency ratio were significantly lower in the MBCa-0 group than in the control group. The body weight gain and food intake did not differ across all groups.
Table 5. Growth parameters,food intake and feed efficiency of rats fed the experimental diets during recovery period. (Exp. 2).
Hemoglobin, hematocrit, plasma iron concentration, and plasma transferrin saturation
Time courses of Hb, Ht, SI, and TSAT during the recovery period are shown in , respectively. A significant decrease in Hb () and Ht () levels were observed in the MBCa-0 group, as compared with the control group, on all days when blood was collected. In the iron-deficient groups, both Hb and Ht levels were significantly higher in the MBCa-6 group than in the MBCa-0 group, after week 3. The values for the MBCa-3 group were also significantly higher on week 4. The SI values for control and iron-deficient diet groups were 317 μg/L and approximately 20 μg/L, respectively, at the beginning of the recovery period. SI value in the MBCa-0 group was significantly lower as compared to the control group (). The values were significantly higher in the MBCa-6 group than in the MBCa-0 group after week 3. The values for the MBCa-3 group were also significantly higher on week 4. At the beginning of the recovery period, the TSAT levels in the control and iron-deficient diet groups were 63% and approximately 3%, respectively. In comparison with the control group, the MBCa-0 group showed a significant reduction at all time periods (). Among the iron-deficient groups, the MBCa-6 group showed significantly higher values on week 4.
Figure 2. Hemoglobin (a), hematocrit (b), serum iron concentration (c) and transferrin saturation (d) of rats fed the experimental diets. (Exp. 2).
Rats were fed the experimental diets shown in for 28 d.○ Control, ▲MBCa-0,■ MBCa-3, ●MBCa-6Transferrin saturation (%) = [Serum iron (µg/dL)/(Unsaturated iron-binding capacity (µg/dL) + serum iron (µg/dL))] ×100Values are expressed as mean±SE (n = 8).For the experimental groups, see . *Significantly different from the control group at p < 0.05 as determined by Student’s t-test. Means in the same week, not sharing the same superscript letter, are significantly different at p < 0.05 as determined by one-way ANOVA, followed by Tukey’s multiple-comparison test except Week 4 in ) and Week 3 in ), which are analyzed by the Steel-Dwass multiple-comparison test.
![Figure 2. Hemoglobin (a), hematocrit (b), serum iron concentration (c) and transferrin saturation (d) of rats fed the experimental diets. (Exp. 2).Rats were fed the experimental diets shown in Table 2 for 28 d.○ Control, ▲MBCa-0,■ MBCa-3, ●MBCa-6Transferrin saturation (%) = [Serum iron (µg/dL)/(Unsaturated iron-binding capacity (µg/dL) + serum iron (µg/dL))] ×100Values are expressed as mean±SE (n = 8).For the experimental groups, see Table 2. *Significantly different from the control group at p < 0.05 as determined by Student’s t-test. Means in the same week, not sharing the same superscript letter, are significantly different at p < 0.05 as determined by one-way ANOVA, followed by Tukey’s multiple-comparison test except Week 4 in Figure 2(a) and Week 3 in Figure 2(b), which are analyzed by the Steel-Dwass multiple-comparison test.](/cms/asset/374b1c16-8603-49a7-be4d-94c77cb00800/tbbb_a_1676694_f0002_b.gif)
Liver weight, spleen weight, and liver iron concentration
The liver weight, spleen weight, and liver iron concentration are shown in . The liver weight and iron concentration in the liver were significantly lower in the MBCa-0 group than in the control group. There was no difference in spleen weight.
Table 6. Weight of spleen and liver, and iron concentration in liver of rats fed the experimental diets. (Exp. 2).
Apparent rate of iron absorption
shows the change in apparent iron absorption during the recovery period. The apparent iron absorption in the MBCa-0 group was significantly higher than that in the control group at all time periods. Furthermore, the values of the MBCa-6 group were significantly higher than values of the MBCa-0 and MBCa-3 groups during week 1 and week 2 of rearing, respectively.
Figure 3. Apparent iron absorption rate of rats fed on the experimental diets (Exp. 2).
Rats were fed the experimental diets shown in for 28 d. Feces were collected and the apparent iron absorption rate was calculated on days 3–5 (Week 1), 10–12 (Week 2), 17–19 (Week 3), and 24–26 (Week 4).○ Control, ▲MBCa-0,■ MBCa-3, ●MBCa-6Apparent iron absorption rate (%): [(total iron intake – iron excretion in feces)/total iron intake] × 100Values are expressed as mean±SE (n = 8). For the experimental groups, see . *Significantly different from the control group at p < 0.05 as determined by Student’s t-test. Means in the same period, not sharing the same superscript letter, are significantly different at p < 0.05 as determined by one-way ANOVA, followed by Tukey’s multiple-comparison test.
![Figure 3. Apparent iron absorption rate of rats fed on the experimental diets (Exp. 2).Rats were fed the experimental diets shown in Table 2 for 28 d. Feces were collected and the apparent iron absorption rate was calculated on days 3–5 (Week 1), 10–12 (Week 2), 17–19 (Week 3), and 24–26 (Week 4).○ Control, ▲MBCa-0,■ MBCa-3, ●MBCa-6Apparent iron absorption rate (%): [(total iron intake – iron excretion in feces)/total iron intake] × 100Values are expressed as mean±SE (n = 8). For the experimental groups, see Table 2. *Significantly different from the control group at p < 0.05 as determined by Student’s t-test. Means in the same period, not sharing the same superscript letter, are significantly different at p < 0.05 as determined by one-way ANOVA, followed by Tukey’s multiple-comparison test.](/cms/asset/47e63b48-c1db-414b-aa88-5b26afb740a4/tbbb_a_1676694_f0003_b.gif)
Discussion
Iron-deficiency anemia results from an iron deficiency, caused by a decreased iron supply. When the iron balance is negatively skewed, the stored iron content begins to decrease. Iron, which is not biologically available, is stored in the liver and kidneys as ferritin and hemosiderin. The amount stored in the liver is especially large [Citation18]. Further, the reduction in iron concentration in the liver is followed by increase in the serum ferritin level. If the intake of iron decreases, the TSAT level decreases and the TIBC level increases, resulting in “latent iron deficiency”. In addition, as the iron-deficiency state progresses, subjective symptoms of anemia appear, and Hb and Ht levels decrease, resulting in “iron deficiency anemia” [Citation19]. At the end of the iron-deficiency-inducing process in Exp.1, the group provided with the iron-deficient diet had significantly lower SI and TSAT levels, as compared to those of the control group (). However, there were no differences in Hb and Ht (data not shown) levels. Therefore, it was confirmed that the latent iron-deficiency condition had been induced in the iron-deficient diet group. SI and TSAT levels were significantly higher in both the MBCa-3 and MBCa-6 groups after 4 days of recovery, indicating that dietary iron was more efficiently absorbed in the MBCa diet groups compared to the MBCa-0 group.
In addition, the iron concentration in the liver was measured as an index, to determine the potential iron-deficiency level in the body. The iron concentration was significantly higher in the liver of rats in the MBCa-6 group than in that of the MBCa-0 group, and the iron content was almost recovered to the level identified in the control group (). From the above results, it was clarified that the ingestion of MBCa promoted the recovery of rats from latent iron deficiencies.
After iron-deficiency induction in Exp. 2, the iron-deficient diet group showed a significant decrease in Hb, Ht, SI, and TSAT levels, which confirmed the induction of iron-deficiency anemia (). On week 4 after the start of recovery, the SI, TSAT, Hb and Ht levels in the MBCa-6 group, and the Hb and Ht levels in the MBCa-3 group, respectively, were observed to be significantly recovered. First, the levels of blood parameters such as Hb and Ht were increased, indicating recovery from iron-deficiency anemia. Finally, the SI and TSAT levels were increased, and the rats recovered from the state of latent iron deficiency [Citation20]. From the above results, it was clarified that the intake of MBCa promoted recovery from iron-deficiency anemia.
Iron is absorbed mainly in the duodenum; there are two types of iron in the diet: non-heme and heme iron, each with a different absorption mechanism. Non-heme iron is taken up into intestinal epithelial cells by the divalent metal transporter 1 (DMT1), though the iron in the intestinal tract would then need to be solubilized [Citation21]. However, acidified digesta by gastric acid is gradually neutralized by pancreatic and intestinal juice in the duodenum, and poorly soluble salts are formed with the coexisting phosphoric acid as the pH increases, which results in poor iron absorption [Citation22]. The solubility of iron citrate used in the test diet in this study was low, and insoluble salts were formed with an increase in pH. However, it was confirmed that the presence of maltobionic acid in the intestinal tract solubilizes calcium and magnesium and contributes to enhanced absorption [Citation12]. Similar effects were also expected to be observed with iron. Notably, the apparent iron absorption rate in Exp. 2 was significantly higher in the MBCa-6 and MBCa-3 groups than in the MBCa-0 group during weeks 1 and 2, respectively (). The iron absorption rate is dependent on the iron-deficiency level and is inversely proportional to the state of anemia [Citation23]. Hence, the iron requirement of the organism was thought to become lowered because of its recovery from serious anemic conditions during week 1 and 2 owing to the intake of MBCa, the increase in iron absorption rate by MBCa diminished after week 3. However, the iron absorption rate was significantly higher in the MBCa-0 group at this period, because the hepatic iron content was not fully recovered.
Indigestible saccharides, such as water-soluble dietary fibers and oligosaccharides, are reportedly fermented by intestinal bacteria in the large intestine to produce short-chain fatty acids, including acetic acid, propionic acid, n-butyric acid, or organic acids, such as lactic acid and succinic acid. These acids acidify the lumen and increase the amount of minerals solubilized in the intestine, and promoting the intestinal absorption of iron [Citation24–Citation26]. In gastrectomized rat models, wherein the process of solubilization by gastric acid was inhibited, the decrease in iron uptake was ameliorated by ingestion of fermentable indigestible oligosaccharides, such as fructooligosaccharide [Citation27] and difructose anhydride III [Citation28], because colonic fermentation and solubilization of iron was promoted in the large intestine. In our previous study, maltobionic acid ingestion was associated with an increase in absorption of calcium, though the cecal pH was not significantly decreased [Citation11,Citation12]. Therefore, instead of enhancing the mineral absorption by decreasing the pH, as caused by indigestible oligosaccharides, maltobionic acid maintains the solubilization state of iron without lowering the pH in the intestine. Moreover, iron absorption is considered to occur efficiently throughout the intestine, because maltobionic acid was liberated from MBCa after absorption of calcium in the upper part of the small intestine [Citation11]. Taken together, these results indicate that early recovery would be observed with the intake of negatively charged maltobionic acid by rats in which subclinical iron deficiency and iron-deficiency anemia had been induced. This suggests that maltobionic acid can promote recovery in rats with both latent iron deficiency and iron-deficiency anemia, and enables iron to be solubilized throughout the intestine, thus resulting in the efficient intestinal absorption of iron.
Iron is an indispensable nutrient; however, excess ironworks as pro-oxidant to produce hydroxyl radicals. Therefore, iron absorption has a regulatory system [Citation29,Citation30]. There are two iron transporters in epithelial cells, namely DMT1, an importer of iron, and feroportin (Fpn), an exporter of iron. Expression of hepcidine, a peptide hormone produced in the liver is promoted during iron deficiency and blood hepsidine binds to Fpn, which accelerates the internalization of the complex and the degradation of Fpn. Thus, the export of iron from enterocytes to ferritin is reduced. An inverse relationship between hepcidine expression and iron absorption, as well as the expression of duodenal iron transporters was reported in rats [Citation31]. In Exp. 1, SI and TSAT of the MBCa-6 group were lower than or equal to those of the MBCa-3 group on day 15, unlike on day 11. These changes may occur in response to changes in iron homeostasis, wherein the iron content in the liver of MBCa-6 was recovered as compared to MBCa-3. At present, we did not delineate the detailed mechanism of these changes. Further experiments are needed to elucidate the exact underlying mechanisms. As compared to Exp. 1, in Exp. 2 recovery from iron deficiency was slow because rats were under severe iron deficiency and the iron content in the recovery diet was half of that of Exp. 1. This may be partly due to the preferential utilization of absorbed iron for hepatogenesis.
Thus, our results suggest that the mechanism of action of maltobionic acid is to aid the solubilization of iron in the entire intestine, as maltobionic acid is retained after absorption of calcium. To further confirm this hypothesis, we need to analyze the dynamic changes in iron and maltobionic acid using an unabsorbable marker. In addition, the possibility of excess absorption of iron needs to be examined using an iron repleted diet and prolonged feeding conditions.
Author contributions
Daiki Suehiro, Haruka Kawase, Sumire Uehara, and Takashi Hayakawa formulated the study design. Daiki Suehiro drafted the manuscript, and Takashi Hayakawa helped to complete the manuscript preparation process. Ken Fukami, Tomoyuki Nakagawa, and Masaya Shimada reviewed the manuscript. Rio Kawase participated in conducting the analyses. All the authors have reviewed and approved of the final manuscript.
Acknowledgments
We would like to thank Editage (www.editage.com) for English language editing. At the same time, we would like to thank others who contributed to the experiment but have not been listed as authors.
Disclosure statement
Daiki Suehiro and Ken Fukami are employees of San-ei Sucrochemical Co., Ltd.
References
- Hentze MW, Muckenthaler MU, Galy B, et al. Two to tango: regulation of mammalian iron metabolism. Cell. 2010;142:24–38.
- Coad J, Pedley K. Iron deficiency and iron deficiency anemia in women. Scand J Clin Lab Invest. 2014;244:82–89.
- Hayashi F, Yoshiike N, Yoshita K, et al. Trends in the prevalence of anaemia in Japanese adult women, 1989–2003. Public Health Nutr. 2008;11:252–257.
- Kusumi E, Shoji M, Endou S, et al. Prevalence of anemia among healthy women in 2 metropolitan areas of Japan. Int J Hematol. 2006;84:217–279.
- Lee GR. Iron deficiency and iron deficiency anemia. In: GR L, Foerster J, Lukens J, et al., editors. Wintrobe’s clinical hematology. 10th ed. Baltimore: Williams and Wilkins; 1999. p. 979–1010.
- Algarín C, Nelson CA, Peirano P, et al. Iron-deficiency anemia in infancy and poorer cognitive inhibitory control at age 10 years. Dev Med Child Neurol. 2013;55:453–458.
- Hallberg L, Bjørn-Rasmussen E, Howard L, et al. Dietary heme iron absorption. A discussion of possible mechanisms for the absorption-promoting effect of meat and for the regulation of iron absorption. Scand J Gastroenterol. 1979;14:769–779.
- Terato K, Hiramatsu Y, Yoshino Y. Studies on iron absorption. II. Transport mechanism of low molecular iron chelate in rat intestine. Am J Dig Dis. 1973;18:129–134.
- Björn-Rasmussen E, Hallberg L, Isaksson B, et al. Food iron absorption in man. Applications of the two-pool extrinsic tag method to measure heme and nonheme iron absorption from the whole diet. J Clin Invest. 1974;53:247–255.
- Fukami K, Kawai K, Takeuchi S, et al. Effect of water content on the glass transition temperature of calcium maltobionate and its application to the characterization of non-Arrhenius viscosity behavior. Food Biophys. 2016;11:410–416.
- Suehiro D, Okada M, Fukami K, et al. The effect maltobionic acid calcium has in increasing calcium absorption in rats. Luminacoid Res. 2017;21:1–7.
- Suehiro D, Okada M, Fukami K, et al. Maltobionic acid enhances intestinal absorption of calcium and magnesium in rats. Biosci Biotechnol Biochem. 2019;83:1766–1773.
- Fukami K, Suehiro D, Takara T. Safety evaluation of long-term intake and effects on bone mineral density of corn syrup solids containing maltobionic acid (maltobionic acid calcium salt) in healthy Japanese women-a randomized double-blind placebo-controlled study. Jpn Pharmacol Ther. 2019;46:229–245.
- Fukami K, Takeuchi S, Fukujyu T, et al. Water sorption, glass transition, and freeze-concentrated glass-like transition properties of calcium maltobionate–maltose mixtures. J Therm Anal Cal. 2019;135:2775–2781.
- Oshiro I, Takenaka T, Maeda J. New method for hemoglobin determination by using sodium lauryl sulfate (SLS). Clin Biochem. 1982;15:83–88.
- Beale RN, Bostrom JO, Taylor RF. Improved rapid methods for the determination of iron content and binding capacity of serum. J Clin Pathol. 1962;15:156–160.
- Ramsay WN. The determination of the total iron-binding capacity of serum. Clin Chim Acta. 1997;259:25–30.
- Pantopoulos K, Porwal SK, Tartakoff A, et al. Mechanisms of mammalian iron homeostasis. Biochemistry. 2012;51:5705–5724.
- Bainton DF, Finch CA. The diagnosis of iron deficiency anemia. Am J Med. 1964;37:62–70.
- Huebers HA, Csiba E, Josephson B, et al. Iron absorption in the iron-deficient rat. Blut. 1990;60:345–351.
- Gunshin H, Mackenzie B, Berger UV, et al. Cloning and characterization of a mammalian proton-coupled metal-ion transporter. Nature. 1997;388:482–488.
- Ward FW, Coates ME. Gastrointestinal pH measurement in rats: influence of the microbial flora, diet and fasting. Lab Anim. 1987;21:216–222.
- Cook JD. Adaptation in iron metabolism. Am J Clin Nutr. 1990;51:301–308.
- Asvarujanon P, Ishizuka S, Hara H. Promotive effects of non-digestible disaccharides on rat mineral absorption depend on the type of saccharide. Nutrition. 2005;21:1025–1035.
- Ohta A, Ohtsuki M, Baba S, et al. Effects of fructooligosaccharides on the absorption of iron, calcium and magnesium in iron-deficient anemic rats. J Nutr Sci Vitaminol. 1995;41:281–291.
- Laparra JM, Díez-Municio M, Herrero M, et al. Structural differences of prebiotic oligosaccharides influence their capability to enhance iron absorption in deficient rats. Food Funct. 2014;5:2430–2437.
- Sakai K, Ohta A, Shiga K, et al. The cecum and dietary short-chain fructooligosaccharides are involved in preventing postgastrectomy anemia in rats. J Nutr. 2000;130:1608–1612.
- Shiga K, Nishimukai M, Tomita F, et al. Ingestion of difructose anhydride III, a non-digestible disaccharide, prevents gastrectomy-induced iron malabsorption and anemia in rats. Nutrition. 2006;22:786–793.
- Nemeth E, Tuttle MS, Powelson J, et al. Hepcidin regulates cellular iron efflux by binding to ferroportin and inducing its internalization. Science. 2004;306:2090–2093.
- Ganz T, Nemeth E. Hepcidin and iron homeostasis. Biochim Biophysi Acta. 2012;1823:1434–1443.
- Frazer DM, Wilkins SJ, Becker EM, et al. Hepcidin expression inversely correlates with the expression of duodenal iron transporters and iron absorption in rats. Gastroenterology. 2002;123:835–844.