ABSTRACT
Bacillus ComQ participates in the biosynthesis of a quorum-sensing signaling molecule (ComX pheromone) through catalyzing the prenylation at a Trp residue of the precursor peptide (pre-ComX) with geranyl diphosphate (C10 type) or farnesyl diphosphate (C15 type). We hypothesized that several residues specifically conserved among either type of ComQs are important for their substrate specificities. Using a simple bioassay, we revealed that Phe63, Asn186, and Gly190 in ComQRO-E-2 (C10 type) were nondisplaceable to Ser63, Gly186, and Val190, the corresponding residues in the C15-type ComQ, respectively. A three-dimensional model suggested that the 186th and 190th residues are involved in the pre-ComX binding. In vitro analysis showed that substitution of Phe63 with Ser in ComQRO-E-2 significantly reduced the geranylation activity but substantially enhanced the farnesylation activity, whereas substitution of Ser63 with Phe in ComQ168 (C15 type) reduced the farnesylation activity. Therefore, the 63rd residue was found to be significant for the prenyl-substrate preference.
Abbreviations: GPP: geranyl diphosphate; FPP: farnesyl diphosphate; IPP: isopentenyl diphosphate; GGPP: geranylgeranyl diphosphate; FARM: first aspartate-rich motif; SARM: second aspartate-rich motif; β-Gal: β-galactosidase; TBABG: tryptose blood agar base supplemented with glucose; X-gal: 5-bromo-4-chloro-3-indolyl-β-D-galactoside
GRAPHICAL ABSTRACT
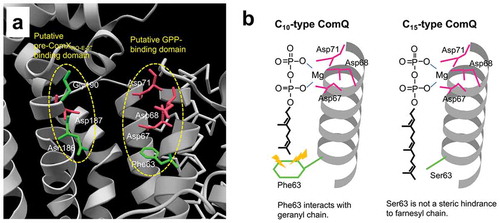
(a) The putative catalytic center of ComQRO-E-2 (C10 type) (b) Proposed mechanism of accommodation of prenyl substrate in each type of ComQs.
Bacterial cells communicate with their cognate cells through quorum-sensing systems to coordinate their physiological activities depending on their cell density. They individually secrete and share a quorum-sensing signaling molecule, called a bacterial pheromone (or autoinducer, or quormone). The released pheromones are sensed by the cognate cells, thereby measuring their own density. When the pheromone concentration reaches a certain threshold with the increase in cell density, the corresponding cells synchronously induce the expression of associate genes for mediating phenomena, e.g. bioluminescence, biofilm formation, genetic competence, and production of antibiotics, biosurfactants, and virulence factors [Citation1–Citation3].
A Gram-positive bacterium, Bacillus subtilis, together with related species, possesses a regulatory system encoded on the comQXPA gene cluster to mediate quorum-sensing responses [Citation3–Citation7] ()). ComQ is involved in the biosynthesis of an oligopeptide-type bacterial pheromone called the ComX pheromone. The comX gene is initially translated as a precursor peptide, pre-ComX, which is converted to the ComX pheromone [Citation5]. ComP is a membrane-bound receptor kinase to detect the ComX pheromone, ComA is a response regulator for ComP, and both comprise a two-component regulatory system. Accumulation of the ComX pheromone activates the autophosphorylation of ComP, which subsequently phosphorylates ComA in the cytoplasm, leading to induction of many genes involved in the quorum-sensing responses [Citation5,Citation8,Citation9]. In B. subtilis, the phosphorylated ComA induces the srfA operon for the biosynthesis of surfactin, a major lipopeptide biosurfactant, and indirectly activates the acquisition of genetic competence through stabilization of the ComK competence transcription-factor by ComS encoded within the srfA operon [Citation10–Citation12]. The production of surfactin also promotes biofilm formation by affecting the neighboring B. subtilis cells [Citation2,Citation3]. The degQ gene located adjacently upstream of comQ is a direct target of the phosphorylated ComA, and degQ encodes a pleiotropic regulator of degradation enzymes [Citation10,Citation13]. DegQ contributes to the function of the DegSU two-component system, which regulates cellular processes including degradative enzyme production, genetic competence, biofilm formation, and swarming motility, by interacting with the DegSU complex to promote the accumulation of the phosphorylated DegU [Citation14]. The phosphorylated DegU response-regulator directly activates the pgsB operon for the poly-γ-glutamate biosynthesis and represses the srfA operon whereas the unphosphorylated DegU, together with ComK, stimulates the comK transcription [Citation15–Citation17]. Interestingly, B. subtilis strain 168, a domesticated laboratory strain, possesses a mutation in the degQ promoter causing decrease in the degQ expression, which enhances the genetic competence and impairs the poly-γ-glutamate production [Citation16,Citation18] ()).
Figure 1. Bacillus ComQXPA quorum-sensing system.
(a) Organization of the comQXPA gene cluster in B. subtilis strain 168. The cluster members and their neighboring genes are indicated by large open arrows, and the promoters and hairpin structures likely functioning as transcription terminators are indicated by bent arrows and stem loops, respectively [Citation6,Citation7]. It was reported that three transcripts, respectively composed of comQXPAyuxO, comQXPA, and comQXP, were obtained by the transcription from the comQ promoter [Citation7]. aa, amino acids. (b) Scheme of quorum-sensing responses through the ComQXPA system. Pre-ComX is converted to the ComX pheromone via prenyl modification by ComQ. ComP detects the secreted ComX pheromone to activate ComA by phosphoryl transfer. The phosphorylated ComA induces the expression of associate genes for mediating phenomena such as surfactin biosynthesis, acquisition of genetic competence, and poly-γ-glutamate production.
![Figure 1. Bacillus ComQXPA quorum-sensing system.(a) Organization of the comQXPA gene cluster in B. subtilis strain 168. The cluster members and their neighboring genes are indicated by large open arrows, and the promoters and hairpin structures likely functioning as transcription terminators are indicated by bent arrows and stem loops, respectively [Citation6,Citation7]. It was reported that three transcripts, respectively composed of comQXPAyuxO, comQXPA, and comQXP, were obtained by the transcription from the comQ promoter [Citation7]. aa, amino acids. (b) Scheme of quorum-sensing responses through the ComQXPA system. Pre-ComX is converted to the ComX pheromone via prenyl modification by ComQ. ComP detects the secreted ComX pheromone to activate ComA by phosphoryl transfer. The phosphorylated ComA induces the expression of associate genes for mediating phenomena such as surfactin biosynthesis, acquisition of genetic competence, and poly-γ-glutamate production.](/cms/asset/22447e74-3239-4af4-bbbd-f88593cba805/tbbb_a_1685371_f0001_b.gif)
The comQX genes and 5ʹ-part of the comP gene are not highly conserved among B. subtilis and Bacillus mojavensis isolates [Citation19–Citation21]. Consequently, the C-terminus of pre-ComX exhibits polymorphism, except that only a Trp residue is conserved (). The Trp residue is post-translationally prenylated by ComQ to form a characteristic tricyclic structure, and the mature ComX pheromone is generated by cleavage processing. The prenylation of pre-ComXs in various B. subtilis and B. mojavensis isolates is classified into two groups: geranylation (C10 type) and farnesylation (C15 type). These prenylations are attributed to the specificity of the respective ComQs to prenyl donors, i.e. geranyl diphosphate (GPP) and farnesyl diphosphate (FPP) [Citation22–Citation24]. For example, B. subtilis strain RO-E-2’s ComQ (ComQRO-E-2) uses GPP for the geranylation of this strain’s pre-ComX (pre-ComXRO-E-2) [Citation25], while ComQs from B. subtilis strain 168 and B. mojavensis strain RO-C-2 (ComQ168 and ComQRO-C-2) use FPP for the farnesylation of their target pre-ComXs (pre-ComX168 and pre-ComXRO-C-2, respectively) [Citation26]. Such different chain lengths of the prenyl modifications confer the structural diversity of ComX pheromones as well as the difference in the amino acid sequence (). It should be noted that the length of a lipophilic side chain of quorum-sensing pheromones is generally responsible for their group-specific biological activities to the corresponding bacteria as a quorum-sensing pheromone [Citation1,Citation21,Citation23,Citation27].
Figure 2. Comparison of amino acid sequences of pre-ComXs and structure of the prenyl-modified Trp residue of ComX pheromones.
(a) Sequence alignment of amino acid sequences of pre-ComXs derived from three B. subtilis strains (RO-E-2, RS-B-1, and 168) and two B. mojavensis strains (RO-B-2 and RO-C-2). The conserved Trp residue that is subjected to prenyl modification is indicated by a dagger symbol. The Trp residues of strains RO-E-2, RS-B1, and RO-B-2 are modified with geranylation (C10-modifiction), whereas those of strains 168 and RO-C-2 are modified with farnesylation (C15-modification). Each peptide sequence of the secreted ComX pheromones is underlined [Citation24,Citation26]. The accession numbers of the respective pre-ComXs are AAL67740 (RO-E-2), AAF82173 (RS-B-1), AAL67731 (RO-B-2), AIY94492 (168), and AAL67728 (RO-C-2). (b) Chemical structure of the Trp residue with prenyl modification (geranylation or farnesylation) of ComX pheromones.
![Figure 2. Comparison of amino acid sequences of pre-ComXs and structure of the prenyl-modified Trp residue of ComX pheromones.(a) Sequence alignment of amino acid sequences of pre-ComXs derived from three B. subtilis strains (RO-E-2, RS-B-1, and 168) and two B. mojavensis strains (RO-B-2 and RO-C-2). The conserved Trp residue that is subjected to prenyl modification is indicated by a dagger symbol. The Trp residues of strains RO-E-2, RS-B1, and RO-B-2 are modified with geranylation (C10-modifiction), whereas those of strains 168 and RO-C-2 are modified with farnesylation (C15-modification). Each peptide sequence of the secreted ComX pheromones is underlined [Citation24,Citation26]. The accession numbers of the respective pre-ComXs are AAL67740 (RO-E-2), AAF82173 (RS-B-1), AAL67731 (RO-B-2), AIY94492 (168), and AAL67728 (RO-C-2). (b) Chemical structure of the Trp residue with prenyl modification (geranylation or farnesylation) of ComX pheromones.](/cms/asset/fe9109dc-d0c6-4ace-bc88-86510e369f53/tbbb_a_1685371_f0002_b.gif)
ComQ shows the sequence similarity to trans-type prenyl diphosphate synthases [Citation22,Citation23], which catalyze the consecutive condensation of isopentenyl diphosphate (IPP) with allylic diphosphate, e.g. GPP and FPP, to produce elongated prenyl diphosphates, e.g. FPP and geranylgeranyl diphosphate (GGPP, C20). In these synthases, two aspartate-rich motifs are crucial for the binding of the two substrates; the first aspartate-rich motif (FARM) is for allylic diphosphate, and the second aspartate-rich motif (SARM) is for IPP. The carboxyl groups of Asp residues in each motif bind to the diphosphate moiety of each substrate via divalent cations [Citation28]. ComQ also possesses the FARM, whereas the region corresponding to the SARM is less conserved and thus is designated as a pseudo-SARM [Citation29] (). A previous study on the in vitro geranylation activity of ComQRO-E-2 showed that Mg2+ was required for the activity [Citation25]. Thus, the FARM in ComQ was estimated to serve as a binding site of prenyl diphosphate (GPP or FPP) via Mg2+ similarly to trans-type prenyl diphosphate synthases. By contrast, the pseudo-SARM in ComQ was inferred to function in the binding of pre-ComX instead of IPP [Citation29]. However, the precise recognition mechanisms for both substrates remained to be elucidated.
Figure 3. Comparison of amino acid sequences of ComQs derived from three B. subtilis strains (RO-E-2, RS-B-1, and 168) and two B. mojavensis strains (RO-B-2 and RO-C-2).
The ComQs from the three strains (RO-E-2, RS-B1, and RO-B-2) are the C10 type and those from the two strains (168 and RO-C-2) are the C15 type. The FARM and pseudo-SARM are underlined, and four Asp residues in these two motifs are indicated by number signs. Twenty-five amino acid residues that are conserved among either C10- or C15-type ComQs are indicated by asterisks, and the positions of 10 amino acid residues, which were substituted in the ComQRO-E-2 point mutants for the in vivo bioassay, are indicated by boldface type. The accession numbers of the ComQs are AAL67739 (RO-E-2), AAF82172 (RS-B-1), AAL67730 (RO-B-2), AIY94493 (168), and AAL67727 (RO-C-2).
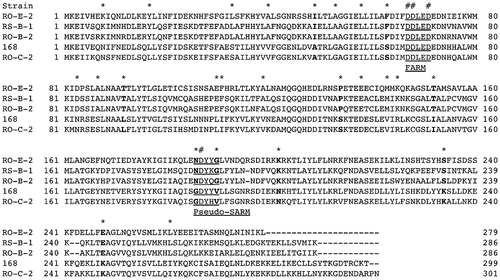
In this study, we focused on type-specific amino acid residues in ComQs based on a multiple-sequence alignment of five ComQ variants. To investigate whether those residues are important for the specificity to each substrate or the catalytic activity, we constructed a three-dimensional model of ComQRO-E-2 (C10 type) based on a known GGPP synthase, and the spatial arrangement of the relevant residues was estimated. Then we evaluated ComQRO-E-2 mutants with each single substitution of those residues through an in vivo bioassay system, together with the wild-type ComQRO-E-2 and ComQ168 (C15 type). In this system, the Escherichia coli transformant carrying each of the wild-type and mutated comQX gene clusters was co-cultivated with two B. subtilis tester strains that respond to the ComXRO-E-2 pheromone (C10 modified) or ComX168 pheromone (C15 modified), respectively. Furthermore, we measured the in vitro prenylation activity of ComQ mutants as well as that of the wild types.
Materials and methods
B. subtilis tester strains
B. subtilis strain BD3020 was constructed from a derivative of strain 168 in the previous study [Citation20,Citation21,Citation23], in which the comQXP168 genes were replaced by the comQXPRO-E-2 genes followed by the comQRO-E-2 disruption by plasmid insertion, and the lacZ gene was integrated into the srfA operon on the chromosome (). Thus, this strain is incapable of producing the ComX168 pheromone and able to induce the β-galactosidase (β-Gal) activity in response to the exogenously added ComXRO-E-2 pheromone. Strain BD3020 and a plasmid pED413 (mentioned below) were kindly provided by Prof. D. Dubnau from Rutgers University.
Table 1. B. subtilis strains used in this study.
To detect the extraneous ComX168 pheromone, B. subtilis strain FU1086 was constructed as follows. A DNA fragment corresponding to a region around the srfA promoter (bases −313 to 406; base 1 is the transcription start base) [Citation10] was amplified by PCR with the genomic DNA of strain 168 () and a primer pair of PsrfA_XF/Psrf_BR (Table S1), followed by trimming with XbaI and BamHI digestion. It was then cloned into the pCRE-test2 vector at the same restriction sites [Citation30]. The resulting plasmid, pCRE-PsrfA (Table S2), was linearized by PstI digestion and then integrated into the amyE locus of strain 1A832, a upp gene disruptant [Citation31] (, provided by the Bacillus Genetic Stock Center, Columbus, OH, USA), to obtain chloramphenicol resistance (5 µg/mL) on a plate of tryptose blood agar base (Difco, Becton, Dickinson and Company, Franklin Lakes, NJ, USA) supplemented with 0.18% glucose (TBABG), which resulted in strain FU1085 carrying the srfA promoter-lacZ fusion (). In-frame deletion of the comQX168 genes from this strain’s chromosome was performed according to the method previously reported [Citation31]. The flanking regions upstream of comQ168 and downstream of comX168 were respectively amplified by PCR with the genomic DNA of strain 168 and the primer pairs of comQ_P1/comQ_P2 and comX_P3/comX_P4 (Table S1). The upp-K7 cassette was amplified by PCR with a pUC19-upp-phleo plasmid [Citation31] as the template and a primer pair of Phleo3/Phleo5 (Table S1). These three fragments were combined by the joining PCR, and the resultant fragment was used for transformation of strain FU1085 to obtain phleomycin resistance (5 µg/mL) on the TBABG plate. After checking the chloramphenicol resistance (5 µg/mL) and 5-fluorouracil sensitivity (25 µM) of the obtained strain, the upp-K7 cassette of this strain was removed through pop-out recombination; since the RecA activation via ComK was impaired by the comQX168 disruption [Citation11,Citation12,Citation32], the RecA activity was induced via LexA inactivation by UV radiation [Citation32,Citation33] to the culture in a minimal medium [Citation31] on a clean bench (MCV-B161F, PHC Holdings Corporation, Tokyo, Japan), followed by cultivation for another 1 h. The cassette-lacking clone was selected on an agar plate of minimal medium containing 25 µM 5-fluorouracil, and its phleomycin sensitivity was checked to obtain strain FU1086 (). Correct cassette integration and pop-out event were also confirmed by PCR with the genomic DNA of each clone as the template and primer pairs of comQ_P1/comX_P4. By this in-frame deletion, the 5ʹ-short part of the comQ168 coding sequence was fused to the 3ʹ-short part of the comX168 coding sequence, which resulted in a fusion of the N-terminal 66 amino acid residues of ComQ168 with the C-terminal 10 amino acid residues of ComX168 having no catalytic function, together with the intact ComPA168 two-component system indispensable for detection of the ComX168 pheromone.
Construction of co-expression systems in E. coli
To construct plasmids for co-expression of the comQXRO-E-2 gene cluster and for co-expression of the comQX168 gene cluster in the E. coli cells, DNA fragments covering the comQX genes of each strain were amplified by PCR with the plasmid pED413 [Citation23] carrying the comQXRO-E-2 genes and strain 168’s genomic DNA as the template and primer pairs of uni_comQ_BXF/ROE2_comX_HSR and uni_comQ_XF/168_comX_SR (Table S1), respectively. The comQXRO-E-2 fragment was cloned into the pUC18 vector (TaKaRa Bio, Shiga, Japan) that had been treated with XbaI and SphI using an In-Fusion HD cloning kit (Clontech, TaKaRa Bio) to produce a plasmid pUC-ROE2-comQX, and the comQX168 fragment was cloned into a pCR2.1 TOPO vector (Invitrogen, Thermo Fisher Scientific, Waltham, MA, USA) by TA cloning to produce a plasmid pCR-168-comQX. In these two plasmids, the comQX genes of each strain are under the control of the lac promoter (Table S2).
To construct plasmids for co-expression of each mutated comQRO-E-2 gene with the wild-type comXRO-E-2 gene in the E. coli cells, site-directed mutagenesis was applied to the comQXRO-E-2 fragment through a two-step overlapping PCR method as follows. The 5ʹ-parts of comQRO-E-2 were amplified by PCR with pUC-ROE2-comQX DNA as the template, oligonucleotide uni_comQ_XF as the forward primer, and oligonucleotides I49A_R, F63A_R, F63I_R, F63S_R, F63V_R, T92L_R, P134S_R, T152I_R, N186G_R, G190V_R, K202N_R, S234K_R, and E248K_R as the respective reverse primers (Table S1). Similarly, the 3ʹ-parts of comQRO-E-2 with the entire comXRO-E-2 were amplified using oligonucleotides I49A_F, F63A_F, F63I_F, F63S_F, F63V_F, T92L_F, P134S_F, T152I_F, N186G_F, G190V_F, K202N_F, S234K_F, and E248K_F as the respective forward primers and oligonucleotide ROE2_comX_HSR as the reverse primer (Table S1). Each pair of mutation-introduced PCR products was combined by the joining PCR, and the resultant fragments were individually cloned into the pUC18 vector using the In-Fusion HD cloning kit as in the case of pUC-ROE2-comQX construction to yield 13 plasmids (pUC-ROE2-comQX_I49A, _F63A, _F63I, _F63S, _F63V, _T92L, _P134S, _T152I, _N186G, _G190V, _K202N, _S234K, and _E248K) for the production of each ComQRO-E-2 mutant carrying the indicated single substitution together with the wild-type pre-ComXRO-E-2 (Table S2).
Bioassay of the ComQRO-E-2 mutants
B. subtilis tester strains BD3020 and FU1086 were grown at 37ºC overnight on TBABG plates, which contained both chloramphenicol (5 μg/mL) and tetracycline (10 µg/mL) or only chloramphenicol (5 μg/mL), respectively. E. coli strain DH5α was transformed with each of the 15 plasmids constructed as described above and grown at 37ºC overnight on an LB agar plate containing ampicillin (50 μg/mL). Subsequently, each E. coli transformant and the two B. subtilis tester strains were inoculated on one TBABG plate containing 5-bromo-4-chloro-3-indolyl-β-D-galactoside (X-gal) (80 μg/mL) and grown at 37ºC overnight to form three compartments of the individual strain’s cells. To clarify the blue coloration caused by the induced β-Gal activity, the plates with the grown cells were held at room temperature for a few days (Figure S1).
Preparation of the wild-type and mutated ComQs
A plasmid pET15-ROE2-comQ for the overexpression of ComQRO-E-2 in the E. coli cells was constructed in the previous study [Citation25]. For site-directed mutagenesis into the comQRO-E-2 in pET15-ROE2-comQ, a KOD-Plus-Mutagenesis kit (Toyobo Life Science, Osaka, Japan) was used with primer pairs of ROE2_F63S_F/ROE2_F63S_R and ROE2_D68E_F/ROE2_D68E_R (Table S1), which resulted in two plasmids of pET15-ROE2-comQ_F63S and _D68E for the overexpression of [F63S] and [D68E]ComQRO-E-2s (Table S2). The comQ168 coding region was amplified by PCR with the genomic DNA of strain 168 and a primer pair of 168_comQ_F/168_comQ_R (Table S1), which was then cloned into the pET-15b vector (Novagen, Sigma-Aldrich, Merck, Darmstadt, Germany) that had been treated with NcoI using the In-Fusion HD cloning kit to yield a plasmid pET15-168-comQ for the ComQ168 overexpression. Site-directed mutagenesis into the comQ168 in pET15-168-comQ was performed using the KOD-Plus-Mutagenesis kit with a primer pair of 168_S63F_F/168_S63F_R (Table S1), and the resultant plasmid pET15-168-comQ_S63F was used for the [S63F]ComQ168 overexpression (Table S2).
The wild-type and mutated ComQs were prepared according to the procedure previously reported [Citation25]. Cells of E. coli strain BL21(DE3) (Novagen, Sigma-Aldrich, Merck), transformed with each of the pET-15b-based plasmids described in the preceding section, were grown in a M9 minimal medium supplemented with ampicillin (400 µg/mL) and a mixture of amino acids [Citation25] at 37ºC until an optical density at 620 nm reached 0.6. After isopropyl-β-D-thiogalactopyranoside was added to a final concentration of 1 mM, the cells were cultivated for another 4 h. The cultured cells were harvested by centrifugation, resuspended in 25 mM Tris-HCl buffer (pH 7.4) containing 0.1 mM MgCl2 and 0.1 mM EGTA, and then disrupted by sonication. After ultracentrifugation of the slurry, the precipitate was collected and resuspended in the same buffer except for the addition of n-dodecyl-β-D-maltoside at 2 mg/mL, which was used as the crude enzyme. The production of each ComQ was confirmed by sodium dodecyl sulfate-polyacrylamide gel electrophoresis (Figure S2).
In vitro prenylation reaction and detection of prenylated peptides
The synthesis of pre-ComXs, prenylation of these peptides using wild-type and mutated ComQs, and detection of the prenylated peptides were performed according to the methods previously reported [Citation25]. In 50 µL of 50 mM N-Tris(hydroxymethyl)methyl-3-aminopropanesulfonic acid (TAPS) -NaOH buffer (pH 8.5) containing 5 mM MgCl2, 2.5 pmol of pre-ComX ([13C2]pre-ComXRO-E-2 or pre-ComX168), 10 pmol of prenyl diphosphate (GPP or FPP, Sigma-Aldrich, Merck), and each ComQ suspension (7.5 mL broth equivalent) were incubated at 37ºC for 2 h. After the addition of 200 µL of CH3CN, the reaction mixture was centrifuged, and 10 µL of the obtained supernatant was combined with 30 µL of the solution containing 2.5 pmol of the synthetic Ala-ComXRO-E-2 pheromone as the internal standard, which was subjected to LC-MS analysis (HCTplus, Brucker Daltonics, Bremen, Germany). The amount of prenylated peptide was calculated from the integration of the peak area (Figure S3).
Results and discussion
Selection of candidate residues responsible for prenyl donor selectivity in ComQ
A multiple-sequence alignment was performed among five ComQs, which were derived from three B. subtilis strains (RO-E-2, RS-B-1, and 168) and two B. mojavensis strains (RO-B-2 and RO-C-2) (). The three strains, RO-E-2, RS-B-1, and RO-B-2, produce the C10-modified ComX pheromones, and the two strains, 168 and RO-C-2, produce the C15-modified ComX pheromones [Citation5,Citation23,Citation24,Citation26]. Strains RO-E-2, RS-B-1, RO-B-2 and RO-C-2 are undomesticated isolates from Mojave desert, Rosamond, CA, USA [Citation34]. The alignment showed that the region including the FARM is relatively well conserved among the five ComQs, suggesting that this region plays a central role in the prenyl transfer reaction common to all ComQs. Notably, no species-specific conservation was found in the five ComQs; however, 25 amino acid residues were found to be type-specifically conserved. They are individually conserved among either the C10 or C15 type of ComQs but are different between the two types. It was assumed that some of these residues play a critical role in the specific recognition of the chain length of the prenyl donor. We focused on 10 such residues to elucidate their functions in the substrate specificity because those residues are quite different in bulkiness or charge between the two types of ComQs.
Prediction of the spatial arrangement of candidate residues in ComQRO-E-2
To explore the spatial arrangement of the 10 residues and essential regions for the ComQ activity, a three-dimensional model of ComQRO-E-2 (C10 type) was constructed through SWISS-MODEL homology modeling based on a crystal structure of the GGPP synthase from Thermus thermophilus strain HB8 (PDB ID: 1WMW) [Citation35,Citation36] ()). The model structure of ComQRO-E-2 showed that the FARM residues, including three Asp residues (Asp67, Asp68, and Asp71), and the pseudo-SARM residues, including Asn186 and Gly190 residues of the 10 residues as well as one Asp residue (Asp187), are arranged to be proximal to each other ()). The three Asp residues in the FARM were predicted to bind to the diphosphate moiety of GPP via the Mg2+ bridge [Citation22,Citation28]. A previous study revealed that the Asn186 and Asp187 residues were necessary for the in vitro geranylation activity and suggested that the pseudo-SARM serves as the pre-ComXRO-E-2 binding site [Citation29]. Therefore, the residues in the FARM and the pseudo-SARM and their peripheral residues probably form the binding domains for the respective substrates to be assembled into the major components of the catalytic center. In addition, another of the 10 residues, Phe63, is situated at the 4th position before the FARM. In trans-type FPP synthases, an aromatic residue at the 5th position or two aromatic residues at the 4th and 5th positions before the FARM are critical for the determination of the product chain length by direct interaction with the ω-terminus of the farnesyl chain of FPP [Citation28]. Hence, the Phe63 residue in C10-type ComQs was assumed to interact with the geranyl chain of GPP. The other seven candidate residues appeared apart from the two motifs, and thus they were not likely to have a catalytic function.
Figure 4. Three-dimensional structural model of ComQRO-E-2.
(a) Overall structure calculated through the SWISS-MODEL homology modeling [Citation36] based on a dimer structure of the T. thermophilus strain HB8 GGPP synthase (PDB ID: 1WMW). (b) Magnified view of a region around the putative catalytic center. Four Asp residues in the FARM and pseudo-SARM are depicted by pink sticks. The 10 amino acid residues of interest are depicted by green sticks. The putative GPP-binding domain and the putative pre-ComXRO-E-2-binding domain are indicated by yellow dashed ovals.
![Figure 4. Three-dimensional structural model of ComQRO-E-2.(a) Overall structure calculated through the SWISS-MODEL homology modeling [Citation36] based on a dimer structure of the T. thermophilus strain HB8 GGPP synthase (PDB ID: 1WMW). (b) Magnified view of a region around the putative catalytic center. Four Asp residues in the FARM and pseudo-SARM are depicted by pink sticks. The 10 amino acid residues of interest are depicted by green sticks. The putative GPP-binding domain and the putative pre-ComXRO-E-2-binding domain are indicated by yellow dashed ovals.](/cms/asset/f7040b85-b90d-4dbe-8535-93aef3879c90/tbbb_a_1685371_f0004_c.jpg)
Construction and in vivo analysis of ComQRO-E-2 mutants
To examine whether each of the 10 C10-type-specific residues of ComQRO-E-2 is displaceable to the corresponding residue in the C15-type ComQ or whether the prenyl donor specificity of ComQRO-E-2 can be changed by either of such substitutions, 10 ComQRO-E-2 mutants ([I49A], [F63S], [T92L], [P134S], [T152I], [N186G], [G190V], [K202N], [S234K], and [E248K]ComQRO-E-2s), were individually produced together with the wild-type pre-ComXRO-E-2 in the E. coli cells by introducing the corresponding mutated comQXRO-E-2 gene cluster. We also prepared the E. coli transformant carrying each of the wild-type comQXRO-E-2 and comQX168 gene clusters.
To evaluate the in vivo activity of ComQRO-E-2 mutants, we established a simple tri-partitioned bioassay system. In this system, each E. coli transformant carrying the wild-type or mutated comQX gene cluster was co-cultivated with two B. subtilis tester strains (BD3020 and FU1086) on a solid medium containing X-gal. Strains BD3020 and FU1086 were constructed to be able to induce the β-Gal activity in response to extraneous ComXRO-E-2 and ComX168 pheromones, respectively [Citation10,Citation21,Citation23] (, ). When the E. coli transformant carrying the wild-type comQXRO-E-2 gene cluster was co-cultivated with the two tester strains, the BD3020 cells near the E. coli cells responded to the ComXRO-E-2 pheromone secreted, resulting in blue coloration, whereas no coloration was observed in the FU1086 cells. By contrast, only FU1086 cells showed blue coloration in response to the ComX168 pheromone when the E. coli transformant carrying the wild-type comQX168 gene cluster was co-cultivated ( and Figure S1). We confirmed that, in the absence of the specific pheromone, the β-Gal activity under the control of the srfA promoter was sufficiently repressed when each tester strain was cultivated on the TBABG plate, but not on the minimal medium plate [Citation31], and we found that the blue coloration by the induced β-Gal activity became clear when X-gal was added to the TBABG plate at high concentration (80 µg/mL) (data not shown). Thus, all bioassay results presented here were obtained under this medium condition. In the previous study, for detection of the ComX pheromone, the sterile pheromone-containing supernatant was prepared from the liquid culture of the E. coli producer strain, and then the supernatant was added to the liquid culture of each of the B. subtilis tester strains such as BD3020, followed by measurement of the β-Gal activity [Citation20]. Compared to that method, our bioassay system can be easily performed in a short time although the induced β-Gal activity is not quantitatively evaluated.
Figure 5. Scheme of production of the ComX pheromone in the E. coli cells and detection of the heterologously produced ComX168 and ComXRO-E-2 pheromones by the two B. subtilis tester strains.
Both tester strains were derived from strain 168. Strain FU1086 lacks the comQX168 genes but it is able to respond to the extraneous ComX168 pheromone through the ComPA168 two-component system to induce the lacZ expression under the control of the srfA promoter. Strain BD3020 does not possess either comQ168 or comQRO-E-2, and the comXP168 genes are replaced with comXPRO-E-2 in this strain. Strain BD3020 is able to detect the extraneous ComXRO-E-2 pheromone by ComPRO-E-2, which phosphorylates ComA168 leading to induction of the lacZ gene integrated into the srfA operon. Production of pre-ComXRO-E-2 without prenyl modification in the BD3020 cells does not affect the pheromone detection [Citation21,Citation23].
![Figure 5. Scheme of production of the ComX pheromone in the E. coli cells and detection of the heterologously produced ComX168 and ComXRO-E-2 pheromones by the two B. subtilis tester strains.Both tester strains were derived from strain 168. Strain FU1086 lacks the comQX168 genes but it is able to respond to the extraneous ComX168 pheromone through the ComPA168 two-component system to induce the lacZ expression under the control of the srfA promoter. Strain BD3020 does not possess either comQ168 or comQRO-E-2, and the comXP168 genes are replaced with comXPRO-E-2 in this strain. Strain BD3020 is able to detect the extraneous ComXRO-E-2 pheromone by ComPRO-E-2, which phosphorylates ComA168 leading to induction of the lacZ gene integrated into the srfA operon. Production of pre-ComXRO-E-2 without prenyl modification in the BD3020 cells does not affect the pheromone detection [Citation21,Citation23].](/cms/asset/b1c9ecfa-e048-4020-825c-75708288f687/tbbb_a_1685371_f0005_b.gif)
Table 2. Response of two B. subtilis tester strains to the E. coli transformant expressing each of the wild-type and mutated ComQs together with its target pre-ComX.
Using the bioassay system that we devised, the effect of each substitution on the ComQRO-E-2 activity was investigated. The results showed that seven mutants ([I49A], [T92L], [P134S], [T152I], [K202N], [S234K], and [E248K]ComQRO-E-2s) mediated the blue coloration of strain BD3020 (ComXRO-E-2-specific tester) but not that of strain FU1086 (ComX168-specific tester), which was the same as the wild-type ComQRO-E-2 ( and Figure S1). These results indicated that none of these substitutions affected the production or secretion of the ComXRO-E-2 pheromone and that seven replaced residues are not essential for the catalytic function of ComQRO-E-2. However, when the E. coli transformants corresponding to [F63S], [N186G], and [G190V]ComQRO-E-2s were tested, neither tester strain responded ( and Figure S1). Since the Asn186 and Gly190 residues are included in the pseudo-SARM, each mutation into these residues probably affected the pre-ComXRO-E-2 binding. The previous study showed that each single substitution of the Asn186 residue with Ala and Asp ([N186A] and [N186D]ComQRO-E-2s) significantly reduced the in vitro geranylation activity, which supports the importance of the Asn186 residue in the catalytic activity [Citation29]. By contrast, the previous study also showed that single substitution of the Gly190 residue with either Ala or Asp ([G190A] and [G190D]ComQRO-E-2s) did not severely impair the in vitro geranylation activity [Citation29]. It was supposed that interaction between pre-ComXRO-E-2 and the pseudo-SARM was prevented due to a large steric hindrance of the Val residue at the 190th position.
No activity of [F63S]ComQRO-E-2 suggested that the Phe63 residue played a critical role in the binding of GPP through a π-π interaction. To clarify the Phe63 residue’s role, the in vivo activities of three point-mutants at this position ([F63A], [F63I], and [F63V]ComQRO-E-2s) were also investigated. The results showed that [F63I] and [F63V]ComQRO-E-2s specifically mediated the blue coloration of strain BD3020 but not that of strain FU1086, although their inducing effects appeared slightly lower than that of the wild-type ComQRO-E-2. By contrast, [F63A]ComQRO-E-2 mediated neither tester strain ( and Figure S1). These results indicated that Ile and Val residues are sufficiently functional as alternatives to the Phe residue at the 63rd position in ComQRO-E-2 and strongly suggested that the hydrophobic interaction of GPP with a bulky side chain of the 63rd residue is essential for the catalytic activity of ComQRO-E-2.
Nevertheless, in this in vivo analysis, we could not exclude the possibility that either of the substitutions of Asn186 with Gly, Gly190 with Val, and Phe63 with Ser or Ala reduced the ComQRO-E-2 stability leading to the formation of an inactive inclusion body or the protein degradation in the E. coli cells.
Measurement of in vitro ComQ prenylation activity
For further analysis of the Phe63 residue’s role in the ComQRO-E-2 activity, we prepared recombinant [F63S]ComQRO-E-2 using an E. coli overexpression system as well as the wild-type ComQRO-E-2. We also prepared [D68E]ComQRO-E-2, in which the 2nd Asp residue in the FARM was substituted with Glu (Figure S2). Geranylation and farnesylation activities of the wild-type and mutated ComQRO-E-2s were measured through the in vitro reaction followed by the LC-MS analysis by using chemically synthesized pre-ComXRO-E-2 with GPP or FPP according to the previous study [Citation25] ()). When GPP and pre-ComXRO-E-2 were used as substrates, the wild-type ComQRO-E-2 in the membrane fraction showed significant geranylation activity to produce the C10-modified ComXRO-E-2 C-terminal heptapeptide (from the 52nd to 58th position) predominantly, and the native form of the ComXRO-E-2 pheromone (from the 53rd to 58th position) was given in a low ratio, similar to the previous data [Citation25]. By contrast, no farnesylation activity was detected when FPP was used instead of GPP in the reaction mixture () and Figure S3(a,b)), and no dimethylallylation activity was detected when dimethylallyl diphosphate (C5) was used (data not shown). These results clearly demonstrated that ComQRO-E-2 has strict prenyl-donor specificity.
Figure 6. Measurement of in vitro prenylation activities of the wild-type and mutated ComQs.
Gray bars represent the geranylation activities and white bars the farnesylation activities of the wild-type, [F63S], and [D68E]ComQRO-E-2s (a) and the wild-type and [S63F]ComQ168s (b), which were determined by quantifying the yields of the C10- and C15-modified ComXRO-E-2 C-terminal heptapeptides and the C10- and C15-modified ComX168 C-terminal nonapeptides, respectively. The bars and error bars indicate the mean values and the standard deviations (n = 3). The “n.d.” means that the farnesylation activity was not detected, and the “n/a” means that the farnesylation activity was not measured.
![Figure 6. Measurement of in vitro prenylation activities of the wild-type and mutated ComQs.Gray bars represent the geranylation activities and white bars the farnesylation activities of the wild-type, [F63S], and [D68E]ComQRO-E-2s (a) and the wild-type and [S63F]ComQ168s (b), which were determined by quantifying the yields of the C10- and C15-modified ComXRO-E-2 C-terminal heptapeptides and the C10- and C15-modified ComX168 C-terminal nonapeptides, respectively. The bars and error bars indicate the mean values and the standard deviations (n = 3). The “n.d.” means that the farnesylation activity was not detected, and the “n/a” means that the farnesylation activity was not measured.](/cms/asset/08144f48-f6ba-45a9-80a4-3c2eaffed356/tbbb_a_1685371_f0006_b.gif)
Remarkably, [F63S]ComQRO-E-2 showed substantial farnesylation activity when FPP was used, while its geranylation activity was severely reduced () and Figure S3(c,d)). This result indicated that the 63rd Phe residue is critical for the specific geranylation activity of ComQRO-E-2. It is likely that the substitution of Phe63 with Ser caused a loss of binding affinity to GPP through the hydrophobic interaction and enabled the accommodation of FPP in the widened cavity space ()). Based on the in vitro property of [F63S]ComQRO-E-2, we estimated that, in the in vivo analysis, the observation that [F63S]ComQRO-E-2 did not mediate the β-Gal induction of either tester strain was attributed to the low prenylation activity of this mutant for both GPP and FPP even though the activity for FPP was elevated by the substitution. Alternatively, the produced C15-modified ComXRO-E-2 pheromone might not be recognized by either ComP168 or ComPRO-E-2 although the previous studies showed that the prenyl-chain length of ComX pheromones was an influential determinant for specific bioactivities for the corresponding strains, rather than their amino acid sequences [Citation21,Citation23,Citation27]. At least, it is unlikely that the substitution of the Phe63 residue with Ser caused destabilization of the ComQRO-E-2 protein structure.
When [D68E]ComQRO-E-2 was similarly tested, the prenylation activity of this mutant was found to be severely impaired. Since it was already reported that substitution of either the 1st or 5th Asp residue with Glu in the FARM abolished the in vivo activity of ComQ168 [Citation22], all three Asp residues in the FARM are presumed to be finely coordinated for the prenyl substrate binding (), ), and Figure S3(e)).
We also prepared [S63F]ComQ168, a ComQ168 mutant with the substitution of the Ser residue with Phe at the 4th position before the FARM, and the in vitro prenylation activity of this mutant, as well as that of the wild-type ComQ168, was measured using chemically synthesized pre-ComX168 together with GPP or FPP () and Figure S2). When FPP and pre-ComX168 were used as substrates, the wild-type ComQ168 in the membrane fraction mainly produced the C15-modified ComX168 C-terminal nonapeptide (from the 47th to 55th position) predominantly, and the native form of the ComX168 pheromone (from the 46th to 55th position) was given in a low ratio (Figure S3(f)). When GPP was used as the prenyl donor, the wild-type ComQ168 unexpectedly provided C10-modified ComX168 peptides, though the prenylation activity of the wild-type ComQ168 obtained with FPP was much higher than that with GPP ()). When [S63F]ComQ168 was tested, this mutant significantly reduced the farnesylation activity using FPP as the prenyl donor, whereas its geranylation activity using GPP was comparable to that of the wild-type ComQ168 ()). The Phe residue at the 63rd position probably acted as a steric hindrance, interfering with the farnesyl chain of FPP but not with the shorter chain of GPP.
Taken together, these in vitro data clearly demonstrated that a single substitution at the 4th position before the FARM significantly affected the prenyl donor preference of both C10- and C15-type ComQs.
Conclusion
The present study demonstrated that, in addition to the previously reported Asp67, Asp71, and Asp187 residues, the Phe63, Asp68, Asn186, and Gly190 residues in ComQRO-E-2 are critical for the geranylation (C10 modification) of pre-ComXRO-E-2 [Citation22,Citation29]. Since the Asn186, Asp187, and Gly190 residues are included in the pseudo-SARM, these residues are probably involved in pre-ComXRO-E-2 binding, and a void space around the Gly190 residue is also likely to be required for catalytic activity in ComQRO-E-2. In addition, it was deduced that the Phe63 residue and all of the three Asp residues (Asp67, Asp68, and Asp71) in the FARM play a crucial role in the specific biding to GPP through the hydrophobic interaction and the Mg2+ bridge, respectively. Moreover, it was demonstrated that a single substitution at the 4th position before the FARM in ComQ enables alteration of the prenyl donor preference. Although the prenyl donner specificity of ComQ may not be solely determined by the 63rd residue, this residue’s property is presumably one of the critical determinants. The other critical residues may be identified using high-throughput screening with the bioassay system established in this study. The prenyl-chain length of ComX pheromones was a major determinant for specific bioactivities for the corresponding strains. The specificity of the ComX pheromone’s bioactivities may be exchangeable by a small number of point mutations at the 63rd position and the other unidentified positions in ComQ, leading to alteration of the prenyl-chain length of the ComX pheromone, which might have been actualized in nature.
Author contributions
SS performed the plasmid construction for the co-expression in E. coli and the bioassay experiments. KH constructed the B. subtilis strains. MO prepared the recombinant ComQs and performed the in vitro analysis. KH and MO drafted the research plan and wrote the manuscript with SS.
Correction Statement
This paper is dedicated to the late Dr. Youji Sakagami.
This article has been republished with minor changes. These changes do not impact the academic content of the article.
supplemental_file_BBB-190724_R2.pdf
Download PDF (1.2 MB)Acknowledgments
We are grateful to Ayaka Tamano, Naoki Matsumura, and Hiroyoshi Komatsu for their help with the plasmid construction and the in vivo bioassay.
Disclosure statement
No potential conflict of interest was reported by the authors.
Supplementary material
Supplemental data for this article can be accessed here
References
- Rutherford ST, Bassler BL. Bacterial quorum sensing: its role in virulence and possibilities for its control. Cold Spring Harb Perspect Med. 2012;2:a012427.
- López D, Kolter R. Extracellular signals that define distinct and coexisting cell fates in Bacillus subtilis. FEMS Microbiol Rev. 2010;34:134–149.
- Kalamara M, Spacapan M, Mandic-Mulec I, et al. Social behaviours by Bacillus subtilis: quorum sensing, kin discrimination and beyond. Mol Microbiol. 2018;110:863–878.
- Dogsa I, Choudhary KS, Marsetic Z, et al. ComQXPA quorum sensing systems may not be unique to Bacillus subtilis: a census in prokaryotic genomes. PLoS One. 2014;9:e96122.
- Magnuson R, Solomon J, Grossman AD. Biochemical and genetic characterization of a competence pheromone from B. subtilis. Cell. 1994;77:207–216.
- Weinrauch Y, Penchev R, Dubnau E, et al. A Bacillus subtilis regulatory gene product for genetic competence and sporulation resembles sensor protein members of the bacterial two-component signal-transduction systems. Genes Dev. 1990;4:860–872.
- Ohsawa T, Tsukahara K, Sato T, et al. Superoxide stress decreases expression of srfA through inhibition of transcription of the comQXP quorum-sensing locus in Bacillus subtilis. J Biochem. 2006;139:203–211.
- Ogura M, Yamaguchi H, Yoshida K, et al. DNA microarray analysis of Bacillus subtilis DegU, ComA and PhoP regulons: an approach to comprehensive analysis of B. subtilis two-component regulatory systems. Nucleic Acids Res. 2001;29:3804–3813.
- Comella N, Grossman AD. Conservation of genes and processes controlled by the quorum response in bacteria: characterization of genes controlled by the quorum-sensing transcription factor ComA in Bacillus subtilis. Mol Microbiol. 2005;57:1159–1174.
- Nakano MM, Xia LA, Zuber P. Transcription initiation region of the srfA operon, which is controlled by the comP-comA signal transduction system in Bacillus subtilis. J Bacteriol. 1991;173:5487–5493.
- D’Souza C, Nakano MM, Zuber P. Identification of comS, a gene of the srfA operon that regulates the establishment of genetic competence in Bacillus subtilis. Proc Natl Acad Sci USA. 1994;91:9397–9401.
- Turgay K, Hahn J, Burghoorn J, et al. Competence in Bacillus subtilis is controlled by regulated proteolysis of a transcription factor. Embo J. 1998;17:6730–6738.
- Msadek T, Kunst F, Klier A, et al. DegS-DegU and ComP-ComA modulator-effector pairs control expression of the Bacillus subtilis pleiotropic regulatory gene degQ. J Bacteriol. 1991;173:2366–2377.
- Do TH, Suzuki Y, Abe N, et al. Mutations suppressing the loss of DegQ function in Bacillus subtilis (natto) poly-γ-glutamate synthesis. Appl Environ Microbiol. 2011;77:8249–8258.
- Ohsawa T, Tsukahara K, Ogura M. Bacillus subtilis response regulator DegU is a direct activator of pgsB transcription involved in γ-poly-glutamic acid synthesis. Biosci Biotechnol Biochem. 2009;73:2096–2102.
- Miras M, Dubnau D. A DegU-P and DegQ-dependent regulatory pathway for the K-state in Bacillus subtilis. Front Microbiol. 2016;7:1868.
- Hamoen LW, Van Werkhoven AF, Venema G, et al. The pleiotropic response regulator DegU functions as a priming protein in competence development in Bacillus subtilis. Proc Natl Acad Sci USA. 2000;97:9246–9251.
- Stanley NR, Lazazzera BA. Defining the genetic differences between wild and domestic strains of Bacillus subtilis that affect poly-γ-DL-glutamic acid production and biofilm formation. Mol Microbiol. 2005;57:1143–1158.
- Tran LS, Nagai T, Itoh Y. Divergent structure of the ComQXPA quorum-sensing components: molecular basis of strain-specific communication mechanism in Bacillus subtilis. Mol Microbiol. 2000;37:1159–1171.
- Tortosa P, Logsdon L, Kraigher B, et al. Specificity and genetic polymorphism of the Bacillus competence quorum-sensing system. J Bacteriol. 2001;183:451–460.
- Stefanic P, Mandic-Mulec I. Social interactions and distribution of Bacillus subtilis pherotypes at microscale. J Bacteriol. 2009;191:1756–1764.
- Bacon Schneider K, Palmer TM, Grossman AD. Characterization of comQ and comX, two genes required for production of ComX pheromone in Bacillus subtilis. J Bacteriol. 2002;184:410–419.
- Ansaldi M, Marolt D, Stebe T, et al. Specific activation of the Bacillus quorum-sensing systems by isoprenylated pheromone variants. Mol Microbiol. 2002;44:1561–1573.
- Okada M. Post-translational isoprenylation of tryptophan. Biosci Biotechnol Biochem. 2011;75:1413–1417.
- Tsuji F, Ishihara A, Kurata K, et al. Geranyl modification on the tryptophan residue of ComXRO-E-2 pheromone by a cell-free system. FEBS Lett. 2012;586:174–179.
- Okada M, Yamaguchi H, Sato I, et al. Chemical structure of posttranslational modification with a farnesyl group on tryptophan. Biosci Biotechnol Biochem. 2008;72:914–918.
- Okada M, Yamaguchi H, Sato I, et al. Structure-activity relationship studies on quorum sensing ComXRO-E-2 pheromone. Bioorg Med Chem Lett. 2007;17:1705–1707.
- Wang K, Ohnuma S. Chain-length determination mechanism of isoprenyl diphosphate synthases and implications for molecular evolution. Trends Biochem Sci. 1999;24:445–451.
- Okada M, Ishihara A, Yamasaki R, et al. A region corresponding to second aspartate-rich motif in tryptophan isoprenylating enzyme, ComQ, serves as a substrate-binding site. Biosci Biotechnol Biochem. 2014;78:550–555.
- Miwa Y, Fujita Y. Involvement of two distinct catabolite-responsive elements in catabolite repression of the Bacillus subtilis myo-inositol (iol) operon. J Bacteriol. 2001;183:5877–5884.
- Fabret C, Ehrlich SD, Noirot P. A new mutation delivery system for genome-scale approaches in Bacillus subtilis. Mol Microbiol. 2002;46:25–36.
- Hamoen LW, Haijema B, Bijlsma JJ, et al. The Bacillus subtilis competence transcription factor, ComK, overrides LexA-imposed transcriptional inhibition without physically displacing LexA. J Biol Chem. 2001;276:42901–42907.
- Lovett CM Jr, Love PE, Yasbin RE, et al. SOS-like induction in Bacillus subtilis: induction of the RecA protein analog and a damage-inducible operon by DNA damage in Rec+ and DNA repair-deficient strains. J Bacteriol. 1988;170:1467–1474.
- Roberts MS, Cohan FM. Recombination and migration rates in natural populations of Bacillus subtilis and Bacillus mojavensis. Evolution. 1995;49:1081–1094.
- Nishio K, Nodake Y, Hamada K, et al. Expression, purification, crystallization and preliminary X-ray studies of geranylgeranyl diphosphate synthase from Thermus thermophilus HB8. Acta Crystallogr D Biol Crystallogr. 2004;60:178–180.
- Waterhouse A, Bertoni M, Bienert S, et al. SWISS-MODEL: homology modelling of protein structures and complexes. Nucleic Acids Res. 2018;46:W296–W303.