ABSTRACT
A liquid chromatography-electrospray ionization-tandem mass spectrometry (LC-ESI-MS/MS) method for the separation and quantification of the enantiomers of N-methylaspartate and N-methylglutamate, after derivatization with Nα-(5-fluoro-2,4-dinitrophenyl)-L-leucinamide was established. The time required for the LC-ESI-MS/MS analysis was within 20 min and the detection limit was approximately 10 fmol per injection, demonstrating that this method can be used for the rapid determination of D-aspartate N-methyltransferase activity in the ark shell clam Scapharca broughtonii.
Abbreviations: NMDA: N-methyl-D-aspartate; NMLA: N-methyl-L-aspartate; NMDG: N-methyl-D-glutamate; NMLG: N-methyl-L-glutamate; NMA: N-methylaspartate; NMG: N-methylglutamate; HPLC: high-performance liquid chromatography; SAM: S-adenosyl-L-methionine; OPA: o-phthalaldehyde; LC-ESI-MS/MS: liquid chromatography-electrospray ionization-tandem mass spectrometry; FDLA: Nα-(5-fluoro-2,4-dinitrophenyl)-L-leucinamide; FDAA: Nα-(5-fluoro-2,4-dinitrophenyl)-L-alaninamide; ESI: electrospray ionization; LC-ESI-MS: liquid chromatography-electrospray ionization-mass spectrometry; MS/MS: tandem mass spectrometry
GRAPHICAL ABSTRACT
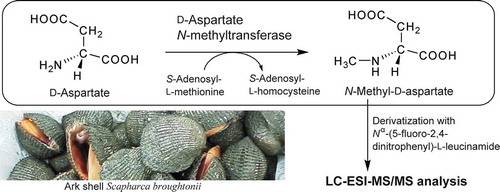
Liquid chromatography-electrospray ionization-tandem mass spectrometric assay for D-aspartate N-methyltransferase activity in ark shells.
N-Methyl-D-aspartate (NMDA), an agonist for NMDA glutamate receptors, is a well-known neuroexitatory compound [Citation1] and has long been used in neurological research. NMDA was first discovered in nature in 1987; when it was isolated from the ark shell clam Scapharca broughtonii [Citation2]. We have previously developed a high-performance liquid chromatography (HPLC)-based method (fluorescence HPLC method) to analyze NMDA [Citation3] and found that, not only NMDA, but also the enantiomer, N-methyl-L-aspartate (NMLA), and the analogous compounds, N-methyl-D-glutamate (NMDG) and N-methyl-L-glutamate (NMLG), were present in the tissues of various aquatic invertebrates [Citation4,Citation5]. Since then, various analytical methods for NMDA have been established [Citation6–Citation11].
D’Aniello et al. have developed an enzymatic HPLC method to investigate the biosynthesis of NMDA [Citation6] and demonstrated that the tissues of Wistar rat [Citation6], the ascidian Ciona intestinalis [Citation12] and the amphioxi Branchiostoma lanceolatum [Citation13] contain enzymes that can synthesize NMDA using D-aspartate as a substrate and S-adenosyl-L-methionine (SAM) as a methyl group donor. The enzyme that catalyzes the synthesis of NMDA is D-aspartate N-methyltransferase (S-adenosyl-L-methionine:D-aspartate N-methyltransferase or N-methyl-D-aspartate synthase) (). We detected D-aspartate N-methyltransferase activity, as well as L-aspartate N-methyltransferase activity that catalyzes the N-methylation of L-aspartate, in the radial nerve cord of the starfish Asterias amurensis, by the fluorescence HPLC method described above [Citation14].
In fluorescence HPLC-based activity assays [Citation14], unreacted SAM and D- aspartate in the samples must be removed through ion-exchange column treatment and o-phthalaldehyde (OPA) treatment, respectively, prior to derivatization of NMDA in the samples with (+)-1-(9-fluorenyl)ethyl chloroformate and HPLC analysis of NMDA, which is produced by the enzymatic reaction. This HPLC analysis takes approximately 60 min. Another enzymatic HPLC-based activity assay requires OPA treatment, thin-layer chromatography, and treatment with several columns, followed by a further treatment with D-aspartate oxidase, prior to the HPLC analysis [Citation6,Citation12,Citation13]. Such complex operations are a major obstacle to the investigation of D-aspartate N-methyltransferase, including in the purification and characterization of the enzyme. Thus, a simple and rapid assay method with high selectivity and sensitivity is desirable for measuring the enzyme activity of samples before denaturation of the enzyme. Therefore, we have developed a method to analyze N-methyl amino acids using liquid chromatography-electrospray ionization-tandem mass spectrometry (LC-ESI-MS/MS). We have also established an assay system based on this method, to determine the level of enzyme activity in the biosynthesis of N-methyl amino acids in biological samples.
The ark shell clam has been identified to have a high content of NMDA [Citation3,Citation4] and D-aspartate [Citation15], as well as a high content of aspartate racemase, which is possibly involved in NMDA production by producing D-aspartate [Citation16,Citation17]. Thus, the ark shell clam is one of the most useful, attractive, and commercially available invertebrates to investigate the metabolism of NMDA and D-aspartate. In the present study, therefore, we determined D-aspartate N-methyltransferase activity in the tissues of the ark shell clam S. broughtonii.
Materials and methods
Animals and chemicals
Live S. broughtonii were obtained from a local fish market in Fukushima, Japan and immediately dissected to harvest the foot muscle and mantles. The harvested tissues were stored at −20°C until further use.
Nα-(5-Fluoro-2,4-dinitrophenyl)-L-leucinamide (FDLA) was purchased from Tokyo Chemical Industry Co., Ltd. (Tokyo, Japan). NMDA, NMLA, N-methyl-DL-glutamate, sodium bicarbonate, LC-MS grade acetonitrile, and 0.1% formic acid were purchased from Sigma–Aldrich (St. Louis, MO, USA). LC-MS grade methanol and special grade reagents were purchased from FUJIFILM Wako Pure Chemical Corp. (Osaka, Japan).
FDLA derivatization of N-methyl amino acids
The FDLA derivatization of N-methyl amino acids was performed in accordance with previous reports [Citation18]. Eighty microliters of 1% FDLA acetone solution and 20 μL of 0.5 mol/L aqueous sodium bicarbonate solution containing the N-methyl amino acid were mixed and incubated at 40°C for 60 min. Five microliters of 2 mol/L HCl was added, and then 1470 μL of methanol was added for dilution, followed by filtration of the diluted solution with a 0.45 μm membrane (Millex-LH, Nihon Millipore, Tokyo, Japan). The filtrate (10 μL) was injected into the LC-ESI-MS/MS system described below.
LC-ESI-MS/MS analysis
The LC-ESI-MS/MS system consisted of an HPLC (LaChrom Elite, Hitachi High-Technologies Corp., Tokyo, Japan) and ion trap mass spectrometer (amaZon SL, Bruker Daltonics GmbH, Bremen, Germany), equipped with an ESI source (Bruker Daltonics GmbH, Bremen, Germany). A Hydrosphere C18 column (3.0 × 150 mm, 3 μm) (YMC Corp., Kyoto, Japan) was used along with a mobile phase of acetonitrile-methanol-0.1% formic acid (37:6:57, v/v/v) at a flow rate of 0.2 mL/min. The column temperature was set at 40°C. The MS/MS conditions were: polarity: positive; capillary voltage: – 4500 V; end plate offset: – 500 V; nebulizer gas: 1.2 bar; drying gas: 11 L/min; and drying temperature: 250°C. The software DataAnalysis 4.1 and QuantAnalysis 2.1 (Bruker Daltonics) were used for data processing of spectra and chromatograms.
Preparation of crude enzyme solution from S. broughtonii tissue
Using a tissue homogenizer (T18 Basic, IKA-Werke GmbH and co., Staufen, Germany), a homogenate was prepared on ice by combining approximately 0.2 g of S. broughtonii tissue and 500 µL of 30 mM Tris-HCl buffer (pH 8.0). The homogenate was centrifuged at 12,000 × g for 20 min at 4°C, and the resulting supernatant was transferred to a microdialysis unit Easy-Sep (TOMY SEIKO CO., Tokyo, Japan) and was subjected to 1-h dialysis against 100 times its volume of 300 mmol/L Tris-HCl buffer (pH 8.0) at 4°C (three times) to obtain a crude enzyme solution.
Measurement of D-aspartate N-methyltransferase activity
A reaction mixture consisting of 20 µL of the crude enzyme solution, 5 µL of 30 mmol/L Tris-HCl buffer (pH 8.0) containing 200 mmol/L D-aspartate, and 5 µL of 30 mmol/L Tris-HCl buffer (pH 8.0) containing 120 mmol/L SAM was incubated at 30°C. After the reaction was quenched by adding 1 mL of cold methanol, the mixture was centrifuged at 12,000 × g for 20 min at 4°C. The supernatant was concentrated and dried under vacuum at 40°C. The residue was dissolved in 100 µL of 0.5 mol/L aqueous sodium bicarbonate solution. The resulting solution was derivatized with FDLA and subjected to LC-ESI-MS/MS as described above.
Results and discussion
Nα-(5-Fluoro-2,4-dinitrophenyl)-L-leucinamide (FDLA) was used for the derivatization of N-methyl amino acids prior to LC-ESI-MS/MS analysis. This reagent is used in the modified Marfey’s method developed by Fujii et al. [Citation18]. FDLA has a better ionization efficiency than Marfey’s reagent [Nα-(5-fluoro-2,4-dinitrophenyl)-L-alaninamide (FDAA); Tokyo Chemical Industry Co., Ltd. (Tokyo, Japan)] in electrospray ionization (ESI) processes, and has been used to determine the absolute configuration of constituent amino acids in a peptide using LC-ESI-MS [Citation18].
After derivatization using FDLA, N-methylaspartate (NMA) and N-methylglutamate (NMG) standards were analyzed using ESI-MS. The protonated molecules [M + H]+ of NMA and NMG were easily detected at m/z 442 and 456, respectively (). Early attempts to detect protonated molecules after derivatization with Marfey’s reagent (FDAA) did not give adequate results. This result is consistent with a previous report that the ionization rate of an FDLA derivative was approximately 30 times higher than that of the FDAA derivative [Citation18]. Therefore, we opted to use FDLA as the derivatization reagent in the present study.
Figure 2. ESI-MS and MS/MS spectra of FDLA derivatives of authentic N-methyl amino acids.
(a) ESI-MS spectrum of FDLA derivatives of authentic NMA. (b) ESI-MS spectrum of FDLA derivatives of authentic NMG. (c) MS/MS spectrum for the precursor ion at m/z 442 shown in a. (d) MS/MS spectrum for the precursor ion at m/z 456 shown in b.
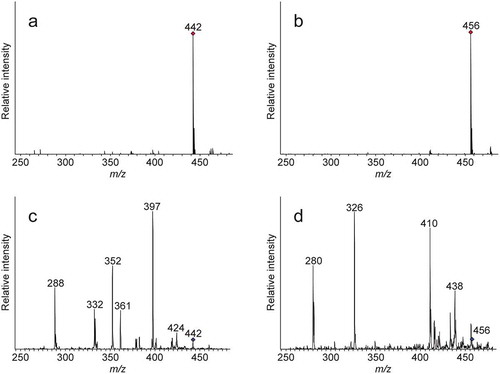
Subsequently, the protonated molecules of NMA and NMG at m/z 442 and 456, respectively, were selected as the precursor ions for further analysis of fragment ions using MS/MS (). Representative fragment ions were detected at m/z 424, 397, 361, 352, 332, and 288 for NMA and at m/z 438, 410, 326, and 280 for NMG, which are characteristic of the respective N-methyl amino acids. Under the same conditions, aspartate produced three major representative fragment ions at m/z 378, 318, and 274 from the protonated molecule at m/z 428; whereas glutamate produced three major representative fragment ions at m/z 396, 312, and 266 from the protonated molecule at m/z 442. Thus, the MS/MS spectra of NMA and NMG were clearly different from those of aspartate and glutamate. Although we used an ion-trap mass spectrometer, a similar result could be obtained using a tandem quadrupole mass spectrometer or quadrupole time-of-flight mass spectrometer (data not shown). These results indicate that the method can be used universally independent of the analytical instrument.
We obtained MS/MS chromatograms using the fragment ion at m/z 288 generated from m/z 442 for NMA and the fragment ion at m/z 280 generated from m/z 456 for NMG as the respective monitoring ions (). Under the conditions described above, NMG and NMA enantiomers were successfully separated in under 20 min. We derivatized and analyzed authentic NMG and NMA enantiomers at various concentrations and plotted calibration curves for the substances. The validation parameters for all four enantiomers are summarized in .
Table 1. Validation parameters for NMDA, NMLA, NMDG, and NMLG.
Figure 3. LC-ESI-MS/MS chromatograms of the FDLA derivatives of authentic N-methyl amino acids and from the assay solutions for D-aspartate N-methyltransferase in S. broughtonii.
(a) LC-ESI-MS/MS chromatograms of the FDLA derivatives of authentic NMDA and NMLA (solid line) with m/z 288 as the monitoring ion, and authentic NMDG and NMLG (dashed line) with m/z 280 as the monitoring ion. A mixture of 5 µmol/L each of NMDA, NMLA, NMDG, and NMLG was derivatized with FDLA.(b) LC-ESI-MS/MS chromatograms of FDLA derivatives in the assay solution prepared from the dialyzed tissue homogenate from the mantle of S.broughtonii, D-aspartate, and SAM without incubation at 30°C (blank). The monitoring ions used the fragment ion of m/z 288 produced from the precursor ion of m/z 442.(c) LC-ESI-MS/MS chromatograms of FDLA derivatives from the assay solution, prepared from the same dialyzed tissue homogenate used in (b), D-aspartate, and SAM with incubation for 10 min at 30°C. The monitoring ions used the fragment ion of m/z 288 produced from the precursor ion of m/z 442.
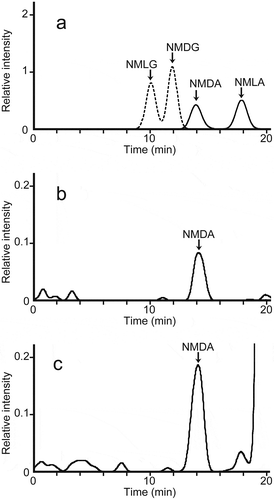
Previously, we have detected D-aspartate N-methyltransferase activity in the starfish radial nerve cord, where NMDA is found at a high concentration [Citation14]. As NMDA was first discovered to naturally occur in S. broughtonii [Citation2], which has a NMDA concentration comparable to that in the starfish radial nerve cord [Citation3,Citation4,Citation14], we used the mantle tissue of S. broughtonii to assay D-aspartate N-methyltransferase activity.
Typical chromatograms of the FDLA-treated assay solutions for D-aspartate N-methyltransferase in S. broughtonii, with or without, incubation for 10 min at 30°C are shown in , respectively. The NMDA detected in the assay solution was identified from the retention time in the MS/MS chromatogram and the fragment ions in the MS/MS spectrum, which were consistent with those of authentic NMDA. The peak area in the MS/MS spectra of NMDA detected in the assay solution was increased during incubation for a further 20 min. The amount of NMDA produced by the crude enzyme from the mantle of S. broughtonii was expressed as a function of the incubation time. The production rate was linearly proportional to the incubation time over the first 20 min in the assay mixtures contained tissue homogenates corresponding to 15 mg of S. broughtonii mantle. After this initial phase, NMDA production slowed down (data not shown), indicating that the routine assay should be performed within 20 min using crude enzyme from S. broughtonii mantle. Under the developed conditions, D-aspartate N-methyltransferase activity in the mantle of S. broughtonii was 0.582 ± 0.102 nmol/min per g of wet tissue (). With homogenates separately prepared from another portion of the mantles from the same three clams used above, a value of 0.517 ± 0.136 nmol/min per g of wet tissue was obtained using the conventional fluorescence HPLC method. These two values obtained from different assay methods were approximately the same, suggesting that the present method using LC-ESI-MS/MS can be used to measure D-aspartate N-methyltransferase activity in biological tissues. In addition, the D-aspartate N-methyltransferase activity in the foot was 0.025 ± 0.038 nmol/min per g wet tissue ().
Table 2. D-aspartate N-methyltransferase activity in the tissues of S. broughtonii.
The detection limit of NMDA in the present LC-ESI-MS/MS method was approximately 10 fmol, and the detection limit in the LC-ESI-MS/MS method to analyze D-aspartate N-methyltransferase activity was estimated to be approximately 0.5 fmol/min. Comparing the conventional enzymatic HPLC method (e.g. 17.3 nmol NMDA produced per assay mixture after 60 min using 200 mg of rat brains) [Citation6] and the fluorescence HPLC method (detection limit 10 fmol/min) [Citation14], it is obvious that the developed enzyme assay method has sufficiently high sensitivity. This rapid method for analyzing D-aspartate N-methyltransferase activity will be useful to assess enzymatic activity in organisms, as well as in the purification and characterization of this enzyme in biological tissues.
Although high levels of NMDA are known to be present in S. broughtonii tissues [Citation2,Citation3], the origin of NMDA in these tissues has not been elucidated to date. In this study, D-aspartate N-methyltransferase activity was shown for the first time in S. broughtonii tissue. This activity was approximately 20 times higher in the mantle than in the foot (). The levels of NMDA have also been shown to be several times higher in the mantle than in the foot [Citation3,Citation7,Citation10]. The NMDA levels appear to be dependent on D-aspartate N-methyltransferase activity, as D-aspartate oxidase activity, which may degrade NMDA, has not been detected in S. broughtonii tissues [Citation15].
D-Aspartate, which is a substrate of D-aspartate N-methyltransferase, is produced by aspartate racemase in both the mantle and foot tissues of S. broughtonii [Citation15–Citation17], and is present at levels that are 10 times or more higher than for NMDA [Citation4,Citation15]. In the foot, D-aspartate acts as a storage material for anaerobic energy metabolism [Citation19,Citation20], so it may not be necessary to convert D-aspartate to NMDA through D-aspartate N-methyltransferase activity. Conversely, in the mantle, which has higher D-aspartate N-methyltransferase activity, D-aspartate appears to act as a precursor of NMDA, and the produced NMDA may have a physiological function. The mantle has eyes at its edge [Citation21], secretes the organic matrix for shell formation from its epithelium [Citation22,Citation23], and regulates the ionic composition of body fluid [Citation22,Citation23]. Further studies are needed to elucidate the physiological role of NMDA in the mantle.
Recently, Vogeler et al. have reported that the NMDA receptor pathway is also present in bivalves and is involved in larval metamorphosis [Citation24,Citation25]. Because D-aspartate N-methyltransferase activity was found in bivalve mollusks in the present study, it is possible that NMDA may act as an endogenous agonist of the bivalve NMDA receptor.
In conclusion, we have developed a simple and rapid method to analyze N-methyl amino acids using LC-ESI-MS/MS, and have developed an assay for D-aspartate N-methyltransferase activity based on this method. This assay allowed the rapid determination of D-aspartate N-methyltransferase activity in a biological sample. This method will contribute to further advances in enzymological studies of D-aspartate N-methyltransferase, as well as studies on the physiological functions and metabolism of NMDA and related compounds.
Author contributions
K.S. conceived and designed the study with K.A., S.T. and Y.K. S.H. carried out most of the experiments. R.O. performed optimization of LC-ESI-MS/MS conditions. K.S. and S.H. interpreted the data. K.S. wrote the manuscript in consultation with K.A., S.T. and Y.K. All authors discussed the results and commented on the manuscript.
Acknowledgments
We thank Ms. Katsue Miura, Ms. Hiromi Ono, Mr. Syun Hisano and Mr. Takaaki Yamakawa of National Institute of Technology, Fukushima College for supporting the analysis with LC-ESI-MS/MS. We also thank Victoria Muir, PhD, from Edanz Group (www.edanzediting.com/ac) for editing a draft of this manuscript.
Disclosure statement
No potential conflict of interest was reported by the authors.
Additional information
Funding
References
- Watkins JC. The synthesis of some acidic amino acids possessing neuropharmacological activity. J Med Chem. 1962;5:1187–1199.
- Sato M, Inoue F, Kanno N, et al. The occurrence of N-methyl-D-aspartic acid in muscle extracts of the blood shell, Scapharca broughtonii. Biochem J. 1987;241:309–311.
- Todoroki N, Shibata K, Yamada T, et al. Determination of N-methyl-D-aspartate in tissues of bivalves by high-performance liquid chromatography. J Chromatogr B Biomed Sci Appl. 1999;728:41–47.
- Shibata K, Tarui A, Todoroki N, et al. Occurrence of N-methyl-L-aspartate in bivalves and its distribution compared with that of N-methyl-D-aspartate and D,L-aspartate. Comp Biochem Physiol B Biochem Mol Biol. 2001;130:493–500.
- Tarui A, Shibata K, Takahashi S, et al. N-Methyl-D-glutamate and N-methyl-L-glutamate in Scapharca broughtonii (Mollusca) and other invertebrates. Comp Biochem Physiol B Biochem Mol Biol. 2003;134:79–87.
- D’Aniello A, Di Fiore MM, Fisher GH, et al. Occurrence of D-aspartic acid and N-methyl-D-aspartic acid in rat neuroendocrine tissues and their role in the modulation of luteinizing hormone and growth hormone release. Faseb J. 2000;14:699–714.
- Sekine M, Fukuda H, Nimura N, et al. Automated column-switching high-performance liquid chromatography system for quantifying N-methyl-D- and -L-aspartate. Anal Biochem. 2002;310:114–121.
- Tsesarskaia M, Galindo E, Szókán G, et al. HPLC determination of acidic D-amino acids and their N-methyl derivatives in biological tissues. Biomed Chromatogr. 2009;23:581–587.
- Nguyen D-T, Kim K-R, Lee G, et al. Chiral separation of N-methyl-DL-aspartic acid in rat brain tissue as N-ethoxycarbonylated (S)-(+)-2-octyl ester derivatives by GC-MS. Biomed Chromatogr. 2012;26:1353–1356.
- Koga R, Miyoshi Y, Negishi E, et al. Enantioselective two-dimensional high-performance liquid chromatographic determination of N-methyl-D-aspartic acid and its analogues in mammals and bivalves. J Chromatogr A. 2012;1269:255–261.
- Fontanarosa C, Pane F, Sepe N, et al. Quantitative determination of free D-Asp, L-Asp and N-methyl-D-aspartate in mouse brain tissues by chiral separation and multiple reaction monitoring tandem mass spectrometry. PLoS One. 2017;12:e0179748.
- D’Aniello A, Spinelli P, De Simone A, et al. Occurrence and neuroendocrine role of D-aspartic acid and N-methyl-D-aspartic acid in Ciona intestinalis. FEBS Lett. 2003;552:193–198.
- D’Aniello S, Fisher GH, Topo E, et al. N-Methyl-D-aspartic Acid (NMDA) in the nervous system of the amphioxus Branchiostoma lanceolatum. BMC Neurosci. 2007;8:109.
- Shibata K, Sugaya N, Ono W, et al. Determination of D-aspartate N-methyltransferase activity in the starfish by direct analysis of N-methyl-D-aspartate with high-performance liquid chromatography. J Chromatogr B. 2011;879:3229–3234.
- Watanabe T, Shibata K, Kera Y, et al. Occurrence of free D-aspartate and aspartate racemase in the blood shell Scapharca broughtonii. Amino Acids. 1998;14(4):353–360.
- Shibata K, Watanabe T, Yoshikawa H, et al. Purification and characterization of aspartate racemase from the bivalve mollusk Scapharca broughtonii. Comp Biochem Physiol B Biochem Mol Biol. 2003;134(2):307–314.
- Abe K, Takahashi S, Muroki Y, et al. Cloning and expression of the pyridoxal 5ʹ-phosphate-dependent aspartate racemase gene from the bivalve mollusk Scapharca broughtonii and characterization of the recombinant enzyme. J Biochem. 2006;139(2):235–244.
- Fujii K, Ikai Y, Oka H, et al. A nonempirical method using LC/MS for determination of the absolute configuration of constituent amino acids in a peptide: Combination of Marfey’s method with mass spectrometry and its practical application. Anal Chem. 1997;69:5146–5151.
- Shibata K, Watanabe T, Yoshikawa H, et al. Nucleotides modulate the activity of aspartate racemase of Scapharca broughtonii. Comp Biochem Physiol B Biochem Mol Biol. 2003;134(4):713–719.
- Watanabe T, Shibata K, Kera Y, et al. Effects of hypoxic and osmotic stress on the free D-aspartate level in the muscle of blood shell Scapharca broughtonii. Amino Acids. 2005;28(3):291–296.
- Nilsson DE. Eyes as optical alarm systems in fan worms and ark clams. Philos Trans R Soc Lond B Biol Sci. 1994;346:195–212.
- Simkiss K, Wilbur KM. Molluscs-epithelial control of matrix and minerals. In: Simkiss K, Wilbur KM, editors. Biomineralization: cell biology and mineral deposition. San Diego (CA): Academic Press; 1989. p. 230–260.
- Kocot KM, Aguilera F, McDougall C, et al. Sea shell diversity and rapidly evolving secretomes: insights into the evolution of biomineralization. Frontiers in Zoology. 2016;13:23.
- Vogeler S, Miller-Ezzy P, Li X, et al. First report of a putative involvement of the NMDA pathway in Pacific oyster (Crassostrea gigas) development: effect of NMDA receptor ligands on oyster metamorphosis with implications for bivalve hatchery management. Aquaculture. 2018;497:140–146.
- Vogeler S, Wikfors GH, Li X, et al. Larval metamorphosis in four bivalve species in response to NMDA receptor ligands: the NMDA receptor pathway as potential regulator of bivalve transition to spat. Aquaculture. 2019;511:634173.